1 Introduction
Unraveling the timing and dynamics of mountain building is a long-standing goal in collisional orogen studies. This goal is traditionally addressed by tectonics-sedimentation analysis of synorogenic deposits and, more recently, by low-temperature thermochronology on the assumption that dated exhumation paths reflect the vertical component of the evolution of thrust belts. In the Pyrenees, the foreland basin record is well known, and thermochronology studies have focused in the past decades on the Paleozoic massifs of the Axial Zone (Fitzgerald et al., 1999; Gibson et al., 2007; Gunnell et al., 2009; Jolivet et al., 2007; Maurel et al., 2008; Metcalf et al., 2009; Morris et al., 1998; Sinclair et al., 2005) (Fig. 1A). More recent thermochronology studies have included the Cenozoic sedimentary rocks of the South Pyrenean foreland basin and the Paleozoic and Mesozoic rocks of the North Pyrenean Zone, to better constrain the relationships between the exhumation in the Axial Zone and the exhumation and burial in the adjacent basins (Beamud et al., 2011; Fillon and van der Beek, 2012; Fillon et al., 2013; Meresse, 2010; Mouthereau et al., 2014; Rushlow et al., 2013; Vacherat et al., 2014; Whitchurch et al., 2011).
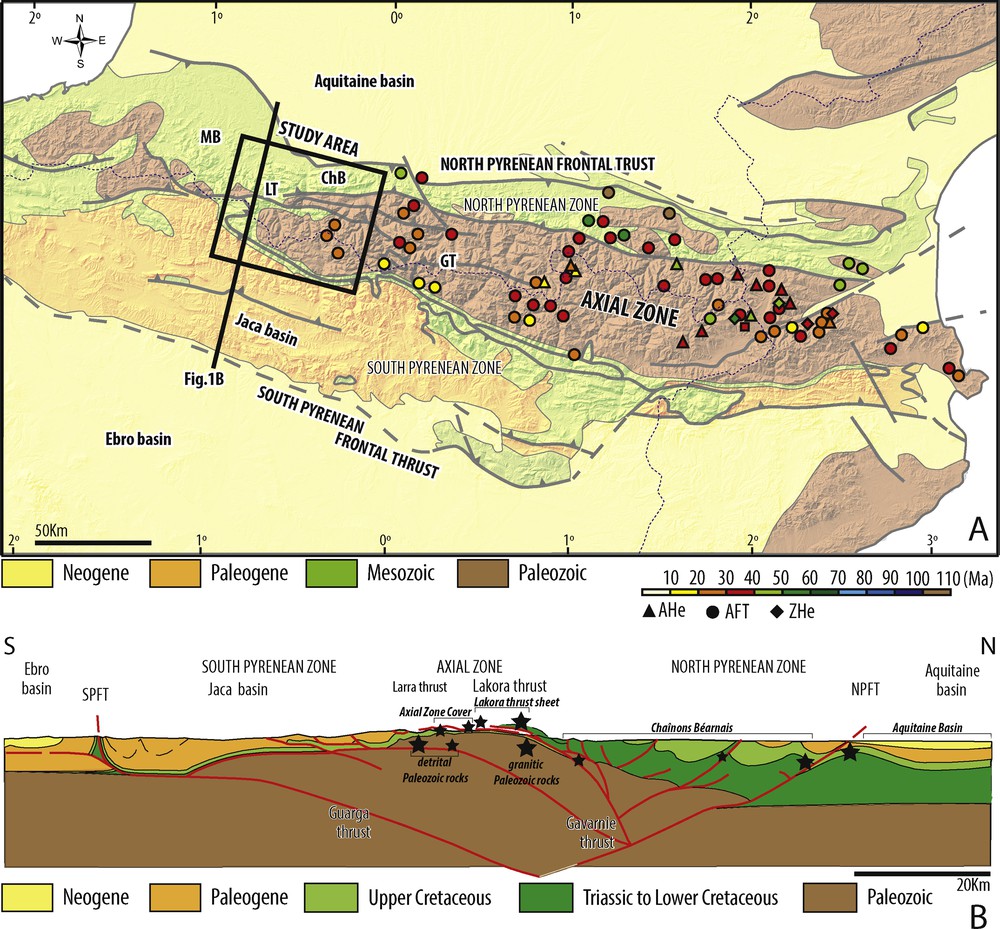
A. Geological sketch of the Pyrenees with previous thermochronology results obtained in the Axial Zone (Fitzgerald et al., 1999; Gibson et al., 2007; Gunnell et al., 2009; Jolivet et al., 2007; Maurel et al., 2008; Metcalf et al., 2009; Morris et al., 1998; Sinclair et al., 2005). MB: Mauleon basin; LT: Lakora Thrust; ChB: Chaînons Béarnais; GT: Gavarnie Thrust. B. Simplified cross-section across the west-central Pyrenees showing the main tectonic units discussed in this work and projected structural locations of the studied samples (large stars: several samples; small stars: single samples; Ansó-Arzaq transect of Teixell, 1998). Masquer
A. Geological sketch of the Pyrenees with previous thermochronology results obtained in the Axial Zone (Fitzgerald et al., 1999; Gibson et al., 2007; Gunnell et al., 2009; Jolivet et al., 2007; Maurel et al., 2008; Metcalf et al., 2009; Morris ... Lire la suite
While most of the thermochronology studies focus on the eastern and east-central Pyrenees, often around the ECORS-Pyrenees profile, the western Axial Zone and adjoining areas have been less investigated. To understand the relationships between the exhumation in the Axial Zone and the dynamics of the fold and thrust belt in the forelands, the western Axial Zone gives key information; this part of the basement interacts to the south with the Tertiary Jaca basin, which contains the most complete foreland basin sequence recording the structural development (e.g., Barnolas and Teixell, 1994; Cámara and Klimowitz, 1985; Labaume et al., 1985; Millán et al., 2000; Teixell, 1996; Teixell and García-Sansegundo, 1995).
This work presents the first multi-method thermochronology database of the western Axial Zone of the Pyrenees (Fig. 1A), including apatite fission track (AFT), (U–Th)/He in zircon (ZHe) and apatite (AHe) data, with the aim to investigate the Pyrenean Cenozoic evolution. A few samples of the adjacent North Pyrenean Zone (Chaînons Béarnais area) are also included in the study. The area investigated is particularly interesting because it comprises the western termination of the Axial Zone Paleozoic units where it plunges under Upper Cretaceous and Paleogene rocks. It also constitutes the only area where can be observed the Cretaceous North Pyrenean basin overthrusting the Southern Pyrenees (Teixell, 1990, 1998). The thermochronology data obtained are compared with the tectonostratigraphic record of the foreland basins and integrated in the tectonic framework of the west-central Pyrenees, providing a more complete picture of the history of thrust uplift and exhumation (both syntectonic and post-tectonic) of this segment of the chain.
2 Geological setting
The Pyrenees formed from Late Cretaceous to Early Miocene times due to convergence between the Iberian and European continental margins (Choukroune et al., 1990; Muñoz, 1992). As a result of the collision, the west-central Pyrenees rose as a doubly-verging orogenic prism built by basement and cover-involved thrusts. This collision belt is underlain by north-directed lower crustal subduction (Lagabrielle et al., 2010; Teixell, 1998; Teixell et al., 2016). The main upper crustal structures of this segment of the chain are shown on Fig. 1B. The North Pyrenean Zone was a rapidly-subsiding Cretaceous basin between the European and Iberian margins, floored by hyper-thinned continental crust and mantle exhumed during Albian–Cenomanian times (Jammes et al., 2009; Lagabrielle and Bodinier, 2008). This basin is now completely inverted, overthrusting to the north the Aquitaine Basin along the North Pyrenean Frontal thrust, and to the south along the Lakora thrust (Fig. 1).
The North Pyrenean Zone in the studied Chaînons Béarnais area contains thick and relatively complete Jurassic and Cretaceous successions and is internally deformed into a system of folds and thrusts detached in the Triassic Keuper facies (Lagabrielle et al., 2010). The Lakora thrust crops out as a gently-dipping fault, largely parallel to bedding in the Upper Cretaceous cover of the Axial Zone (the footwall), and carrying a thin thrust sheet of Paleozoic, Triassic and Middle–Upper Cretaceous rocks in its hanging wall (Lakora klippe and Iguntze–Mendibelza massifs, Fig. 1; Teixell, 1990, 1996). Eastward, the Lakora thrust passes laterally to various thrusts also carrying thin Paleozoic basement slices located at the southern edge of the Chaînons Béarnais (e.g., Eaux-Chaudes and Cinq-Monts thrusts; Ternet et al., 2004). The Lakora thrust and these eastern extensions derive from the inversion of extensional structures in the upper Iberian continental margin (Teixell et al., 2016).
The southern part of the west-central Pyrenees is characterized by south-verging thrusts and includes (1) the Axial Zone, a basement antiformal culmination caused by the Gavarnie thrust, and (2) the Paleogene Jaca basin, a large-scale asymmetric synform between the Axial Zone and the South Pyrenean Frontal thrust (Fig. 1).
Paleozoic rocks of the Axial Zone are unconformably covered by Upper Cretaceous shelf carbonates. A branch of the Lakora thrust, the Larra thrust, propagated across Upper Cretaceous–Eocene rocks of the Axial Zone cover and the northern Jaca basin. East of the study area, the Axial Zone comprises stacked basement thrust sheets that caused a greater structural relief and a large basement exposure (e.g., Muñoz, 1992; Roure et al., 1989). There, the northern boundary of the Axial Zone is marked by the North Pyrenean Fault, a steeply-dipping structure with complex kinematics that passes westward to a south-verging décollement at the southern edge of the Chaînons Béarnais. The westward plunge of the Axial Zone in the study area provides constraint on the structural relief and shape of the top of the Axial Zone and on the relationships between the main structural units. Non-exposed basement thrusts underlie the Jaca basin and cause major variations of structural relief (e.g., the Guarga thrust, Fig. 1B; Cámara and Klimowitz, 1985; Labaume et al., 1985; Teixell, 1996; Teixell and García-Sansegundo, 1995).
Pre-orogenic Mesozoic successions in the Jaca basin are relatively thin and incomplete; in contrast, the Paleogene infill is very thick (up to 9 km) and conforms to a typical flysch-to-molasse foreland basin sequence (Mutti et al., 1988; Puigdefàbregas, 1975). Tectonics–sedimentation relationships indicate a piggy-back sequence of thrusting from the Lakora to the Guarga thrusts that spans the entire Pyrenean orogeny. The Lakora thrust probably initiated in the Late Santonian, as indicated by flexure in its footwall sediments, and its main activity continued until the Middle Eocene (Bartonian). This includes the footwall splays of the Larra thrust and the laterally equivalent Eaux-Chaudes thrust (Teixell, 1996). The Gavarnie thrust was active from the Late Eocene to the Early Oligocene, whereas the Guarga thrust took up final compressive deformation from the Late Oligocene to the Earliest Miocene (Teixell, 1996).
The chronology of the North Pyrenean thrusts is less known. The internal structures of the Chaînons Béarnais were initiated during the Late Jurassic–Early Cretaceous as diapiric salt walls in extensional context (Canérot, 1985; Teixell et al., 2016), but their evolution in the Pyrenean orogeny is less constrained in time. The North Pyrenean Frontal thrust appears as a long-lived structure partly contemporaneous to the Lakora thrust and extending until more recent times. Indeed, in the study area, growth strata in its footwall syncline indicate thrusting beginning in Campanian–Maastrichtian times and continuing during the Paleogene (Poitevin et al., 2014), and thermochronologically-constrained cooling in the Mauléon segment of the North Pyrenean Zone, to the west of the study area, begun some 50 Ma ago (Vacherat et al., 2014). In spite of this early thrusting activity, the molasse deposition in the Aquitaine basin derived from the Pyrenean reliefs spans from the Late–Middle Eocene to the Miocene (Biteau et al., 2006).
The extent to which the described sequence of thrusts is reflected in the exhumation history is not known yet. Northeast of the Jaca basin, AFT in the granites of the Axial Zone, yield Cenozoic cooling ages (e.g., Néouvielle and Bielsa massifs; Jolivet et al., 2007), but the degree of post-Variscan reset and the amount of exhumation of the westernmost Axial Zone and the Lakora thrust are unknown. In spite of the rich tectonostratigraphic record of the Jaca basin, discrepancies remain for the timing of some major structures. Muñoz et al. (2013) recently attributed the emplacement of the Gavarnie thrust sheet to the Middle Eocene, on the basis of a correlation between the basement thrust and the growing and rotating cover structures in the Aínsa basin. The previous attribution of the Gavarnie thrust to more recent times was based on the refolding it produced in the overlying Larra–Monte Perdido thrust, which was linked to the Boltaña anticline, in turn dated as Late Lutetian to Bartonian (Teixell, 1996).
A Late Eocene to Oligocene age for the Gavarnie thrust has also been favored by Jolivet et al. (2007) on the basis of AFT ages of ca. 35 Ma of the thrust hanging wall at high elevation in the Néouvielle granite. On the other hand, AFT ages around 20 Ma dominate the southernmost Axial Zone in the central Pyrenees, in the footwall of the Gavarnie thrust (Fitzgerald et al., 1999; Jolivet et al., 2007; Sinclair et al., 2005). In post-tectonic times, an acceleration of exhumation rates at 9 Ma was detected by AHe modeling in the eastern part of the South Pyrenean foreland basin (Fillon and van der Beek, 2012). To date, this event has not been reported in the Axial Zone except for a 10.9 ± 1.0 Ma sample obtained by Jolivet et al. (2007) in the Bielsa massif.
3 Sampling and methods
A total of 18 samples were collected for ZHe, AFT and AHe studies and five more samples from Meresse (2010) were used to complete the dataset (see location on Fig. 2). Five samples were taken in the Paleozoic granites of the Balaitous–Panticosa and Eaux-Chaudes plutons, and three in Paleozoic detrital rocks to the west to unravel the timing of exhumation of the western Axial Zone. These were complemented with the five previous AFT results from Meresse (2010) and new He data made on these samples. To gain insight into the activity of the Lakora thrust, two samples were taken in Upper Cretaceous and Lower Eocene turbiditic sediments from the footwall (Axial Zone cover) and three in Albian conglomerates from the hanging wall. To complete the study to the north, four samples were taken in the Chaînons Béarnais (NPZ) and two in the Aquitaine basin, in the footwall of the North Pyrenean Frontal Thrust.
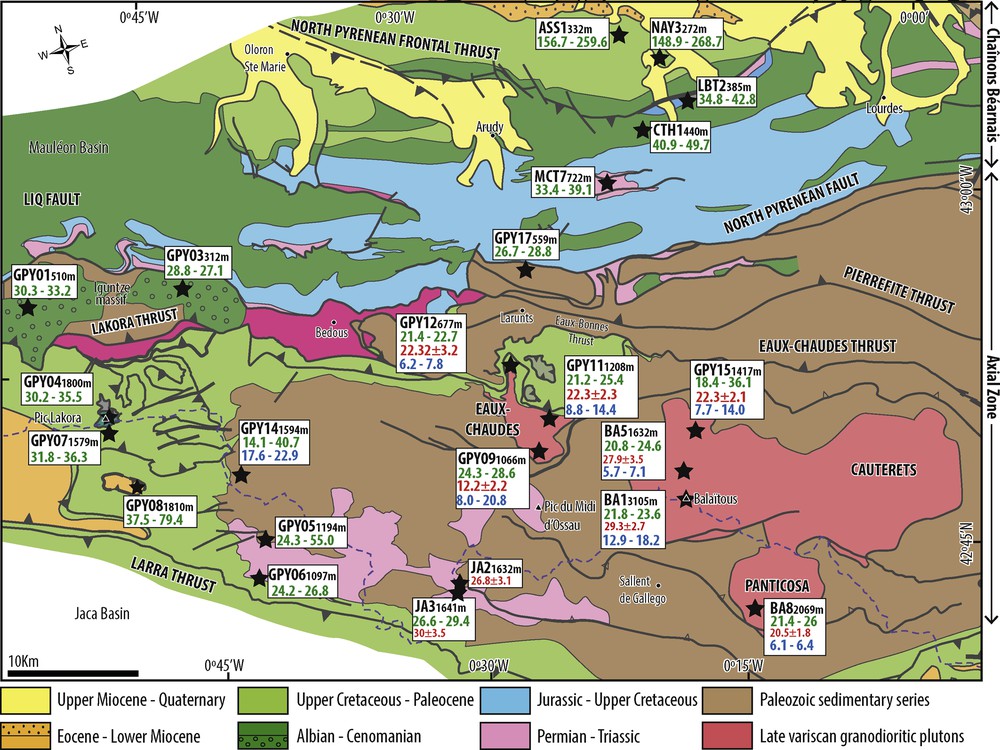
Map of the western Pyrenean Axial Zone and adjacent Chaînons Béarnais, showing location of samples and thermochronological results; zircon (U–Th)/He (ZHe) results in green, apatite fission track (AFT) in red and apatite (U–Th)/He (AHe) in blue. For He data, we indicate the age ranges (for further information, see Tables 1, 2 and 3 in Annex 1, online supplementary data). The blue dashed line indicates the drainage divide.
The nine samples that contained a sufficient number of high-quality apatites were analyzed for fission tracks and/or (U–Th)/He (Tables 1 and 2 in Annex 1, online supplementary data). Zircon grains could be retrieved from most samples, and ZHe analyses were performed on 18 samples (Table 3 in Annex 1, online supplementary data). Sample JA2 did not provide zircon suitable for analysis. The data obtained allowed thermal modeling of four profiles in the granitic massifs of Balaitous–Panticosa and Eaux-Chaudes and in the Lakora thrust sheet.
The AFT analyses were performed following the procedure described by Jolivet et al. (2007). The mounted samples were sent to Oregon State University for irradiation. Ages were calculated using an overall zeta value of 344 ± 2 a·cm2 (GVB) obtained on both Durango (McDowell et al., 2005) and Mount Dromedary (Green, 1985; Tagami, 1987) apatite standards.
Apatite (U–Th)/He dating measurements were performed at Geosciences Montpellier, following the procedure described in Romagny et al. (2014). Prismatic apatites were selected, with two or no pyramids and sizes ranging from 50 to 200 μm. For the samples from the Balaitous–Panticosa pluton, two to three apatite grains of the same size were used for each aliquot with the exception of sample GPY15 for which single crystals were dated. In the Eaux-Chaudes pluton, only two aliquots from sample GPY09 contained two grains.
Zircon (U–Th)/He dating was carried out at the University of Texas-Austin using laboratory procedures described in Wolfe and Stockli (2010). Individual ages were calculated using standard α-ejection corrections (e.g., Farley et al., 1996; Farley, 2002) and reported age uncertainties of about 8% (2σ) are based on the reproducibility of replicate analysis of laboratory standards (Farley et al., 2001; Reiners et al., 2004). Both uncorrected and α-ejection corrected ages are reported (Table 3 in Annex 1, online supplementary data).
4 Results
In what follows, data are organized according to the structural position of the samples in the different tectonic or lithologic units (Fig. 2). For ZHe ages, we present age-elevation plots and ages versus Ue plots in Annex 1 (online supplementary data).
4.1 The Chaînons Béarnais and the Aquitaine Basin
The sample set of the Chaînons Béarnais and the Aquitaine Basin consists of terrigenous rocks of Carboniferous to Cretaceous age that provided only zircon crystals suitable for analysis. In the Aquitaine Basin, samples ASS1 and NAY2 are poorly cemented sandstones of Campanian and Maastrichtian, respectively, and yield a dispersion of ZHe ages older than the depositional age, ranging between ∼ 150 and ∼ 270 Ma (for error margins see Table 3 in Annex 1, online supplementary data), indicating no reset after deposition. In the Chaînons Béarnais, ZHe ages range between 26 and 50 Ma, which is younger than the depositional ages, and attest for exhumation in the hanging wall of the North Pyrenean Frontal thrust. Sample CTH1 yields the oldest age range (41–50 Ma) in accordance with its highest structural position in an Albian–Cenomanian syncline. The southernmost sample GPY17 from the Carboniferous sandstone of the Chaînons Béarnais basement yields the younger age range, between 26 and 29 Ma, in spite of a higher elevation. Samples from the Permian–Triassic red beds (LBT2 and MCT7) yield intermediate ages between 33 and 42 Ma.
4.2 The Lakora thrust sheet
Three samples from the Albian Mendibelza conglomerate (Boirie and Souquet, 1982) provided zircons suitable for analysis, but no apatites. The three samples provide ZHe ages in the Late Eocene–Early Oligocene interval, ranging from 27 and 29 Ma at the lower altitude (312 m, GPY03) to 30 and 35 Ma at higher altitude (1800 m in the Lakora klippe, GPY04).
4.3 The Axial Zone cover
In the post-Variscan cover of the Axial Zone (hanging wall of the Gavarnie thrust), a Maastrichtian turbiditic sandstone (GPY07, 1579 m) provides ZHe ages of 31–36 Ma, younger than the depositional age and strikingly similar to the age in the Lakora thrust sheet just above (Fig. 2). A sample of Lower Eocene flysch located ∼ 5 km to the south (GPY08, 1810 m) yields a wide dispersion of ZHe ages (37–80 Ma), some of them older than the depositional age, indicative of partial reset.
4.4 The western Axial Zone: detrital Paleozoic rocks
Samples from the westernmost Paleozoic exposures of the Axial Zone also belong to the hanging wall of the Gavarnie thrust and comprise Carboniferous and Permian sandstones that provided apatite and zircon crystals with large age dispersion. Samples JA2 and JA3 of the southern Axial Zone near the Somport pass yield comparable AFT (Meresse, 2010) and ZHe central ages that may indicate rapid cooling at 25–30 Ma, as do some zircon crystals from samples GPY05 and GPY06 from the Upper Aragón Subordán valley. The latter samples show however a greater dispersion, as does Carboniferous sandstone from the Lescun area further north (GPY14), whose age dispersion ranges between 14 and 52 Ma (Fig. 2 and Table 3 in Annex 1, online supplementary data).
4.5 The western Axial Zone: the Eaux-Chaudes and Balaitous–Panticosa plutons
These granitic bodies provided apatite and zircon crystals that were suitable for AFT, AHe and ZHe analyses. Most of the samples show ZHe ages independent of the eU concentration, suggesting complete reset (see Annex 1). In the Eaux-Chaudes pluton, samples GPY11 and GPY12 yield similar ZHe and AFT ages that may be indicative of rapid cooling between 20 and 25 Ma, further attested to by the similarity in age between the samples in spite of a difference in elevation of 531 m. Sample GPY09 at an intermediate altitude yields a ZHe age of 24–28 Ma, while the AFT age is significantly younger, around 12 Ma (Figs. 2 and 3, Tables 1 and 3 in Annex 1, online supplementary data). In the Balaitous–Panticosa granites, ZHe ages are also markedly clustered at 20–25 Ma for different elevations. However, samples BA1 and BA5 from the Balaitous mountain give AFT ages of 28–29 Ma (Meresse, 2010), slightly older than the ZHe ages obtained for the same samples in this study (Table 3 in Annex 1, online supplementary data). Sample GPY15 at lower elevation yields an AFT age of 18 Ma, whereas ZHe ages are more dispersed (18–36 Ma). The AHe ages obtained for this set of samples range between 21 and 6 Ma, with a cluster between 6 and 10 Ma, again younger than the AFT ages (Fig. 3 and Table 2 in Annex 1, online supplementary data), but with similar age if we take into account the 2σ errors of both AFT and AHe data.
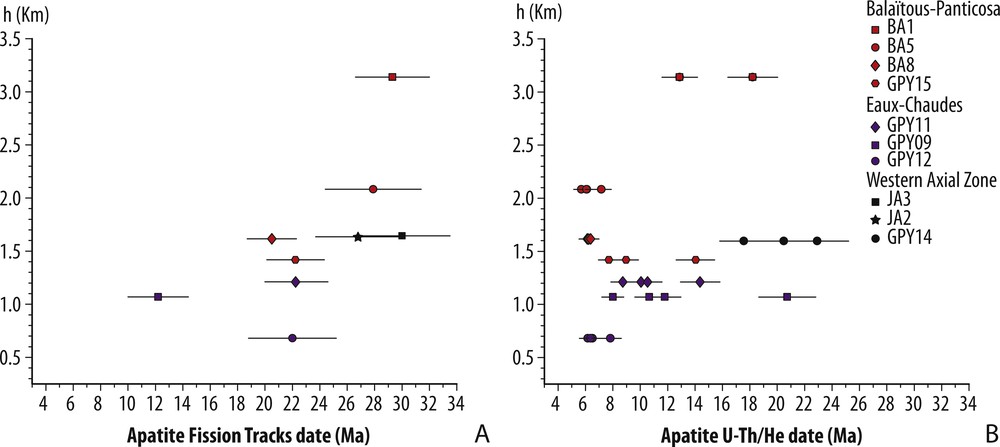
Apatite fission track (AFT) age-elevation plot of the Eaux-Chaudes and Balaitous–Panticosa granodiorites dataset (in red), and of the GPY14 sample from Paleozoic sediments in the westernmost Axial Zone (black).
5 Thermal modeling
Thermal history modeling was performed using QTQt software (Gallagher, 2012; Gallagher et al., 2009). For AFT modeling of the only sample with confined track lengths (GPY11), we used Ketcham et al.’s (2007) multikinetic annealing model, with the Dpar parameter as kinetic constraint. (U–Th)/He ages were modeled using a spherical diffusion domain (based on the crystal's equivalent spherical radius), and taking into account eU-dependent radiation damage modulated diffusivity for He diffusion, following the models of Flowers et al. (2009) and Guenthner et al. (2013). In cases where AHe ages were obtained by multigrain aliquots, we did not use a specific diffusion model. Models were run for the sub-vertical profiles at the Balaitous–Panticosa and Eaux-Chaudes granites and at the Lakora thrust sheet. All models were forced to be at surface temperature at present time and we allowed the temperature offset between samples to vary through time in a range equivalent to geothermal gradients of 15 to 35 °C/km.
For the Balaitous–Panticosa and Eaux-Chaudes granites, independent constraints derived from field geology (unconformity of the Cenomanian carbonates above Paleozoic rocks of the Axial Zone) were used as input parameters in the thermal models to force the cooling curves to pass near the surface in Cenomanian times. The profile in the hanging wall of the Lakora thrust was constrained to pass near the surface in Albian times (stratigraphic age of the Mendibelza conglomerate), whereas a model of the westernmost Axial Zone (Paleozoic rocks and their cover) was constrained to be at shallow levels from the Cenomanian to the Early Eocene.
Models of the Eaux-Chaudes and Balaitous–Panticosa sub-vertical profiles show similar results, with a fast exhumation from ∼ 30 Ma to ∼ 20 Ma (Fig. 4). Prior to that age interval, the thermal history is not well constrained by the data, as reflected by the high degree of uncertainty in the thermal path, taking into account the 95% credible intervals (Fig. 4A–B). After 20 Ma, the Eaux-Chaudes profile shows a slower cooling towards the surface. In contrast, the Balaitous–Panticosa profile shows a period of stability, although not well constrained by the data, with a last rapid cooling event at 8–9 Ma. The mean geothermal gradient inferred from the temperature offset between samples is of 25 °C/km, with no major variation over time (Fig. 4C–D). ZHe and AHe ages predicted from modeling are coherent within error margins with the observed ages. AFT show worse predicted ages; therefore, the predicted ages are within the error margins of the observed AFT ages only in the case of GPY09 and GPY15 (Fig. 4E–F).
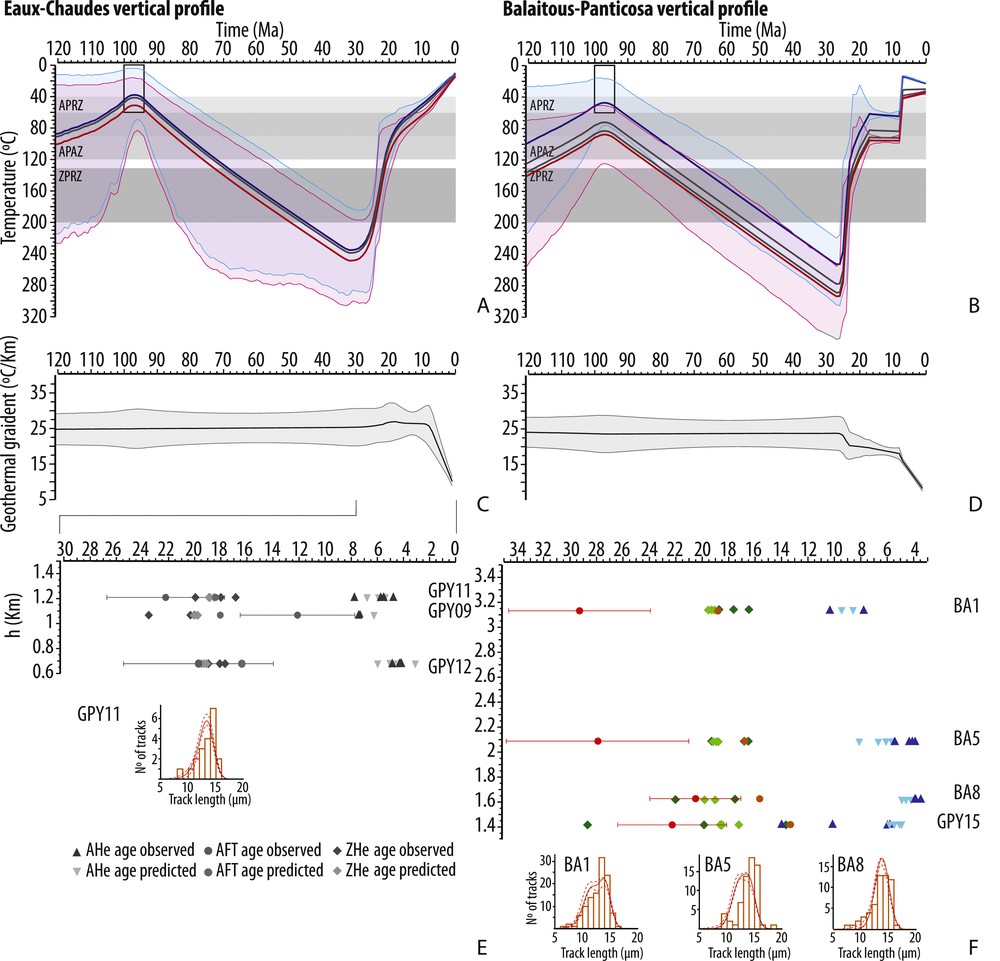
A, B. Modeled thermal history of the Eaux-Chaudes and Balaitous–Panticosa sub-vertical profiles. The red line corresponds to the path of the hottest (lowest elevation) sample (with 95% credible interval range in magenta) and the blue line corresponds to the coolest (highest elevation) sample (with 95% credible interval range in cyan). Intermediate samples are shown in grey. Black boxes correspond to the constraints imposed on the modeling (see text for further information). C, D. Modeled geothermal gradients in red with 95% credible interval range in grey. E, F. Observed and model-predicted apatite (U–Th)/He (AHe) and zircon (U–Th)/He (ZHe) uncorrected ages versus elevation. The predicted track length distribution on sample GY11 is plotted in red with 95% credible intervals in orange in comparison with observed track length data (histograms). Masquer
A, B. Modeled thermal history of the Eaux-Chaudes and Balaitous–Panticosa sub-vertical profiles. The red line corresponds to the path of the hottest (lowest elevation) sample (with 95% credible interval range in magenta) and the blue line corresponds to the coolest (highest ... Lire la suite
Modeling results of the Lakora thrust sheet show that the samples crossed the lower limit of the zircon partial-retention zone (ZPRZ) at 50–42 Ma (bottom and top samples), with a moderate rate of cooling, and passed through the upper limit of the ZPRZ at 25–30Ma (Fig. 5). The pre-Eocene thermal history is not well constrained, due to large uncertainties in the models, reflected by the 95% credible intervals (Fig. 5A). Therefore, the onset age of exhumation cannot be determined from the models, although modeling suggests they were already exhuming by 50–42 Ma. Above the ZPRZ, the thermal path is not constrained, as no AFT and AHe ages were obtained in those samples. As shown on Fig. 5E, the model-predicted ages are consistent, within error margins, with the observed ages.
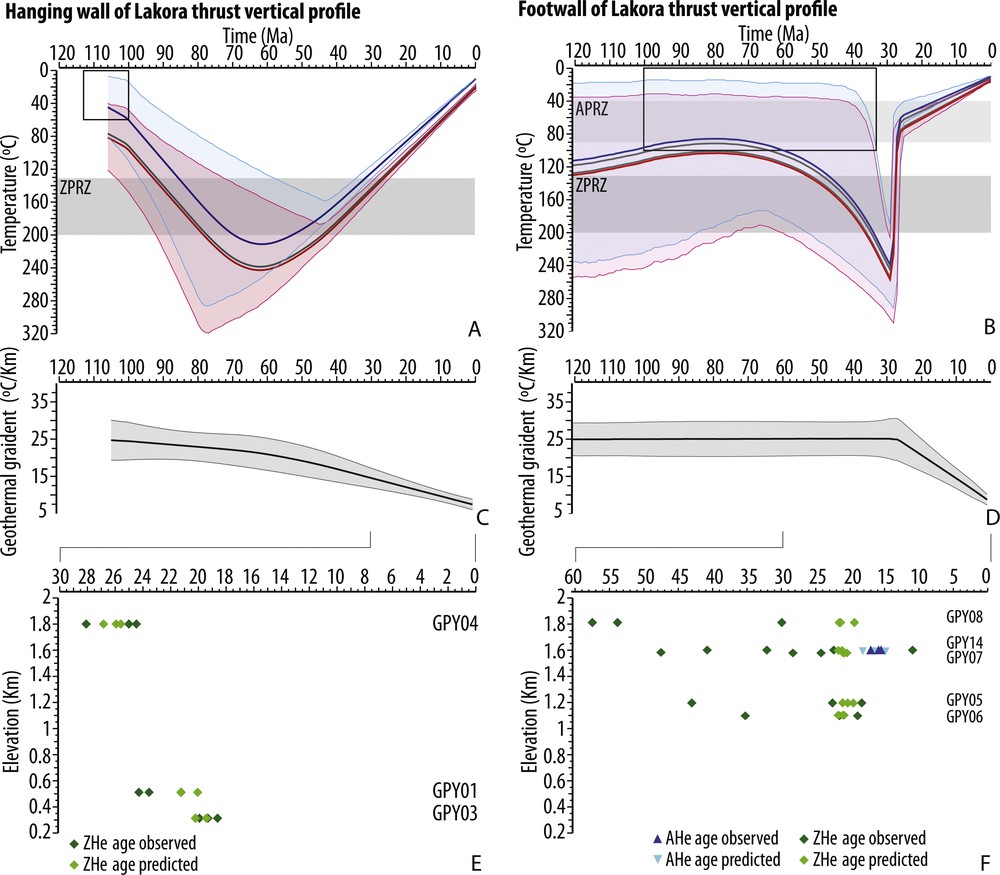
A, B. Modeled thermal history of the vertical profile located in the footwall and hanging wall of the Lakora thrust. The red line corresponds to the path of the hottest (lowest elevation) sample (with 95% credible interval range in magenta). The blue line corresponds to the coolest (highest elevation) sample (with 95% credible interval range in cyan). Intermediate samples are shown in grey. Black boxes correspond to the constraints imposed into the modeling (see the text for further information). C, D. Modeled geothermal gradients in black with 95% credible interval range in grey. E, F. Observed and modeled predicted apatite (U–Th)/He (AHe) and zircon (U–Th)/He (ZHe) uncorrected ages versus elevation. Masquer
A, B. Modeled thermal history of the vertical profile located in the footwall and hanging wall of the Lakora thrust. The red line corresponds to the path of the hottest (lowest elevation) sample (with 95% credible interval range in magenta). The ... Lire la suite
The modeled thermal path of the Lakora thrust's footwall indicates a fast cooling at ∼ 25 Ma, followed by a slower rate final cooling from 25 Ma to the Present. However, this final cooling pattern is not well constrained, since AHe ages were only obtained from one sample (Fig. 5B). Data modeling shows a constant geothermal gradient of 25 °C/km, inferred from the offset between samples. In this case, ZHe ages are badly predicted, as shown on Fig. 5F. Samples from this vertical profile show large intra-sample age dispersion that cannot be predicted by the QTQt software, indicating that the dispersion cannot be explained by crystals eU content or size. The age dispersion could be produced, for example, by complex internal zonation in zircon grains, which are not incorporated in the modeling due to lack of information. The poor predictions showed by the model imply that this last cooling history should be considered with caution.
6 Interpretation and discussion
Single-grain dating performed in this study provide the following five main results:
- • in the Aquitaine Basin, ZHe data indicate no post-depositional reset of ages;
- • the Chaînons Béarnais of the North Pyrenean Zone record a protracted exhumation between 50 and 26 Ma (Eocene to Oligocene);
- • the Lakora thrust sheet was exhumed through the ZHe closure temperature together with its footwall (upper levels of the Gavarnie thrust sheet) at 30–36 Ma (Late Eocene to Oligocene);
- • the granitic massifs of Eaux-Chaudes and Balaitous–Panticosa, and the Upper Paleozoic rocks of the Somport area, all located in the Gavarnie thrust sheet, record a rapid exhumation at 26–20 Ma (Late Oligocene to Aquitanian);
- • the granitic massifs record a final acceleration of exhumation at 8–9 Ma (Late Miocene), which is constrained by the AHe data.
Thermal models further constrain the cooling history of the Lakora thrust sheet, indicating that it was exhuming at least between 50–42 Ma and 30–25 Ma (Early Eocene to Oligocene), and better define the rapid exhumation history of the Eaux-Chaudes and Balaitous–Panticosa granites.
In what follows, we interpret the obtained cooling ages and paths as indicating exhumation primarily linked to the activity of tectonic units, although we understand that climatic events may have played a role to an unknown degree. The samples from the Uppermost Cretaceous rocks of the Aquitaine Basin preserve ZHe detrital ages older than the stratigraphic age, indicating that burial under Cenozoic foreland basin deposits was not enough to reset the ZHe system. This is consistent with the limited degree of diagenesis and cementation observed in these rocks. This pattern changes across the NPFT, where rocks from Paleozoic to Albian age have been buried and heated enough to reset the ZHe system. In the Chaînons Béarnais area, the effect of burial was reinforced by high heat flow during the Middle and Late Cretaceous times, detected by Raman spectroscopy of carbonaceous material that provided paleo-temperatures of 250–300 °C (Clerc et al., 2015). The range of ZHe cooling ages obtained attest for long-lived exhumation in the North Pyrenean Zone initiating at ∼ 50 Ma (including the Lakora thrust hanging wall), consistent with thermal modeling by Vacherat et al. (2014) in the Mauléon basin. To the east of the study area, AFT ages by Meresse (2010) from the Bagnères-de-Bigorre North Pyrenean massif were centered at ca. 41 Ma. Farther east, along the ECORS-Pyrénées transect, most of the AFT data from the North Pyrenean basement rocks indicate exhumation during the Eocene (Fitzgerald et al., 1999; Morris et al., 1998). These data together support an early exhumation of the North Pyrenean Zone during the Early to Middle Eocene. In the study area, we associate the exhumation of the Chaînons Béarnais with the pop-up extrusion of the former North Pyrenean basin by the NPFT to the north and the Lakora thrust and its eastern extensions such as the Eaux-Chaudes thrust (Teixell et al., 2016). Younger cooling ages obtained in the area, especially at deep stratigraphic levels, indicate that thrust-related exhumation proceeded during younger times, caused by continued uplift on the NPFT and probably also by the thick-skinned basement thrusts of the southern Pyrenees, such as the Gavarnie and Guarga thrusts (Fig. 1B). ZHe ages centered on 34–40 Ma in Permian–Triassic rocks indicate exhumation during Middle and Late Eocene to Oligocene, which is consistent with the onset of molasse sedimentation of this age in the Aquitaine basin (Biteau et al., 2006). On the other hand, the southward extent of the Lakora thrust sheet is constrained by the non-completely reset Eocene sample from Pico Matz (GPY08), in agreement with the hanging wall ramp of the Lakora thrust observed in the Lakora klippe, 6 km to the north of Pico Matz.
ZHe ages indicate that the Lakora thrust sheet and its immediate footwall of the Axial Zone cover underwent joint exhumation at 30–36 Ma (Late Eocene to Oligocene), which we must attribute to thrust faulting under the Axial Zone. This cooling age is correlative with Late Eocene to Early Oligocene conglomerate pulses in the Jaca basin, which are dominated by clasts derived from Lower to Middle Eocene turbidites (Puigdefàbregas, 1975; Roigé et al., 2016). We attribute this event to the motion along the Gavarnie thrust (Fig. 1B), because it is the first major south-directed thrust that underlies the Axial Zone in the study area, producing a significant duplication of Paleozoic rocks and creating a marked structural relief. This interpretation is in agreement with the timing of the Gavarnie thrust proposed by Teixell (1996), Jolivet et al. (2007), and Labaume et al. (in review) on the basis of structural relationships and tectonics-sedimentation relationships in the Jaca basin, and differs from Muñoz et al. (2013) attribution of the thrust to the Middle Eocene. It could be argued that the entire profile of the Gavarnie thrust sheet was emplaced below the ZPRZ, and that all the exhumation was driven by the underlying Guarga thrust (Fig. 1B), from the Late Eocene to the Miocene (e.g., samples from Balaitous, Panticosa and Eaux-Chaudes). We consider this unlikely for the study area on the basis of fault slip magnitudes and cross-section balancing in the Jaca basin (e.g., Teixell, 1996), even if the Guarga thrust causes a component of uplift on the Axial Zone. No distinct thermochronology signal can be attributed to the Larra thrust, probably because this thin-skinned branch of the Lakora thrust did not create significant structural relief during its propagation in the Jaca basin fill during the Lutetian–Bartonian.
Following these considerations, we attribute the second ZHe age cluster at 20–26 Ma observed in the western Axial Zone and the fast cooling between 30 and 20 Ma modeled in the granitic massifs to the east to continued uplift of the Axial Zone along the Guarga thrust. This activity of the Guarga thrust was correlated to the main emergence of the South Pyrenean thrust front of the External Sierras, recorded by the Late Oligocene to Aquitanian conglomerates and fluvial sandstones of the Uncastillo Formation (Millán et al., 2000; Puigdefàbregas, 1975; Teixell, 1996).
AHe data show exhumation signals between 6 and 10 Ma in the Eaux-Chaudes and Balaitous–Panticosa plutons, with samples located on both sides of the present drainage divide (Fig. 2). In the Balaitous–Panticosa profile, samples on both sides of the divide can be fit in a single coherent model, indicating that they experienced a similar cooling history. Therefore, the incision during this time could not be caused by the capture of the south-flowing Ebro River, as defended by Fillon and van der Beek (2012).
7 Conclusions
A low-temperature thermochronology study of the western Axial Zone of the Pyrenees and of the adjacent Chaînons Béarnais (North Pyrenean Zone) provides the following constraints on the tectonic and erosional history of this segment of the chain.
The uppermost Cretaceous foreland basin sediments of the Aquitaine basin have not been buried enough for post-depositional reset of the ZHe thermochronology system and preserve Permian to Jurassic detrital signals.
Within the Chaînons Béarnais, the ZHe system was reset and record continued pop-up-like exhumation of the North Pyrenean Zone between the North Pyrenean Frontal Thrust and the major south-directed thrusts of Lakora and others further south (Gavarnie and Guarga) from 50 to 26 Ma (Eocene to Oligocene).
The leading edge of the Lakora thrust sheet, which was reported to bring the North Pyrenean Zone on top of the Axial Zone during Late Cretaceous to Middle Eocene times, shows a cooling path at least from Early Eocene to Oligocene times. The Lakora thrust sheet together with its immediate footwall forming the post-Variscan cover of the Axial Zone were exhumed through the ZHe closure temperature at 36–30 Ma (Late Eocene to Oligocene) along the underlying Gavarnie thrust. Hence, the cooling path of the Lakora thrust sheet is the result of the activity of the Lakora thrust itself and of the underlying Gavarnie (and possibly Guarga) thrusts.
Paleozoic sediments of the westernmost Axial Zone often yield scattered ZHe thermochronology results indicating partial reset. The granite samples from the Eaux-Chaudes and Balaitous–Panticosa plutons provide a good ZHe and AFTL Late Paleogene to Miocene signal, clearly reflected in models as fast cooling between 30 and 20 Ma (Late Oligocene to Aquitanian). We attribute this cooling to thrusting on the Guarga thrust, ultimately uplifting the older Gavarnie thrust sheet lying above.
AHe results from the Eaux-Chaudes and Balaitous–Panticosa plutons cluster at 9–8 Ma (Late Miocene) attesting for post-orogenic cooling that was detected in previous studies of the southern foreland basin.
Acknowledgements
This work was supported by projects CGL2010-15416 and CGL2014-54180-P (MINECO, Spain) and PYRAMID (ANR, France), and by Géosciences Montpellier. We acknowledge the constructive reviews by Luis Barbero, Camille Clerc and the co-editor Yves Lagabrielle, which helped to improve the manuscript. Andreu Badia is thanked for field assistance during sampling.