1 Introduction
The intracontinental plateaus located far away from plate boundary display huge canyon whose origin is debated: the Colorado plateau with the Grand Canyon and the South-African plateau with the Fish River canyon. The Fish River canyon, the second largest canyon, carved the South-African plateau of 550 m along approximately 65 km. The genesis of such continental canyons requires a fall of base level relative to the top of the plateau and appropriate climatic conditions. In continental domain, the base level is either the sea level (global reference), or a lake or a river (local reference). This change in relative base level can be either attributed to intracontinental deformation or variations in local or global eustatism (change in climate conditions). The first hypothesis reveals that intra-continental areas located far from plate boundaries may deform. Therefore, tracking the genesis of intracontinental canyons may reveal the subtle deformation occurring inside a continent that is not so stable, as commonly assumed. A general consensus agrees that the Colorado River is independent of topographic or structural control and that the canyon is mainly controlled by climatic variations (Anders et al., 2005, Hill and Ranney, 2008). But nothing was proposed about the Fish River canyon: when was it generated and to accommodate which process climate or tectonics?
The Namibia recorded Earth history since Archean and recently the opening of the South Atlantic Ocean. A coastal plain and the South-African plateau mainly shape its landscape. This latter feature affects nearly one third of the African continent and is shaped by several erosion surfaces whose elevations range from 1000 to 1500 m (Fig. 1) including the famous “African surface” (King, 1962), and separated by scarps. The evolution of these surfaces is largely debated and results from the coupling between climates processes, erosion efficiency and deformation (Burke, 1996; Partridge and Maud, 1987). The plateau underwent a more or less continuous denudation that may reach 5 km in some places (Brown et al., 2000; Gallagher and Browns, 1999). Several models were proposed to explain such a high plateau: a genesis prior to Gondwana breakup (Dauteuil et al., in correction; Doucoure and de Witt, 2003; Gilchrist and Summerfield, 1994; Pysklwec and Mitrovica, 1999; Van Der Beek et al., 2002), an uplift during the Upper Cretaceous (de Wit, 2004; Nyblade and Sleep, 2003; Partridge and Maud, 1987; Smith, 1982) or during the Cenozoic (Burke, 1996). In this debate, the genesis of the Fish River canyon should constrain the evolution of this plateau. After a long humid and hot phase during the Late Cretaceous and the Early Cenozoic, the climate changed to the arid conditions during the mid-Miocene (Burke and Gunnell, 2008; Partridge and Maud, 1987).

Structural map of Fish River canyon with faults and slope trend plotted over a shaded DEM. In insert, topographic map of South-West Africa with location of the Fish River canyon.
Carte structurale du canyon de la Fish River reportée sur un relief. En encart, carte topographique du Sud-Ouest de l’Afrique montrant la localisation du canyon et le bassin versant de la rivière.
The Fish River flows over 650 km inside the South African plateau from north to south where it joins the Orange River (Fig. 1). At latitude 27°28′S, the Fish River canyon incises the plateau up to 550 m along 65 km. The incision reveals sub-horizontal strata of lower Nama group of Late Neoproterozoic, over tilted metamorphic rocks (granites and gneiss) forming the Namaqualand group of Palaeoproterozoic (Fig. 2). A main unconformity separates these two formations. From the canyon mouth to its confluence with the Orange River, the Fish River runs through widely eroded area of Namaqualand formations. About twenty kilometers westward, the Konkiep River flows over the plateau more or parallel to the Fish River (Fig. 1). It less incises the plateau and joins the Fish River close to its junction with the Orange River.

Photography of Fish River canyon showing the unconformity separating the subhorizontal Nama group (Late Neoproterozoic age) and the Namaqualand metamorphic complex (Paleoproterozoic age) dipping 50° toward the northwest.
Panorama de la Fish River montrant la rivière incisant les formations du groupe de Nama (Neoprotérozoïqe tardif) et le complexe métamorphique du Namaqualand (Paléoprotérozoïque) plongeant à 50° vers le nord-ouest. Les deux formations sont séparées par une discontinuité majeure.
2 The upper canyon
The upper canyon located in the northern part of the system and trends at N040° inside a wide depression with a flat bottom, long of 22.8 km and wide of 10 km. The square-shaped valley incised up to 400 m the surrounding upper surfaces of the South African plateau. The river displays large meanders running over the entire graben bottom. They slightly incised the bottom and generated two intermediate surfaces (Fig. 3) that gently dip toward southwest. This sinuosity (ratio between stream length and river length) (Schumm and Holbrot, 2000) reaches a value of 2.18 with a southward increase of meander size. This value is twice higher than on the plateau, at north. The high sinuosity and the change in wavelength toward the southwestern border of the canyon attest to a change in the river flowing induced by a lock at the downstream. The mapping of the unconformity between Namaqualand complex and Nama group indicates NE-SW and NNW–SSE normal faults that offset this unconformity (Fig. 2). The Namaqualand complex never crops out inside the valley, while it appears on the canyon flanks.
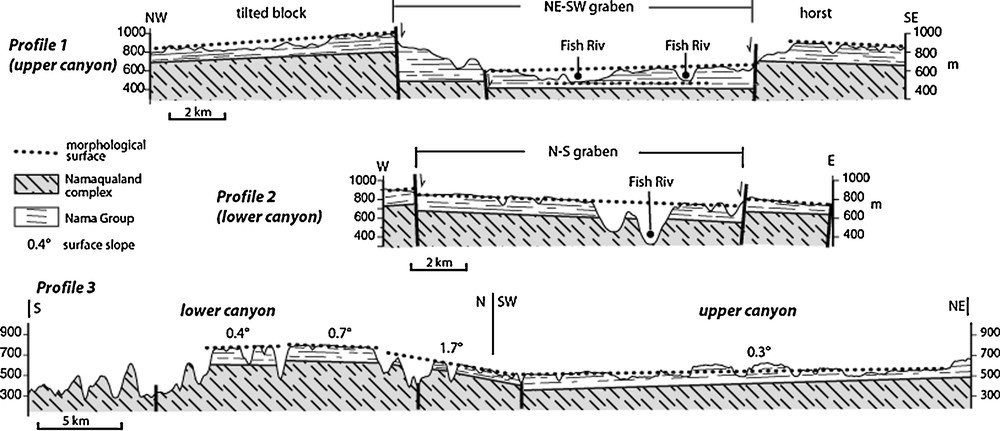
Geological sections across (1, 2) and along the Fish River canyon (3). Their locations are drawn on Fig. 1. The sections 1 and 2 point out the graben pattern while the section 3 reveals that the river flows in opposite way compared to the slope of the graben floor.
Coupes géologiques perpendiculaires (1,2) et parallèle (3) aux canyons. Leur position est indiquée sur la Fig. 1. Ces coupes illustrent les structures des deux canyons et montrent comment la rivière coule à contre-pente.
The northern flank displays a rough surface dipping northeastward carved up to 100 m by the Fish River (Fig. 1). Inside the flank, the river sinuosity increases (2.43) typical of the erosion generated during vertical displacements. It is interpreted as a tilted block incised by the river at the same rate. A drainage network slightly incising the plateau shapes the surface of the southern flank. It is connected to the Fish River by only one connector. This area network increases toward northeast where the elevation is lower. Thus the network is more mature at northeast, that is interpreted as a larger relative uplift at the junction with the lower canyon. A normal fault limits this flank at southeast. By consequence, the upper canyon was installed inside a graben bordered by a tilted block and a horst. The relative motion between these blocks modified the drainage network: a meandering inside the graben and on the northwestern flank and the asymmetric network on the southeastern horst.
3 The lower canyon
The southern part of the Fish River canyon trends north-south. Meanders deeply incise a wide depression high from 700 to 900 m while the river flows at an elevation of 420 m at north and of 285 m at south (Fig. 3). The incision largely affects the Namaqualand metamorphic complex inside the canyon and the main unconformity is easily seen on the canyon cliffs. The meanders have short tributaries mainly developed on the western side of the river. The sinuosity is 2.57, the highest value of the study area. The meander size, the incision amount and the number of tributaries increase toward the canyon mouth. All theses observations reveal that this zone was uplifted while the river dug.
The outcrops show north-south normal faults that offset the main unconformity and the topography. The western flank is higher of 200 m than the eastern one: this difference was generated by faults with greater throw at east than at west (Fig. 3). Therefore, the structure corresponds to an asymmetric graben trending N010°. This graben belongs to a set of north-south normal faults located on both side of the canyon. The normal faults offset slightly the topographic surface (Fig. 3). The graben floor has a variable dip: at south it dips 0.4° southward, at middle part 0.7° northward and at north 1.7° northward. Continental erosion flat surfaces outside river-controlled domains have slopes less than 1°; higher angles result from a tilting. Thus, the graben floor displays the differential vertical displacement of 250 m between south and north, at the junction with the upper canyon. At this place, the floor dips northward while the river flows southward proving that the river existed before the graben genesis and before the tilting. Two deformation stages affected this area: a first small east-west extension followed by a large uplift.
At the canyon mouth, the sudden change in morphology suggests the location of a main fault zone trending NE–SW. However, it was intensively eroded and this prevents it from being visible in the landscape: it remains a discontinuous scarp at the northeast of the canyon exit. This NE–SW faulted zone is parallel to the trend of the upper canyon. Downstream, the canyon ends in a wide plain at an elevation of 600–500 m where the Fish River runs with small meander over 60 km until the Orange River.
4 Genesis of the canyon
The Fish River canyon is shaped by two grabens attesting to a strong feedback between a deformation and the erosion. The relative timing between the grabens indicates the north-south graben was generated before the NE–SW one. The next question concerns the timing between the deformations and the evolution of the Fish River (Fig. 4). Firstly, the river existed longer before the deformations because it incised all the structures generated by the deformation. Furthermore, the relative motions of the blocks modify the stream shape by increasing locally the meandering. By consequence, the river had probably a more linear stream mainly oriented north-south before the deformations. The stream would probably be as the current stream at north when it flows over the South-African plateau. The genesis of the small north-south grabens slightly affected the river stream because its vertical component was small and rate very slow. Reversely, the NE–SW horsts and grabens largely disturbed the landscape because the vertical component was higher: it generated the main incision and an important meandering that affected differently the stream: large meanders in the upper canyon and small meanders in the lower canyon.

Evolution of the Fish and Konkiep Rivers since the Late Paleocene. The evolution is controlled by two stretching phases during Late (?) Eocene and Plio-Pleistocene that modified the meandering and the entrenchment of the rivers. The Pleistocene phase had the greater impact because it generated large vertical motions that generated the main incision.
Évolution des rivières Konkiep et Fish depuis le Paléocène terminal. Cette évolution est contrôlée par deux épisodes de déformation : l’un probablement Éocène tardif et l’autre Plio-Pléistocène. Cette dernière phase est à l’origine de l’incision majeure du plateau.
The main incision occurred during the second deformation stage that should be probably contemporaneous either to a humid period or during a dry period with extreme climatic events. Reversely, it is impossible to determine the climatic context during the first stage because the topographic pattern is not favorable to erosion. Thus, the parameter controlling the canyon genesis mainly is the tectonics compared to the climate context.
If these deformations increased the meandering and the entrenchment of the Fish River, they did not significantly shift the main stream of the river. However, the Konkiep River located west of the Fish River has a current stream that was probably shifted in the past (Fig. 4). Today, this river has a north-south stream that suddenly trend toward southwest at latitude 27°37′S (Fig. 1). This bend is associated to a sinuosity increase. This change is located where the river flows on the tilted block located on the northern side of the NE–SW graben. The track of the ancient stream is preserved in the topography at west of the lower canyon where a north-south elevated valley digs the African surface. The change of river stream modified the confluence with the Fish River that was probably at least 30 km further north before the deformation event.
These two rivers behaviors well illustrate the challenge between tectonic and climatic processes: the Fish River increased its sinuosity and its entrenchment while the Konkiep River increased locally the sinuosity and shifted the stream. These differences could not be attributed to the climate variability because the two rivers are too close: they are interpreted as resulting from the efficiency to erode the substratum, which is higher for the Fish River than for the Konkiep River. The age of these deformations is difficult to estimate because of the lack of geological markers. To constrain it, we will review the deformations that affected the South-African plateau.
5 Deformations of the South-African plateau
The two grabens are limited by parallel normal faults trending as the main graben direction. The method of Tron and Brun (1991) and of Dauteuil and Brun (1996) indicate that the lower canyon is associated to an east-west stretching generating small grabens, widespread over a wide zone, and that the upper canyon results from NW–SE extension forming a deep and narrow graben. Relative chronology between faults indicates that the east-west extension occurred before the NW–SE extension. Furthermore, the large uplift associated to the NW–SE phase affected the extensional north-south structures.
Several studies indicate that discrete faulting affect the South-African plateau (Fig. 5). The well documented is the Kalahari rift that trends NE–SW and joints the Okavango delta to the East-African Rift throughout the Kariba rift and the Zambezi transform zone. This rift zone is interpreted as a southwestward propagation of East-African Rift (Modisi et al., 2000). Scarce works (Huntsman-Mapila et al., 2005; Ringrose et al., 2002; Thomas and Shaw, 1991) suggest a similar fault south of the Okavango delta. By consequence, the NE–SW graben of the Fish River is a new evidence of the westward location of this deformation. The Kalahari sands masks the connection between the Kalahari and Fish River rifts. However, the isopach map (Haddon and McCarthy, 2005) shows several deposit centers trending NE–SW and a NE–SW basement high, at south of the Kalahari rift. Therefore, this NE–SW deformation is older to contemporaneous to the first deposits whose age is estimated less than 5 Ma for the lower part (Thomas and Shaw, 1991). The maximum of accumulation occurred during the late Pleistocene (Stokes et al., 1997) or 120,000 BP (Kinabo et al., 2007). Thus the rift system is older than 120,000 yr and probably active during the Pliocene during an arid period (Weigelt et al., 2008).
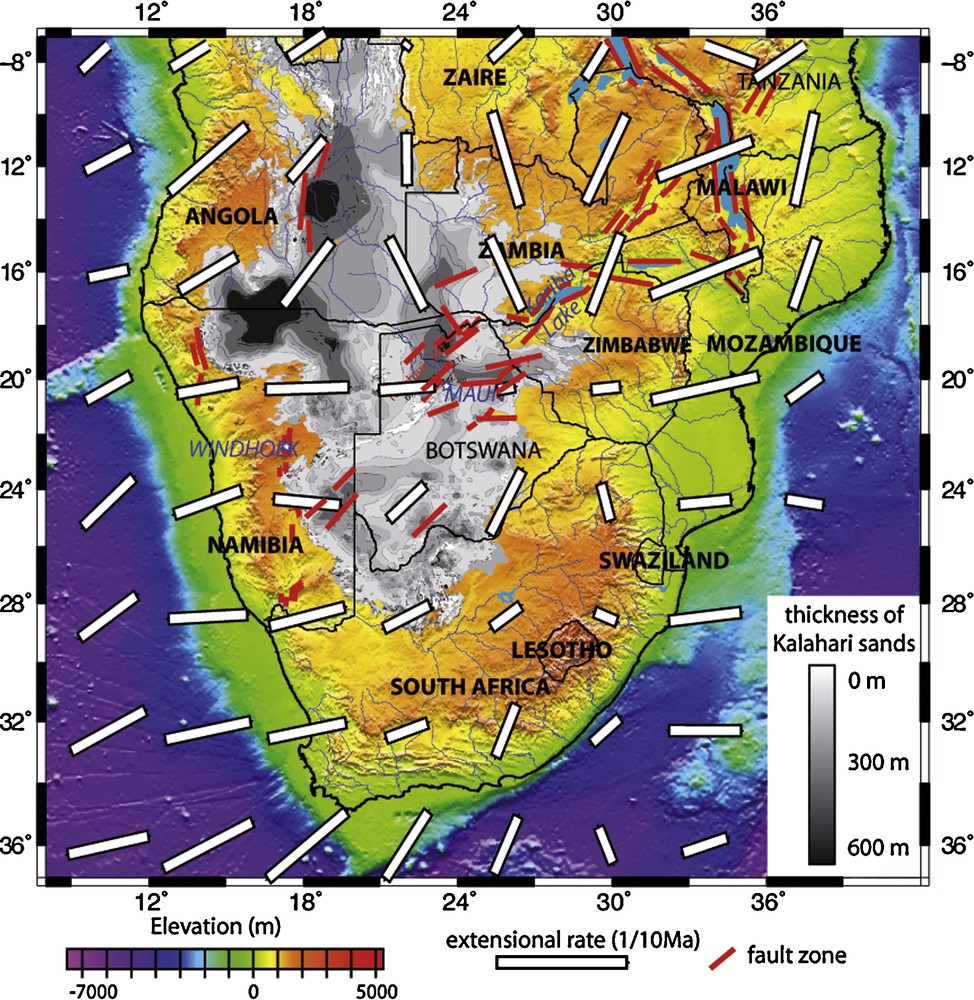
Deformation pattern of South-African plateau during the Cenozoic drawn on the topography. The Cenozoic deformation is subtle and corresponds to two sets of normal faults: NE–SW and north-south. The north-south set is restricted to the western side of the while the NE–SW set widely deformed the plateau. This later deformation seems have controlled partly the Kalahari deposits. The green lines represent the extensional direction at the base of the lithosphere (Forte et al., 2010).
Champ de déformations du plateau Sud-Africain pendant le Cénozoïque, reporté sur la topographie. Ce champ de déformations correspond à deux phases : une première extension ténue, est-ouest, limitée à l’ouest du plateau sud-africain et la seconde une extension NW–SE omniprésente qui contrôle les dépôts des sables du Kalahari. Les lignes vertes correspondent aux directions d’étirement en base de lithosphère, issues des travaux de Forte et al. (2010).
The second N–S structural trend is poorly described throughout the South-African plateau, except in the East-African rift. However, north-south rifts disappear at latitude of 20°S on the eastern margin of Africa and no structures affected the inner plateau. The only evidence is the graben of Windhoek that is associated to phonolite intrusions of Paleocene (Burger and Walraven, 1976; Marsh, 2010). The geological maps and satellite images reveal also meridian faults that affect Karoo basalts at South of Windhoek and close to the Skeleton coast, at north (Fig. 5). These later structures were active probably during Cenozoic. This east-west stretching seems localized on the western side of the South-African plateau. These features show a small subsidence and indicate a low stretching amount, as observed for the lower canyon of the Fish River canyon. In addition, the isopach map of the Kalahari sands does not display large north-south depot centers.
By consequence, the South-African plateau is affected by two extensional phases during the Cenozoic: one during the Earlier Oligocene that corresponds to a tiny east-west extension restricted to its western side; the second one stretched the entire plateau along a NW–SE direction during the Late Pleistocene, maybe slightly before. This latter phase generated important displacements that controlled the deposits of Kalahari sands. In the central part, the deformation started early, during the Eocene by an east-west extension mainly focused on the western side of the plateau.
The origin of two other extensional phases is unknown. Classically, the NE–SW structures are interpreted, as a consequence a southwestward propagation of the East-African rift (Modisi et al., 2000). The world stress map highlights also a difference in the stress direction between the East-African rift and the inner South-African plateau (Heidbach et al., 2009). Forte et al. (2010) inverted the present mantle dynamics until the Oligocene thanks the seismic tomography. Their simulations display NW–SE shearing at the base of the lithosphere below the inner plateau and east-west shearing at the western side of the plateau. This simulated shearing field displays a direction variability that fits well with the geological observations. Therefore, these deformations are generated by deep-mantle dynamics that impacted the Cenozoic evolution of the landscape.
The last question is the effect of climate on the canyon evolution. The main incision and the change in meandering occurred during the second deformation with an inferred age of Plio-Pleistocene. This period corresponds to the beginning of the arid conditions. Even if the first phase is not well dated (Late Mesozoic–Early Cenozoic) is contemporaneous to a long humid period. No strict correlations seem exist between climatic events and canyon evolution. Thus, the Fish River canyon is mainly controlled by the tectonic and rather than by the climatic conditions. This genetic model is completely different from the model currently proposed for the Grand Canyon (Anders et al., 2005).
6 Conclusions
This study about the Fish River canyon reveals that the deformation is the main process controlling the canyon genesis relative to the climatic conditions. The deformation formed two distinct grabens: the lower canyon during an Eocene east-west extension, the upper canyon during a Plio-Pleistocene NW–SE extension. This latter phase generated large vertical displacements that induced a large carving of the plateau and changing the stream pattern of the Fish River. These deformations are consistent with structures affecting the South-African plateau. If the east-west extension is poorly documented over of the plateau, the NW–SE extension was previously documented on the middle and eastern part. Therefore, this NW–SE extension affected a large part of the plateau shaped into NE–SW structures that controlled partly the Kalahari sand deposits. The characteristics of this deformation are consistent with a mantle dynamics that acted on the lithosphere of the South-African plateau and impacted the landscape evolution during the Late Cenozoic.
Acknowledgments
The CNRS-INSU program “Action Marges” and ANR TopoAfrica support this work. We appreciate constructive discussions with A. Forte and R. Moucha about the mantle dynamics.