1 Introduction
In many countries, landslide is considered as one of the most severe natural disasters. A landslide causes huge socioeconomic losses especially in mountainous areas. Statistics from the Center for Research on Epidemiology of Disasters (CRED) indicate that landslides are responsible for at least 17% from all fatalities of natural hazards worldwide. The landslides are found to have caused approximately 5000 bereavements and property deprivations, which represent over US$ 3000 billion during the last century (Laccase and Nadim, 2009). Malaysia is one of the countries where landslide has become a major fatality source. It is a fact that in Malaysia, landslides have been identified as the second most damaging natural disaster after floods (Matori et al., 2011). From 1973 to 2007, the total economic loss due to landslides was estimated at about US$ 5 billion (JKR, 2009). The first written record of major landslides in Malaysia occurred on 7 December 1919 at Bukit Tunggal, Perak. Some major recorded landslide events in Malaysia since 1990 that turned out as catastrophic events are summarized in Table 1. Landslide often becomes a serious threat for residential and commercial structures, among which transportations, natural resource management, tourism, as well as certain infrastructures, i.e., highways, waterways, pipelines, and others (Pradhan and Youssef, 2009). A landslide is a complex geological phenomenon that involves movement of ground, rock and soil under the effect of gravity (Lim et al., 2011). One or more of the following factors can cause landslide: geological (climate change and rainfall), physical (increased susceptibility of surface soil to failure), human factors (anthropogenic activities, growing urbanization, uncontrolled land-use and increased vulnerability of population and infrastructure) and earthquakes (Laccase and Nadim, 2009; Tatard et al., 2010). Each one of these factors may initiate an immediate landslide by rapidly increasing shear stress, removing lateral support, reducing the strength of slope materials or initiating debris flow activity (Wieczorek and Snyder, 2009).
Major landslides since 1990 (Jaapar, 2006; See-Sew and Siew-Wai, 2008; Low Tian Huat et al., 2012).
Date | Location | Impacts | Reports |
December 11, 1993 | Highland Towers Condominium, Kuala Lumpur | Block 1 collapsed, killing 48 people | There had been ten days of continuous rainfall |
January 6, 1996 | North South Expressway Kampar, Perak | Swept a container truck off the road, killing the co-driver of the truck | There was some accumulation of water at the top of the slope due to earlier rainfall |
November 28, 1998 | Paya Terubong, Penang | Blocked Jalan Bukit Kukus and 17 cars were buried | There were massive land clearances the top of the hill |
January 28, 2002 | Ruan Changkul, Simunjan, Sarawak | 10 houses were destroyed, claiming 16 lives | The district has suffered from bad weather for more than one week |
November 26, 2003 | Bukit Lanjan, New Klang Valley Expressway, Kuala Lumpur | Blocked the entire expressway to the public for more than six months | Landslide occurred immediately after a period of heavy rainfall |
May 31, 2006 | Kampung Pasir, Ulu Klang, Selangor | Four people were killed | There was failure of a retaining wall |
December 27, 2007 | Kampung Baru Cina, Kapit, Serawak | Three people were killed and nine families were made homeless | Landslide occurred after two hours of heavy rainfall |
November 30, 2008 | Bungalow in Ulu Yam Perdana, Selangor | Two sisters were buried alive | The incident has been caused by heavy rainfall |
December 4, 2008 | CIMB Commerce Square and Amanah Raya | Three hundred people were forced to evacuate the buildings | Heavy rain one day before had caused landslide at an adjacent hill |
December 6, 2008 | Taman Bukit Mewah, Bukit Antarabangsa, Ulu Klang, Selangor | 14 bungalow houses were destroyed, five fatalities and injury to 14 people | Prolonged rainfall during the months of October and November |
May 21, 2011 | Madrasah Al-Taqwa orphanage, Hulu Langat, Selangor | Sixteen people (fifteen children and a caretaker) of an orphanage were killed | Landslide caused by heavy rainfall |
Landslide events, including those, which occur in Malaysia, generally occur during rainy seasons or after prolonged heavy rainfalls (Friedel et al., 2006; Huang et al., 2008; Lee et al., 2011; Matori et al., 2011; Pradhan and Lee, 2010; Sezer et al., 2011). Rainfalls cause a change in the moisture contents of the soil, which increase the interstitial pore water pressure, seepage pressure and soil weight as well as instantly reduce cohesion and soil shear strength. The presence of heavy boulders in soil masses also contributes to triggering slide mechanism (Lee et al., 2011; Pradhan and Lee, 2010; Wieczorek and Snyder, 2009). Heavy rainfall was the triggering factor for most of the serious landslide events. For the massive and potentially disastrous landslides, there is no effective and economically viable mitigation scheme. The possible solution is through providing a reliable early warning system that is affordable to the public (Sandra et al., 2011). For an effective early warning system to be developed, extensive monitoring of slope activity over an area and identification of potential failure are vital. This paper is aimed at giving a brief review on the currently available techniques for monitoring and evaluating the stability of soil slopes, which are based on surface measurement, morphology study and stability assessment, followed by a discussion and a review of the technique based on acoustic emission (AE) developed to achieve the same objectives. Owing to its principles of application, it is considered that AE has the capability to provide information for improving the understanding of slope instability. In some documented cases, the AE technique has fundamentally proven itself as being effective in detecting pre-failure processes on soil slopes.
2 Soil slope monitoring method
2.1 Currently available methods for soil slope monitoring
Recently, various methods have been implemented for slope monitoring. They are classified into four main categories, as follows: geodetic methods [i.e. terrestrial laser scanning (TLS) and global positioning systems (GPS)], geotechnical methods [i.e. time domain reflectometry (TDR) and fiber optic sensing (FOS)], geophysical methods [i.e. electrical resistivity technique (ERT) and acoustic emission (AE)], and remote sensing [i.e. synthetic aperture radar (SAR) and geographic information system (GIS)]. The GIS, SAR, GPS and TLS methods are found to be more commonly used for slope monitoring.
The GIS method is the most commonly used. It is useful for subsurface mapping of soil slopes in many countries, including the United States, Japan, Hong Kong, and Malaysia (Ayalew et al., 2005; Dai and Lee, 2002; Mandy et al., 2001; Oh and Lee, 2011; Pradhan, 2010; Pradhan et al., 2012; Sezer et al., 2011; Syed Omar et al., 2004). GIS was found effective in identifying areas that are susceptible to slope failure (Oh and Lee, 2011). Important information about landscape, geology, hydrology, vegetation as well as land usage are readily obtained from field observation and satellite image interpretation (Shafri et al., 2010). However, GIS only acquires surface horizontal motion data that indicate the topographical expression of slope failure. Motion data concerning the slide that has massed down inside the rock and the soil cannot be accessed (Jongmans and Garambois, 2007; Zhu et al., 2011).
The other practical method is SAR, which is an active microwave-based instrument capable of recording the electromagnetic (EM) echo backscattered from the Earth's surface, the dimensions of which are considered as the sensor–target distance and the platform flight direction (azimuth). An advanced version of the SAR system is able to operate for both short- and long-term slope monitoring. The monitoring can be remotely carried out without a direct installation of sensors on the slope, thus, it may provide an effective alternative solution for satellite survey (Pieraccini et al., 2006). Despite its capability, SAR also presents considerable data processing and interpretation difficulties, hindering its popularity to a certain extent (Colesanti and Wasowski, 2006).
Another method of slope monitoring is TLS. The aim of TLS is to record surface data under a wide range of environmental conditions. TLS has become a tool for assessing landslide hazards through monitoring the change of slope surface (Abellán et al., 2010; Akib et al., 2012; Luzi et al., 2009). Since the scanning system of TLS can generate all of the point clouds from the area, any change on the surface can be differentiated by the point clouds coordinates. The major drawback of this system is that there is no standard for describing precision and accuracy. Moreover, generally, the influence of incidence angles, surface reflectivity, and ambient light conditions remain hard to understand (Syahmi et al., 2011).
GPS is another common method used in slope monitoring (Abidin et al., 2007; Calcaterra et al., 2012; Gili et al., 2000; Kondo et al., 1996; Mora et al., 2003; Othman et al., 2011; Újvári et al., 2009). GPS measures displacements to evaluate slope stability. During the survey, monuments are placed on the ground covering a slope area as reference points. The coordinates of the monuments are periodically determined at certain intervals. The characteristics and types of displacement are evaluated by studying the behavior and rate of point change. The results of GPS surveys provide information on the magnitudes of the slope movements in the study area. The method usually produces highly accurate geodetic results. However, the antenna of this system must have a clear access to at least four common satellites. The satellite cannot be effectively employed in jungle areas because its signal can be blocked by trees (Kondo et al., 1996; Othman et al., 2011).
Among GIS, SAR, TLS and GPS methods, the GIS method is the most widely used. The method facilitates classification of slope area (Syed Omar et al., 2004). In order to enhance its reliability, it is therefore required that geotechnical methods, e.g., TDR, inclinometer, and fiber optic sensing and geophysical methods, e.g., seismic methods, be adopted to complete the assessment of slope stability. These methods allow one to obtain more detailed geological and physical descriptions of soil materials as well as to define the vertical boundary of the slope. It is also found that TDR, inclinometers, FOS and other similar geotechnical methods are capable of carring out real time monitoring of slopes. However, these methods are limited to the measurement of slope movements after a disaster has occurred (Jurich and Miller, 1987). Therefore, seismic methods, e.g., AE are considered as good complementary methods to the TDR method. The AE technique is often capable of detecting pre-disaster deformation (Dixon et al., 2003), so that an early warning can be given to allow for evacuation, which may also facilitate capturing of the slope derivation for detailed investigation and effective remedy (Dixon and Spriggs, 2007). AE can also be utilized in seismic survey, which has the potential to provide crucial information for improving the understanding of slope instabilities. Seismic survey can be used to track slope movements (Spillmann et al., 2007). It consists of generally identifying seismic sources induced by slope movements using seismic sensors. The seismic signals can be induced by several mechanisms, such as bending, shearing, rock falls, debris flows or slip at the bedrock interface (Tonnellier et al., 2013). A typical seismic survey system usually involves one or more transducers (sensors), an amplifying system, a filtering system, and a recorder. A system of seismic survey detects a seismic activity associated with initiation, formation, growth and coalescence of slope movements (Amitrano et al., 2005).
2.2 The prospect of acoustic emission (AE) technique for slope monitoring
AE is a non-destructive technique (NDT) of materials health monitoring, which is based on the detection of elastic waves from the surface or the subsurface of the structure under test, and conversion of the waves into electrical signals through coupled piezoelectric sensors (Michlmayr et al., 2012). AE has been implemented for NDT and monitoring in many fields, including pipelines, machines, metal structures, composite structures, concrete structures, and geotechnical materials. The sensor is a primary tool for detecting emissions resulting from mechanical phenomena, such as fracture, friction, and sliding. A simplified representation of the emitted signal as well as of commonly used parameters is shown in Fig. 1. A local material change, giving rise to AE is denoted as an event. The main parameters of AE for analysis purposes are amplitude, count, duration, rise, energy, and threshold level. The maximum amplitude of an event is defined as the peak of the signal. The threshold level is the minimum number that provides the required probability of registering a signal for a specified probability level of false registration, or operation caused by noise. The parameter used to define threshold level in practice depends on the material. The intensity of an AE signal detected by a sensor is considerably lower than the intensity that would have been observed in the close proximity of the source due to the attenuation. Attenuation levels vary from a material to another. AE signals are rapidly attenuated due to the energy loss when the emissions transferred in the material. A material with high attenuation has a higher threshold level than another with low attenuation. There are three main causes of attenuation, beginning with geometric spreading, material damping, the third cause of attenuation being wave scattering (Kaphle et al., 2011; Xiaoli, 2002).
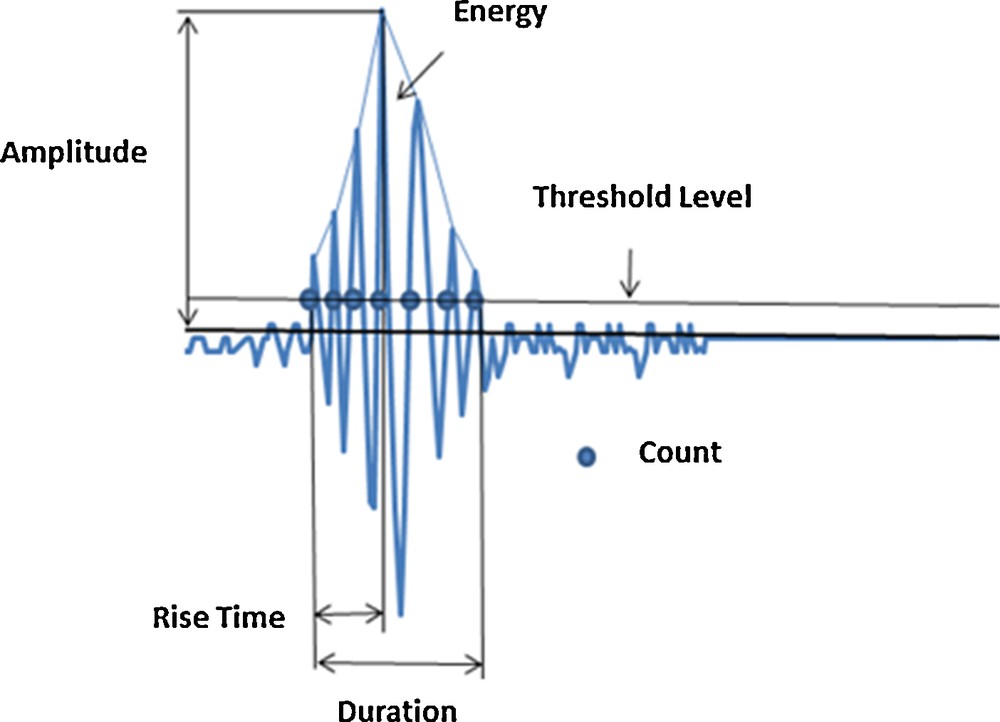
(Color online.) Elements reflecting an AE event.
Since AE signals are emitted through a wide range of frequencies, monitoring systems must be configured according to the investigated material (Hardy and Taioli, 1988). Fig. 2 shows the typical frequency range of AE signals for different types of materials or study purposes. Geological materials have been found to emit AE signals in a broad frequency range, from 10 Hz to 500 kHz. Various types of sensors are available to acquire AE signals from geological structures, ranging from velocimeters, seismometers, geophones, accelerometers, piezoelectric sensors to hydrophones, depending on the conditions on the site and on the specific properties of the material (Dixon et al., 1996).

Frequency range (Hz) of AE monitoring.
Reproduced and modified from Hardy (1972).
In geotechnical monitoring, AE can be used for detecting interparticle friction and displacement along discontinuities in the soil (Dixon and Spriggs, 2007; Dixon et al., 2003). Usually the soil is in equilibrium and in a stable state if there is no emission (Koerner, 1986). If there is an unstable soil volume, the particles constituting the material might scrape each other. As the consequence, this activity releases energy in the form of heat and emissions, known as AE signals (Jurich and Miller, 1987). AE signals are rapidly attenuated in unconsolidated materials like soil due to the energy loss when emissions are transferred from one particle to another. For example, AE signals with a frequency of 30 kHz are attenuated by over 100 dB when they propagate along 100 mm in the sand (Shiotani and Ohtsu, 1999). Therefore, an instrument must be positioned near and close to the source of AE activities. Since the soil in the disaster area usually falls down, a low attenuation acoustic path (waveguide) will be used to transmit the signal to the sensor (Dixon et al., 2003). This study reviews comprehensively the capability of the AE technique employed to monitor slope activity and discusses the distinct advantages of the AE technique for low- (a few Hz to a hundred Hz) and high-frequency (> 1 kHz) measurements.
3 AE measurements
3.1 Instrumentation of AE monitoring systems
The typical components of an AE monitoring system are shown in Fig. 3; they consist of a waveguide for bringing the signals from within the materials to the sensor, a sensor as a transducer for converting elastic waves into variable voltage waves, a pre-amplifier for amplifying the low-level signal detected by the sensor, analogic band-pass filters for improving the signal-to-noise ratio, and a last amplifier for further amplifying the signal for processing and then converting it into a digital signal for storage and analysis (Dixon et al., 2003). In soil slope monitoring, the effective detection of AE signals generated by a deformation of the soil is a challenging task because of the high attenuating characteristics of soil materials. Fig. 4 shows some typical variation of AE attenuation (dB/m) with frequency for a soil, and it includes the attenuation values of other materials for comparison.
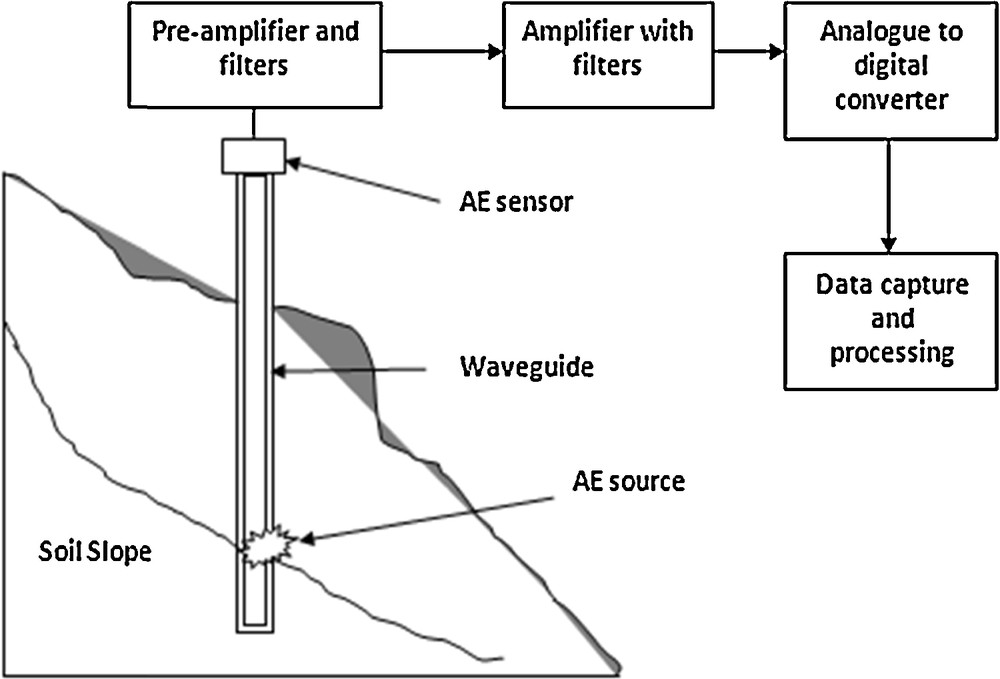
Components of an AE monitoring system.
Reproduced and modified from Dixon et al. (2003).

AE attenuation of some materials.
Reproduced and modified from Koerner et al. (1978).
Due to the attenuation problem, a very limited volume of soil could be monitored at one time. Fig. 5 shows the results of tests conducted by Shiotani and Ohtsu (1999), showing the change of the attenuation trend with regard to the signal's frequency in sand. At 30 kHz, the AE signal is attenuated in an abrupt manner after propagating over 80 mm. This means that only limited AE data would be effectively detected from the host soil. The effective frequency range for AE monitoring was identified to be under 10 kHz for propagation up to 100 mm. The waveguide could be under the form of solid or hollow materials for intercepting potential slope movements. Conceptual diagrams representing both types of waveguide are given in Fig. 6. The waveguide with a solid material can be considered as a low attenuation type, from which AE signals are generated by friction between the waveguide and the soil. The solid materials include steel, aluminum, and stainless steel. Metals are often used as waveguides because of their low attenuation characteristics.

Relationship between AE attenuation in sand and frequency.
Reproduced and modified from Shiotani and Ohtsu (1999).

(Color online.) Waveguide types: (a) solid type and (b) hollow type.
Reproduced and modified from Shiotani (2006).
It is to be noted that a solid type waveguide cannot detect AE signals with a satisfactory sensitivity when there is high acoustic impedance mismatch. This type of waveguide is sometimes identified as a passive waveguide. The hollow type waveguide or active waveguide, on the other hand, is designed to self-generate emissions due to its own deformation when soil movements occur. The sensors are usually positioned on the top of the waveguide. Besides, there are some different set-ups of sensors in the waveguide, e.g., a sensor attached to the internal wall of the pipe and a sensor suspended in the backfill material. For these types of waveguides, hollow metal pipe and materials, such as soil, water, resin, and fiberglass are used as backfill. For example, aluminum pipes and polyvinyl chloride (PVC) pipes were proposed as waveguides by Shiotani and Ohtsu (1999). As a result of the deformation by soil movement, AE signals were generated from the friction between the waveguide and backfill material.
For effective detection of AE signals, the waveguides must be positioned strategically to intercept the potential failure plane. Shiotani (1998) proposed an improvement of a set-up to overcome some issues caused by attenuation and high mismatch in variable soil properties. The set-up was comprised of a waveguide made of a material that prevents sharp attenuation. The merits of the proposed set-up compared to the previous one are as follows: firstly, the specific signal is generated based on the ground's properties and the soil type; secondly, it can determine the source locations owing to the high accuracy of the AE sensors, and finally, it can eliminate the background noise through processing the AE technique.
A pre-amplifier is usually used to amplify the low-level signals detected by the sensor and transmit them to the main amplifier, which is typically located close to the data capture place and the processing hardware, and may be several meters away from the sensor and the pre-amplifier. Band-pass filters are also used to improve the signal-to-noise ratio by removing unwanted low-frequency and high-frequency signals. The acquisition of AE signals is made possible via an analogic-to-digital conversion board linked to a PC, which is used for data storage and signal processing (Dixon et al., 2003).
3.2 Low-frequency AE measurement (1 Hz–1 MHz)
Low-frequency AE response sensors are progressively installed in boreholes for a relatively long period of monitoring, e.g. from a few months to a few years. Low-frequency AE monitoring is usually adopted for observing the microseismic (MS) activities that could be linked to landslides and glaciers or ice quakes. In monitoring the landslide, Spillmann et al. (2007) have completed a pilot MS study of the unstable mountain slope immediately on the huge Randa rockslide scarp in the Swiss Alps. Their MS network of 12 three-component geophones placed in shallow and moderately deep holes was distributed across 60,000 m2 of the rugged terrain. During the period of 31 months, the network recorded signals from 66,409 events, of which only 0.3% were originating from mountain slope micro-earthquakes. Tatard et al. (2010) installed six seismological stations [i.e. New Zealand, California, Grenoble (in the French Alps), Val d’Alry (in the French Alps), Australia, and Wollongong (New South Wales)] to monitor seismic activity of landslide. On the other hand, Tonnellier et al. (2013) presented the characteristics of seismic sources in two clay-shale landslides, which were located in Super-Sauze (France) and Valoria (Italy), through observing the displacement rate in relation to external triggering factors. The results showed that the rate of MS and rock fall activity is associated with the landslide displacement rate after long period of rainfalls.
In glacier or ice quake monitoring, low-frequency AE measurements have also been introduced by some researchers. For example, Neave and Savage (1970), who studied seismicity related to surface fracturing on the Athabasca glacier. The study revealed that the glacier was caused by a wide variety of sources and physicals origins, ranging from surface events to crevassing processes. Anandakrishnan et al. (1998) and Wiens et al. (2008), on the other hand, also observed seismic activity related to stick-slip motions at the bed of Antarctic ice streams. Meanwhile, O’Neel et al. (2007) deployed an array of 11 seismometers across the lower 20 km of glacier for a period of 12 months to remotely monitor and automatically detect ice quakes generated by the Columbia glacier. The results showed that seismic observations yield a direct measurement technique for mechanical calving at the glacier. Monitoring of the temporal evolution of fracture process of glaciers and their dependence on glacier flow and glacier hydrology was studied by Roux et al. (2010). They showed that some portions of the glacier generated a compressed zone with the strain axes, which are parallel to the glacier flow. Based on the previous studies presented herein, it was found that the accuracy of the detection of events and of their location was highly essential to assign seismic activity to glaciers or failure processes.
Seismic signals are difficult to identify and distinguish from other signals because of the presence of external noises that come from certain sources, such as rainfalls, water streams, winds, and operating electrical equipments, apart from a high attenuation exhibited by slope materials, such as clays, shales, and marls (Tonnellier et al., 2013). Some recent monitoring techniques have been developed to offset the effects from external noises and material attenuation, for example, by identifying the useful signal through the analysis of the variation with time of signal frequency contents based on spectrograms. The detection of MS is based on the evaluation of seismic signals and spectrograms of the signal simultaneously. The amplitude of signal was obtained through estimating the intensity of spectrogram stacked in the frequency range (Helmstetter and Garambois, 2010).
3.3 High-frequency AE measurement (>1 MHz)
There are two major applications of high-frequency AE measurement: rockslide and landslide monitoring. In rockslide monitoring, a prospective method to install waveguide for AE waves due to rock deformation (WEAD) was developed, which demonstrated its promise in monitoring AE activity due to rock deformation (Shiotani et al., 2001). Through this application, in situ measurements suggested a correlation between AE activities with the strain increases in boreholes. The method seemed to be capable of evaluating the final failure (Shiotani, 2006). Most recently, Cheon et al. (2011) proposed an improved monitoring apparatus and method to evaluate the damage level by the AE technique, which is completed with a waveguide and a wave generator. Besides that, there are some references on AE monitoring without waveguide. Amitrano et al. (2005) installed five seismic stations for monitoring a natural chalk cliff (Haute-Normandie, France) before collapse. They observed a tremendous rising of seismic activity and energy a few hours prior to the fall. Got et al. (2010) monitored the displacement and seismic activity of an unstable rock column in a natural limestone cliff (Vercors massif, French Alps) during 3 months preceding its failure. On the other hand, Gaffet et al. (2010) developed a seismic monitoring system and used it to investigate the La Clapière unstable mountain slope in the southern French Alps. A sensor network composed of four seismometers was installed over the territory of 1.5 km2 for a period of four months. Most of the above references have been applied to the slopes using geophones or accelerometers.
In monitoring landslide activities, the feasibility of using high-frequency AE for slope monitoring has been principally investigated at the laboratory level through applying waveguides as wave transmitters for the sensors. Jurich and Miller (1987) nevertheless demonstrated the applicability of high-frequency AE in monitoring the failure of three actual slope failures. In utilizing high-frequency AE for slope monitoring, Koerner et al. (1981) proposed a classification scheme for the status of the AE signal, which is linked to slope deformation rates and states. In short, when there is no emission, the slope is basically considered as intact or as having suffered no deformation. The emission rate will increase together with the increase of deformation. Recently, the interpretation of AE data has been qualitative and mainly used as a coarse prediction for slope stability. There was however a lack of study about the propagation behavior of AE signals and potential different waveguide configurations for effective quantitative evaluation schemes.
Some researchers applied steel waveguides to the soil embankment. Jurich and Miller (1987) presented an analysis of three active landslides using the AE technique with steel waveguides (Fig. 7). The results showed that the measurement set-up successfully detected pre-failure activities of soil slope. The number of AE counts, AE events, and the average ratio of counts per event are described as key aspects for the quantification of slope stability, as exemplified in Fig. 8. Based on the figure, the number of AE activities, i.e. counts and events in the months of February and March were relatively low. The AE activities showed increases in March and April, where changes in the stability of the slopes were observed. The stars in the figure indicate the initiation of slope movements. It could be seen that the AE activities continued to fluctuate, but in general show an increase in their number compared to the preceding ones. These trends suggested that the occurrence of AE activities could be considered as a function of the failure mode of the slope.
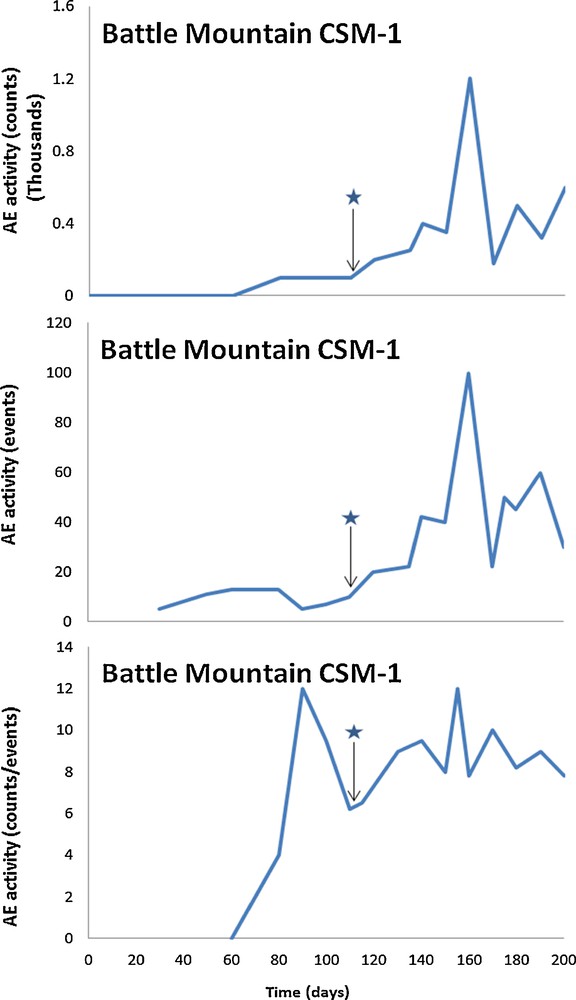
(Color online.) Sample measurement of AE counts, events, counts/event ratio data plot.
Reproduced and modified from Jurich and Miller (1987).
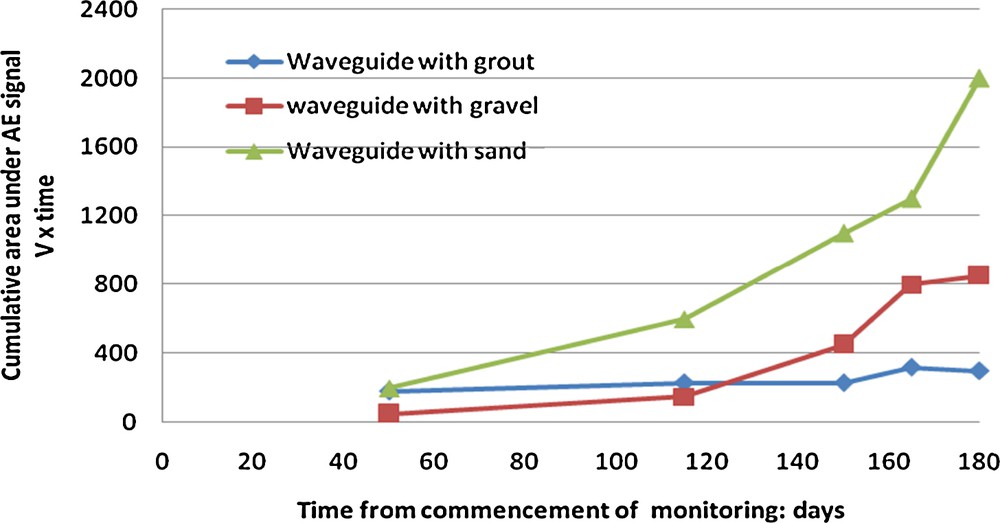
Cumulative area under the AE signal for waveguides 1, 2 and 3.
Reproduced and modified from Dixon et al. (2003).
Chichibu et al. (1989) monitored an embankment and an unstable natural slope using the AE technique. The measurement indicated that the period of AE count rate occurrence coincided with the movements of the embankment. In this natural slope, the significant increases in AE count rates were recorded at least two months before the observation of detectable ground movements. In another research study, Rouse et al. (1991) monitored slope failure from two sides. Four distinct types of AE signals have been recognized so far, with frequencies ranging from 1–7 kHz, with particular peaks at 1.2, 2.0, 4.2, 4.9, 5.5 and 6.5 kHz. AE events were also detected using steel waveguides embedded in the slope. In the measurements, changes in signal characteristics during heavy rainfalls were also observed. The results suggested that the AE technique could be an effective means for an early warning of slope failure events.
Previous studies [i.e. Jurich and Miller (1987), Chichibu et al. (1989) and Rouse et al. (1991)] have successfully detected the pre-failure of soil slopes by passive waveguides. However, the passive waveguide was ineffective in suppressing transmission loss between the soil and the outer position of the waveguide. The waveguide was also ineffective in decreasing attenuation. Therefore, some researchers used active waveguides for monitoring. Active waveguides consist of metal or non-metal acoustic tubes with a type of soil backfill placed inside or around the tube. The active waveguide has the advantage in such a manner that the generated AE signals suffer less attenuation before reaching the sensor, because of the tight association of the backfill with the waveguide (Dixon et al., 1996; Spriggs and Dixon, 2005). Shiotani and Ohtsu (1999) presented an approach for predicting the failure of soil slope using active waveguides. In their experiment, it was revealed that optimum AE signal could be obtained within a 5-cm propagation distance from 50 kHz. They adopted a polyvinyl chloride (PVC) pipe as a waveguide and AE sensors were suspended inside the pipe, which was filled with water. A new method was proposed to determine the b-value in real time from the peak-amplitude distribution of the acquired AE waveforms. The proposed b-value was a sensitive parameter to the slope conditions and suitable for predicting of slope failures. The curve fitting technique and the rate process analysis were also implemented in order to evaluate the AE activity at the time of slope failure. It was found that the results of the rate process analysis of AE activity provided more promising results than the curve fitting technique.
In another study, Dixon et al. (2003) also introduced the use of active waveguides that were composed of steel tubes containing two different backfill materials, i.e. sand and gravel installed in boreholes. The steel tube waveguides were used because they facilitate lamb waveforms to be transmitted at low attenuation. Simple laboratory and field trial experiments were designed to validate the model with active waveguides. In the laboratory experiment, a length of steel tube was located in a soil-filled rigid plastic box. The box was filled with sand or medium gravel, both of which were used as backfills in the field trials. The next step was by attaching a sensor to the top of the tubing. Two field trials were conducted at a coastal slope at Cowden, England, and at a brick slope at Arseley, England. The results indicated that there was a relationship between AE rates and deformation rates of soil slope. It also indicated that an active waveguide with relevant signal processing methods could be used to provide an early indication of the instability of the slope. The figure shows the signal processing result in the form of the cumulative area under the AE signals recorded for different types of waveguide backfill. The area under the AE signals provided an indication of the AE energy, which would increase together with the increase in the rate of displacement. The figure shows different behaviors between the three backfill materials. A waveguide backfilled with sand has recorded the highest response of AE, followed by that with gravel and bentonite. These findings were in agreement with that obtained from test models using the active waveguides. The sand backfill gave a higher response than gravel, because it remained unstable for a longer period following the destabilization event. A relationship was successfully established between the AE signal with an active waveguide and the rate of the slope displacements (Dixon et al., 2003).
Dixon and Spriggs (2007) extended the study by detailing a quantification method of displacement rate of the slope using an active waveguide. A series of laboratory experiments were presented to define the relationship between AE event count rate and displacement rate. It was found that the event rates directly related to the displacement rate over a range that was in line with the standard of slope movement classification (i.e., 0.001–1 mm/min). It was also observed that all the data sets gave consistent and repeatable trends in accordance to their displacement rate groups, i.e. rapid rate (1.0125 mm/min), moderate rate (0.098 mm/min), slow rate (0.01764 mm/min) and very slow rate (0.00176 mm/min). The clear banding of data provides a mechanism for differentiating the magnitude of the displacement rate using AE event rates. The slower the displacement rate, the longer the time until reliable data was obtained (i.e. time taken to achieve 1 mm of displacement). It was possible to predict the slope displacement rate using the gradient of AE event rate. In addition, it was possible to detect a change in the displacement rate within 2 min even at very slow rates (i.e. 0.0018 mm/min). Based on the active waveguide, the quantification of AE is necessary for estimating the deformation rate. Based on this, the status of the soil slope can be classified as proposed in Table 2. In addition, the change of the deformation was identified within a few minutes of the occurrence of the change, even at very slow rates (Dixon and Spriggs, 2007). The quantification of the increase in the deformation rate provided a key indicator of slope instability. The instability was potentially due to the occurrence of large movements. The AE monitoring has been sufficiently sensitive, serving as an early warning for the progressive development of slope failure.
Assessment of slope displacement rates and state using quantified AE generated by active waveguide (Dixon and Spriggs, 2007).
Slope deformation rate (mm/min) | Gradient of AE event rate (events/min2) | State of slope | |
Rapid | > 1 | > 100 | The slope is undergoing a large deformation State of failure Urgent need for public safety measures |
Moderate | 0.1 | 100 to 1 | Substantial deformation and considered unstable Immediate remedial and public safety measures are required |
Slow | 0.01 | 1 to 0.01 | Deform slightly but marginally stable Continued monitoring is necessary |
Very slow | < 0.001 | < 0.01 | The slope can be considered essentially stable |
4 Conclusions
The AE technique has been found to be a very effective method for monitoring and detecting fracture and failure of materials. Despite the lack of its practical use, this technique exhibited promising application for monitoring and assessing the stability of soil slopes. More studies are deemed essential to develop and to refine the methodology that caters such purposes. As a result of this review, it has been found that the failure of the slope could be detected and monitored with small quantities of AE sensors, even when the slope movements are minimal and the failures are at their preliminary stage. Realizing its potential, the AE technique can be utilized for developing an effective early warning system for slope failure, by providing an indication of an incoming failure to facilitate early evacuation and other countermeasures that can minimize loss. Several methods for analyzing AE data should be employed for reliable monitoring and evaluation of slope stability. The number of different AE parameters, i.e. counts, events and the ratio of average counts per event, describe the various aspects of the quantity as well as the duration of AE activity, which can be interpreted to assess slope stability. An accurate record of the changes in slope stability depends on the frequency of the observation. Continuous monitoring of the AE activity is always desirable to provide the most detailed record on the changes in slope stability. The interpretation of AE data has been carried out both in qualitative and quantitative ways. A sensitive measurement and monitoring instrumentation as well as a comprehensive benchmarking scheme would provide an effective solution to establish a reliable and robust early warning system for soil slope failure. Some researchers have developed an evaluation scheme that quantifies data as “moderate”, ‘“high” and “very high” levels of AE activity. This scheme would enable AE trigger levels to be established and linked to appropriate measures. The main challenge in this case consists in obtaining data from deforming soil bodies, which are deep lying due to a high level of attenuation and to signal contamination by ambient noise.
Acknowledgments
This work was supported by AUN/SEED-Net CRI 2011.
Vous devez vous connecter pour continuer.
S'authentifier