1 Introduction
The Sanandaj–Sirjan Zone (SSZ) is a part of the Zagros Orogenic Belt (ZOB), which is a member of the Alpine–Himalayan Orogenic Belt. The Zagros Belt comprises several NW–SE-trending zones that were formed as a consequence of the opening and closure of the Neo-Tethys Ocean beneath the Iranian Plate (Agard et al., 2005; Alavi, 1980, 1994; Berberian and Berberian, 1981; Berberian and King, 1981; Sengor, 1990). The ZOB of Iran consists of three parallel tectonic subdivisions trending NW to SE (Alavi, 1994):
- • the Zagros Simply Folded Belt;
- • the Sanandaj–Sirjan Zone (SSZ);
- • the Urumieh–Dokhtar Magmatic assemblage (UDMA) (Fig. SM 1).
The reported ages for the granitoid plutons in the SSZ range from Neoproterozoic (Hassanzadeh et al., 2008) to Tertiary (Mahmoudi et al., 2011; Sepahi et al., 2014). The Paleozoic granitoid rocks are less distributed in the Iranian Plate and are related to the extensional intracontinental regime that accompanied the rifting of the Arabian Plate and the opening of the Neo-Tethys (e.g., Abdulzahra et al., 2016; Alirezaei and Hassanzadeh, 2012; Bea et al., 2011; Shafaii Mogadam et al., 2015; Shakerardakani et al., 2015). The majority of the granitoid rocks belong to the Middle to Late Jurassic and are linked to the subduction of the Neo-Tethys Ocean beneath the Iranian Plate (e.g., Ahmadi Khalaji et al., 2007; Azizi et al., 2011, 2015; Chiu et al., 2013; Esna-Ashari et al., 2012; Fazlnia et al., 2009; Hassanzadeh and Wernicke, 2016; Mahmoudi et al., 2011; Omrani et al., 2008; Shahbazi et al., 2010). However, little is known about the Cretaceous granitoid rocks in the SSZ as compared with the Jurassic granitoids. Zircon U–Pb age of the Hasan Salary I-type granites is 108.8 ± 0.3 Ma (Mahmoudi et al., 2011) and linked to the subduction-related magmatism of the Neo-Tethys Ocean beneath the Iranian Plate. Mazhari et al. (2011) dated the Naqadeh complex and reported a crystallization age of 96 ± 2.3 Ma. Cretaceous K–Ar and Rb–Sr ages were reported in the SSZ (e.g., Baharifar et al., 2004; Masoudi et al., 2002). These K–Ar and Rb–Sr isotope data show resetting of variable degrees by secondary processes (Mahmoudi et al., 2011) and are useful to estimate the tectonic and metamorphic events (Allègre, 2008; Asmerom et al., 1991; Evans, 1995) that occurred in the SSZ rather than those of crystallization.
In this study, a new radiometric age as well as chemical and Sr–Nd isotopic data are reported from the Sirstan granitoid rocks (SG) in the Shalair Valley within the SSZ in northeastern Iraq (the details of the analytical techniques are supplied as supplementary material). The present study throws light on the existence of a Cretaceous igneous activity that was previously considered to be a magmatic gap in the SSZ, as reported by Chiu et al. (2013).
2 Geological setting and sampling
The Sirstan granitoid body is located in the Shalair Valley area within the so-called Iraqi Suture Zone in northeastern Iraq near the Iraqi–Iranian border. This zone is considered as part of the Zagros Orogenic Belt (ZOB) that extends ca. 2000 km from Southeast Turkey through northeastern Iraq–northwestern Iran to northern Oman (Fig. SM 1; Alavi, 1994). This zone is linked to the Neo-Tethys Ocean and thrusted over the Arabian Plate in the Late Cretaceous obduction followed by collision during Miocene–Pliocene times (Jassim and Goff, 2006). The Zagros Suture Zone in northeastern Iraq is divided into three units that trend parallel to the main trend of the Zagros Belt, including the Qulqula–Khwakurk, Penjween–Walash and Shalair units (Buday and Jassim, 1987; Jassim and Goff, 2006) (Fig. 1a). The Shalair unit is located within the Shalair Valley area and is considered as part of the Sanandaj–Sirjan Zone. Structurally, the Shalair Valley is an eroded east–west-trending asymmetrical anticline whose axis gently plunges toward the east (Smirnov and Nelidov, 1962). It extends approximately over 30 km with a width of more than 20 km. The value of the dip ranges from 30° to 70° for the southern limb and from 20° to 40° for the northern limb (Smirnov and Nelidov, 1962). The core of the Shalair anticline is composed of metasedimentary sequences (Qandile Rock Series), whereas the limbs comprise the Katar Rash Volcanic Unit (also known as the Walash “Volcanic” Rock Series; Al-Shible and Kettaneh, 1972; Smirnov and Nelidov, 1962; Fig. 1b) that ranges in composition from basic to acidic igneous rocks (Al-Shible and Kettaneh, 1972; Buday and Jassim, 1987; Jassim and Goff, 2006). The Qandile Rock Series covered the majority of the Shalair Valley area and comprise 2000 m (Smirnov and Nelidov, 1962) of phyllite, schist, slate and greywacke with minor felsic volcanic and some lenticular limestone (Buday and Jassim, 1987; Jassim and Goff, 2006; Smirnov and Nelidov, 1962). The contact with the overlying Katar Rash Series is unclear, maybe gradational contact or low-angle unconformity (Jassim and Goff, 2006). Based on microfacies analyses, the estimated age of the Qandile Rock Series is Aptian–Cenomanian. However, a geochronological study is required to confirm this age. The Qandile Rock Series are reported to be formed in a fore-arc basin in front of the subduction zone (Jassim and Goff, 2006). The Katar Rash Series (also known as the Walash “Volcanic” Rock Series; Al-Shible and Kettaneh, 1972; Smirnov and Nelidov, 1962) are exposed along the limbs of the Shalair anticline and comprise 1000 m of calc-alkaline series of basaltic andesite, andesite, dacite and rhyolite (Jassim and Goff, 2006). This unit has been dated using U–Pb zircon age and yielded a crystallization age of ca. 108 ± 2.9 Ma (Ali et al., 2016).
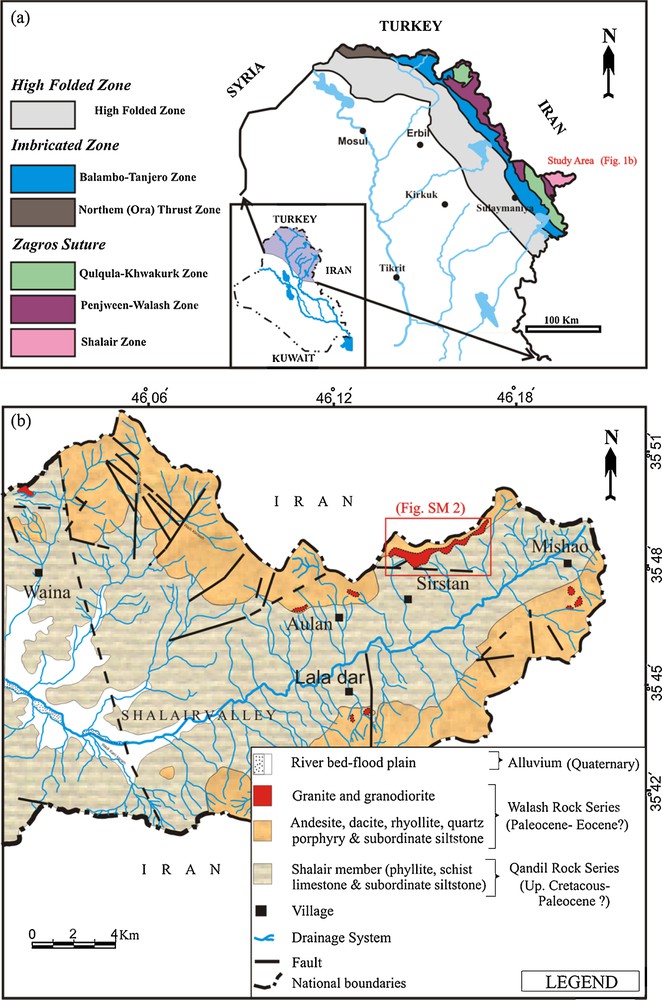
a: tectonic zones of the Iraqi Zagros Suture Zone (Jassim and Goff, 2006), showing the distribution of igneous and metamorphic rocks; b: geological map of the Shalair Valley area from Al-Shible and Kettaneh (1972), modified.
Several granitoid bodies with variable dimensions crop out within the Shalair anticline; these are: Aulan, Sirstan, Laladar and Mishao which are exposed at the limbs within the Katar Rash Volcanic Unit and one body, the Damamna is exposed in the core within Qandile Rock Series (Abdulzahra et al., 2016; Al-Hafdh and Qasim, 1992; Al-Rubaie, 1976; Al-Shible and Kettaneh, 1972; De Villiers, 1957; Smirnov and Nelidov, 1962).
Various ages have been reported concerning the igneous activities in the Shalair Valley. Al-Shible and Kettaneh (1972) and Smirnov and Nelidov (1962) suggested that the igneous activity was during the Tertiary. Buday and Jassim (1987) and Jassim and Goff (2006) estimated that the Shalair Valley igneous activity was in the Late Cretaceous. Al-Hafdh and Qasim (1992) proposed Late Cretaceous to late Miocene. However, these estimated ages are based on field observation and correlation with the Upper Cretaceous igneous activities in the SSZ. For more details on the geology of the Shalair Valley area (see Jassim and Goff, 2006).
Cretaceous magmatic activities are well developed in the northwestern part of the SSZ compared with the southeastern part (Azizi and Moinevaziri, 2009; Mohajjel et al., 2003). In the northwestern part of the SSZ, the country rocks are composed mainly of shale, sandstone and calcareous rocks, which are intercalated, by basalt, andesitic basalt and andesite. These rocks show calc-alkaline magmatic affinity and are linked with a continental arc setting during the subduction of the Neo-Tethys Ocean beneath SSZ (Azizi and Jahanjiri, 2008; Azizi and Moinevaziri, 2009). Azizi and Moinevaziri (2009) suggested that the metasomatized mantle was responsible for introducing calc-alkaline magmatism during the subduction of Neo-Tethys Ocean. These magmatic activities were developed to form the magmatic arc system in the northwestern part of the SSZ.
In this study, we focus on the Sirstan granitoid (SG). The present new geochemical and geochronological data on the Sirstan rocks add new information on events in continuation with the events that occurred in the Sanandaj–Sirjan Zone and cover the missing age (Middle Cretaceous). Therefore, we employed the geochemical data and age relationship of the Siristan rocks, which seem to be important in the study of magmatic and tectonic history in Zagros Belt. The SG body is located to the north of the Sirstan village close to the Iranian border (Fig. SM 2). It is the largest intrusive body in the Valley and extends for about 5 km. This body is characterized by a very steep slope with rugged topography and is surrounded by volcanic rocks of Katar Rash from the north and phyllite rocks of Qandil Rock Series from the south (Fig. SM 2, SM3a). The contact relation between the SG and the surrounding rocks is not so clear (Al-Shible and Kettaneh, 1972; De Villiers, 1957; Smirnov and Nelidov, 1962). Generally, the rocks are gray to greenish gray in color, massive, coarse grained, fractured (Fig. SM 3a, b) and are cut by numerous quartz and epidote veins. Numerous mafic enclaves were noted within the coarse granitoid rocks throughout the Sirstan granitoid body. These enclaves show variable shapes and some are spherical, elliptical with sharp contact with the hosted granitoids. Their lengths are from a few centimeters to more than 15 cm; they are apparently finer grained than the surrounding or enclosing rocks (Fig. SM 3c, d).
3 Results
3.1 Petrography
The rock samples of the Sirstan pluton are composed mainly of plagioclase (45%), quartz (38%) and hornblende with minor amount of pyroxene (9%). Zircon, sphene and magnetite are the accessory minerals identified making approximately 7%, with sericite, epidote and chlorite as alteration products. The SG samples show an equigranular texture. According to the extinction angle measurement, the average plagioclase composition is from albite to oligoclase. They are subhedral and show variable size (0.3–3.0 mm) with an average of approximately 1.4 mm. Some of the plagioclase grains display deformation twins and fracturing (Fig. 2a). The quartz grains are anhedral with an average size of approximately 1.2 mm and a few of them show undulose extinction (Fig. 2b). The mafic minerals are dominated by hornblende, with minor pyroxene (augite), which are observed in all the studied samples, and make about 5 to 25 percent by volume. The grains are generally fresh and have well developed cleavage (Fig. 2c). They are subhedral to anhedral, greenish brown to faint brown in color and are peleocroic. In some thin sections, hornblende contains inclusions of plagioclase, quartz and opaque minerals (Fig. 2d, e). Some grains are altered due to an urilitization process along the grain boundaries and cracks. Opaque minerals are mostly magnetite, which occurs as single euhedral grains or as disseminated very fine-grained aggregates associated with highly deformed and altered regions. The enclave samples exhibit more or less similar mineralogical and textural features to the enclosing host granitoids, but are finer grained than the host and constitute more mafic minerals that are mainly hornblende along with minor amounts of biotite. These mafic minerals are surrounded by quartz and plagioclase forming a microgranular texture (Fig. 2f).
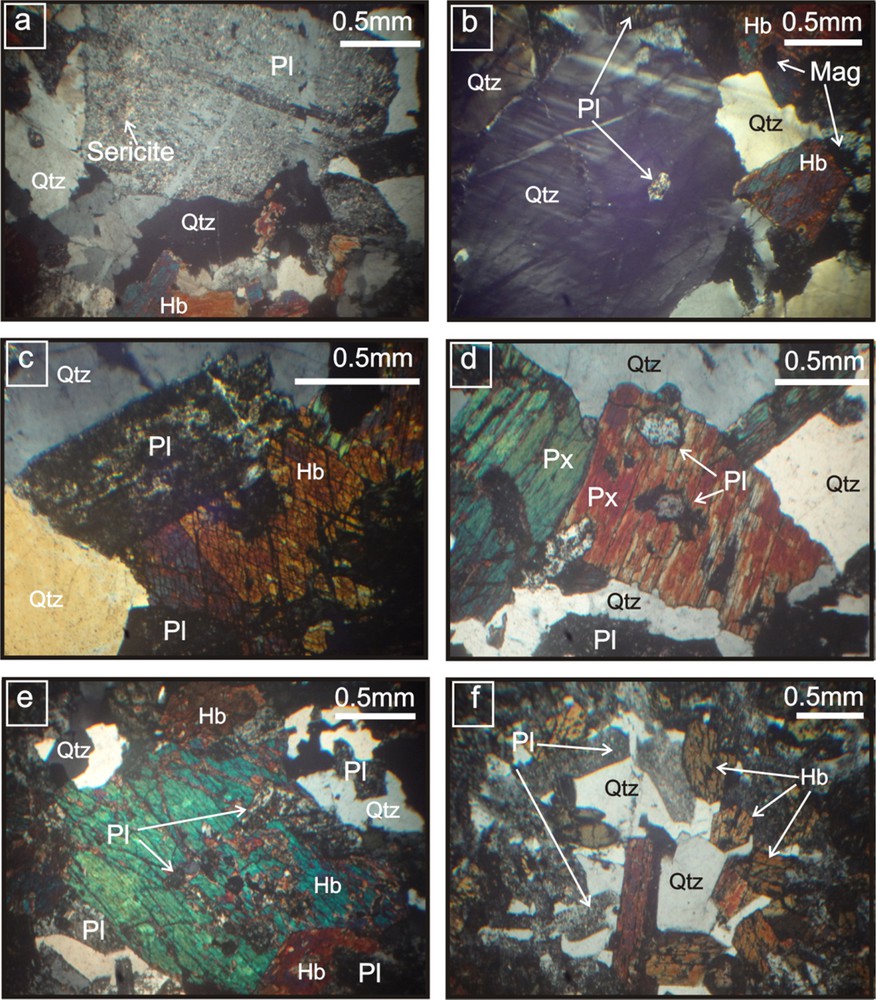
Photomicrographs of the SG. a: deformation twins lamellae in plagioclase; b: undulose extinction in quartz; c: fresh hornblende with altered plagioclase; d, e: plagioclase imbedded in pyroxene; f: mineralogy of enclave samples, showing similar mineral composition with the host SG rocks. Symbols: Pl = plagiocalase; Qtz = quartz; Mag = magnetite; Hb = hornblende and Px = pyroxene.
3.2 Whole-rock geochemistry
The analytical results of the major oxides in weight percent and the trace elements including REEs in ppm are listed in Table SM 1. The Sirstan granitoid and the enclave sample have moderate contents of SiO2 (57.0–61.4 wt.%), high Al2O3 (15.3–16.7 wt.%) and (Na2O + K2O) (3.85–5.47 wt%). These rocks contain 0.62–0.74 wt.% TiO2, 6.6–8.8 wt.% Fe2O3, 2.5–4.0 wt.% MgO and 2.7–7.1 wt.% CaO contents. The enclave sample (XeS) shows relatively less CaO, and high Fe2O3 and Na2O contents than the enclosing host SG granitoids.
The SG rock samples show a good fractionation trend of major and trace elements with SiO2 when plotted on the variation diagrams (Fig. SM 4).
In the alkali versus silica diagram (Middlemost, 1985), all samples of the SG and the enclave sample (XeS) are plotted in the diorite field with few SG samples along the border between diorite and granodiorite fields (Fig. SM 5a) and are calc-alkaline on the AFM diagram (Fig. SM 5b: Irvine and Baragar, 1971). On Al2O3/(CaO + Na2O + K2O) versus Al2O3/(Na2O + K2O) of Shand (1943) (Fig. SM 5c), the SG samples are defined as metaluminous with ASI values less than one, whereas the enclave sample has peraluminous affinity.
The chondrite-normalized REE patterns (normalization after Sun and McDonough, 1989) for the SG rocks are homogeneous (Fig. 3a) with fractionation REE (10 to 40 times chondrite) and slight LREE enrichment (La/Yb)N = 1.9–2.5 (Table SM 1). The patterns show negative Eu anomalies (Eu/Eu* = 0.83–0.97) that indicate plagioclase fractionation during the melting and crystallization stages. The REE pattern for the enclave sample more or less runs parallel to the host rock patterns, but shows relative enrichment with respect to the host granitoids with lower (La/Yb)N (= 1.3) and more pronounced negative Eu anomaly (Eu/Eu* = 0.54). On the primitive mantle–normalized multi-component element diagram (normalization after Sun and McDonough, 1989) (Fig. 3b), the SG samples show a good consistency in the distribution of the elements with high amounts of K, Rb, and Pb, which are probably due to alteration, and distinct Nb, Ta and Ti depletions, which are characteristic geochemical indicators of subduction-related calc-alkaline magma (Pearce et al., 1984). The enclave sample exhibits a geochemical similarity with the host rocks, but is more depleted in Nb, Ta, and Ti, and slightly more enriched in HREE.
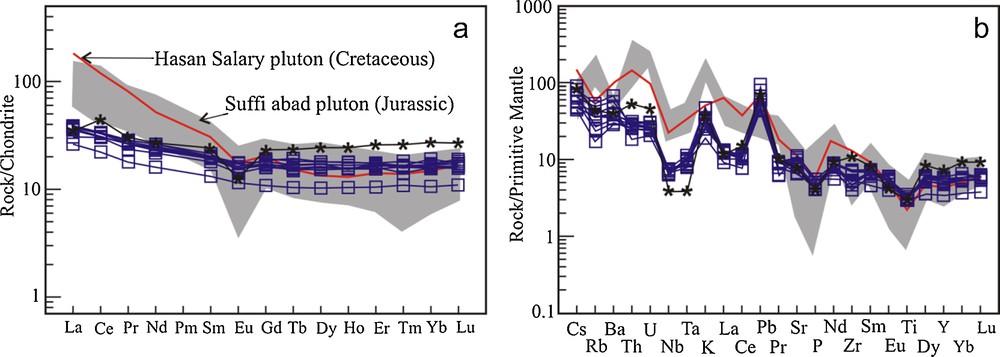
a and b: REE patterns in the SG samples normalized by chondrite and primitive mantle, respectively (normalization after Sun and McDonough, 1989). See text for details. Data for the Suffi abad pluton from Azizi et al. (2011) and the Hasan Salary pluton from Mahmoudi et al. (2011) are shown for comparison. The star symbol indicates an enclave sample.
On the 10,000 × Ga/Al-versus-Nb diagram (Fig. SM 6a), the SG samples and enclave samples are plotted in the M-S-I-type fields. The average values of 10,000 × Ga/Al ratios for the studied rocks of the SG and enclave (Table SM 1) are similar to the average values of I-type granitoids proposed by Whalen et al. (1987).
3.3 U–Pb zircon geochronology
Two samples (SG1 and SG2) were selected for zircon U–Pb age determination. The zircon grains are elongated, with lengths up to 250 μm. CL images (Fig. SM 7a, b) show that some zircon grains have zoning. And the zircon grains have microfractures that have affected grain size and shape. This could be due to deformation processes or to crushing of the rock sample during preparation.
The U–Pb analytical results of the selected zircon grains are listed in Table SM 2. According to the estimates from 204Pb intensity measured by LA–ICP–MS analysis, these zircon grains have low common lead contents (about 2% for SG1 and SG2).
The values of the Th/U ratio of the zircon grains for SG1 and SG2 are 0.75 and 0.59, respectively, from which a magmatic origin can be inferred (Chen et al., 2007; Hartmann and Santos, 2004; Hoskin and Schaltegger, 2003; Rubatto, 2002). Both rims and cores for the zircon grains have the same age (Table SM 2). The U–Pb ages of the SG samples are calculated using ISOPLOT v. 4.15 software (Ludwig, 2012) and are 109.3 ± 1.3 Ma, with a mean square weighted deviate (MSWD) value of 1.4 for sample SG1 and 114.9 ± 4.9 Ma (MSWD = 9.5) for sample SG2 (Fig. 4a, b). Because of approximately similar results of the two-concordia ages, a weighted average age of 109.7 ± 1.3 Ma is taken as the crystallization age of the SG, which is in correspondence with the Middle Cretaceous period (i.e. Albian).
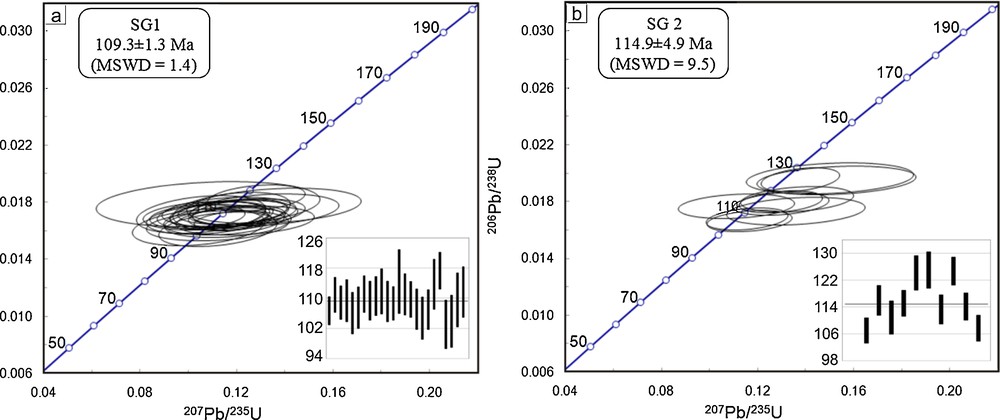
U–Pb concordia ages for the SG. a: SG1 and b: SG2. Data-point error ellipses are 2σ.
3.4 Rb–Sr whole-rock isochron
Eight out of nine samples were used to calculate Rb–Sr isochron. The excluded sample (S17) is slightly altered and it falls slightly off the isochron. The whole-rock Rb–Sr isochron system for the SG rocks shows a regular linear distribution of points (Fig. 5) and gives an age of 52.4 ± 9.4 Ma with a MSWD of 1.7, which is younger than the zircon U–Pb age (110 Ma). This is probably attributed to an incomplete reset of the whole-rock Rb–Sr isotope system during alteration and/or metamorphism processes (Allègre, 2008; Asmerom et al., 1991; Evans, 1995). This Rb–Sr data can be used to discuss the tectonic and metamorphic events that occurred in the area within Northeast Iraq and Northwest Iran during Paleogene period (i.e. the Eocene). It is possibly related to evidence of final closure of the Neo-Tethys (Ghasemi and Talbot, 2006; Jassim and Goff, 2006; Kazmin, 1991; Mazhari et al., 2009; Sharland et al., 2001; Vergès et al., 2011).
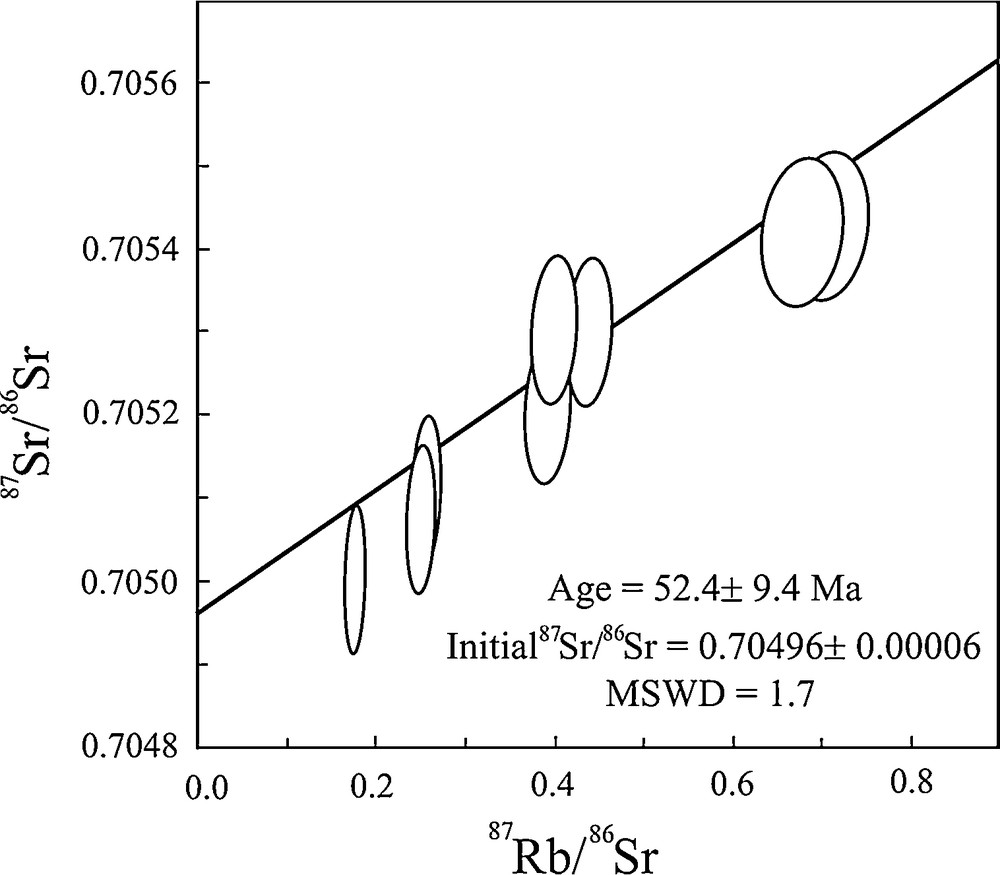
Rb–Sr whole-rock isochron for 8 SG samples, shows an age of 52.4 ± 9.4 Ma. Data-point error ellipses are 2σ.
3.5 Sr and Nd isotope ratios
The results of our isotope analyses for Rb–Sr and Sm-Nd are listed in Table SM 3.
The initial values of both Sr and Nd isotope ratios (87Sr/86Sr, 143Nd/144Nd) for the SG and the enclave rocks were calculated based on the U–Pb zircon age of 110 Ma. The values of 143Nd/144Nd (i) vary between 0.51250 and 0.51263, with positive ɛNd (110 Ma) values ranging from +0.1 to +2.7, indicating mantle origin for both SG and enclave rocks. The initial 87Sr/86Sr values range from 0.7044 to 0.7057 for SG rocks which is the characteristics of I-type granites (Chappell and White, 1974, 1992). Armstrong et al. (1977) and Chappell and White (1974) suggested a critical boundary of 0.7060 for 87Sr/86Sr value with I-type granites having initial 87Sr/86Sr ratios between 0.7040 and 0.7060. Pitcher (1979) suggested the characteristic of initial 87Sr/86Sr values less or more than 0.7060 for the I- and S-type granites, respectively.
4 Discussion
4.1 Magma source and fractional crystallization
As previously stated, the rocks of the Sirstan granitoids (SG) and the enclosing enclave have the characteristics of I-type granite. In the classification scheme, they have chemical signature of calc-alkaline magma in subduction zone environment. These rocks are part of magmatic arc as appeared in tectonic discrimination diagram of Pearce et al. (1984) (Fig. SM 6b), and are classified as orogenic granite as appeared in K/Rb vs. Rb/Sr diagram (Fig. SM 8a; Abdel-Rahman and El-Kibbi, 2001). The vast majority of SG rocks are plotted within the active continental margin (ACM) field, whereas the enclave sample shows oceanic arc affinity in the Ta/Yb–Th/Yb and Yb–Th/Ta diagrams (Fig. SM 8c, d: Gorton and Schandl, 2000). The enclave sample has Th/Ta ratio values reaching up to 27.4. In addition, the SG rock samples follow the assigned fractional crystallization trend (Fig. SM 8c).
The whole-rock geochemical characteristics, Sr–Nd isotopic data with positive ɛNd (110 Ma) values (+0.1 to +2.7), and their clumped distribution within the mantle array in 87Sr/86Sr–ɛNd (110 Ma) diagram (Fig. SM 8b) indicate that the magma source for the SG rocks and the enclave lies in the mantle origin. Furthermore, the low values of Rb/Sr coupled with high Sm/Nd ratios (Table SM 1) let us infer that the parental magma of these rocks was generated by partial melting of a depleted mantle that underwent fractional crystallization when ascended into the continental crust. The geochemical aspects, including the relatively linear trend of the major and trace elements with SiO2 (Fig. SM 4); the similarity of the REE patterns (Fig. 3) and the incompatible elements ratios (Fig. SM 8c) all support the role of the fractional crystallization in the evolution of the source magma of the SG rocks. Furthermore, the relationship of various trace elements and their ratios also support the effect of fractional crystallization in the evolution of the studied rocks. For example, the plots of Rb/Ba vs. Ti/Y ratios (Fig. SM 8e) show a random distribution and scattering of the plotted points. If the parental magma was contaminated or mixed with continental crustal components, then the trend of these rocks should be more or less linear and show increasing, i.e. these should be some sort of increase in the values of Rb/Ba and Ti/Y ratios. Also, on the plot giving the initial 143Nd/144Nd ratios vs. SiO2 (Fig. SM 8f), the 143Nd/144Nd trend, which is almost a straight line, shows no considerable increase when SiO2 increases. All these aspects in addition to the initial 87Sr/86Sr and ɛNd (110 Ma) values indicate that neither contamination nor mixing with crustal components had any influence in the evolution of the magma, and that these rocks were most probably derived from the mantle and evolved through fractional crystallization processes.
Many propositions have been put to explain the genesis of the enclaves that occur with the granitoid rocks including enclaves of wall rock fragments magma mingling, restite and chilled margins (Barbarin, 1988; Barbarin and Didier, 1992; Chappell et al., 1987; Chen et al., 1990; Elburg, 1996; Kocak, 2006; Silva et al., 2000).
As previously noted, the enclave in the study area shows more or less similarities in their chemical compositions with the SG rocks in terms of the major and trace elements as well as the Sr–Nd isotopic ratios, which all indicate the cogenetic origin for both. However, the enclave sample is more mafic, containing higher contents of hornblende and biotite, with lesser alkalis; it is finer grained than the hosted SG rocks. It is peraluminous and shows oceanic arc affinity (Fig. SM 8c, d) and is relatively enriched in REE relative to the hosted granitoids. These lines of evidences more likely indicate that the enclave represents the earlier stage of evolution of the source magma than the SG rocks. In other words, the less evolved portion of the magma underwent the processes of the replenishment plus fractional crystallization (RFC) during the partial melting that had extracted the incompatible elements. The processes of RFC could be responsible for the observed trends of the REE and multi-elements (Fig. 3a, b) (e.g., Smith and Gray, 2011). However, more detailed studies are needed concerning the age of the enclaves in these rocks in order to provide reliable insights into their petrogenesis.
4.2 Middle Cretaceous igneous activity within the Zagros Belt
As previously discussed, the zircon U–Pb age of the SG rocks is 110 Ma, which indicates the existence of the igneous activity in SSZ during the Middle Cretaceous (i.e. Albian). The whole-rock Rb–Sr isochron for the SG rock samples yield rough estimated age of 52.4 ± 9.4 Ma, which is younger than the zircon U–Pb age. Although the estimated Rb–Sr isochron age (52.4 Ma) is not used here for magmatic geochronology, but probably gives reliable information about the timing of the tectonic and metamorphic events that occurred during the Paleogene period (i.e. Eocene) in northeastern Iraq and northwestern Iran within the Zagros Belt.
Chiu et al. (2013) reported a summary of the U–Pb zircon ages to constrain the magmatic evolution related to the Neo-Tethys subduction and the Zagros Orogeny during Mesozoic and Cenozoic times. They concluded that the highest magmatic activities in the SSZ had occurred during the Jurassic period (176–144 Ma). However, little is known about the magmatic activities during Early and Middle Cretaceous times (Fig. 6). Agard et al. (2011) concluded that the Middle to Late Cretaceous period (115–85 Ma) represents a specific time of perturbation of subduction marked by blueschist exhumation along the northern part of the Neo-Tethys subduction zone and the obduction processes in the southern part of the Neo-Tethys. Furthermore, this time is marked by rifting in the back-arc setting followed by closure during Late Cretaceous times with the development a supra subduction zone ophiolite (e.g., Agard et al., 2011; Ali, 2012; Ali et al., 2012; Shafaii Mogadam et al., 2009, 2014). Agard et al. (2005) suggested that the Middle to Late Cretaceous time recorded a change in the rates of convergence and speed of the Neo-Tethys subduction beneath SSZ. It is likely that the SG rocks were formed during the time of the perturbation of the subduction processes during the Middle Cretaceous time.
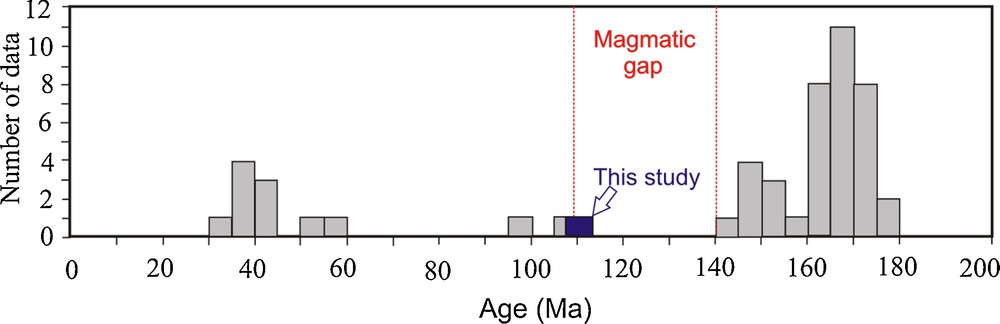
Histogram of the age results of igneous rocks in the SSZ (after Chiu et al., 2013), showing the magmatic gap during the Cretaceous period.
The geochemical and isotopic characteristics of the SG rocks indicate that these rocks came from partial melting of depleted mantle-derived materials above the subduction zone without significant contamination by continental crust. Furthermore, these features imply magmatic arc intrusion related to an active continental margin as the origin of the SG rocks. Based on our study, the zircon U–Pb age (110 Ma), which corresponds to the Middle Cretaceous adds new information about the magmatic activity that occurred in the Middle Cretaceous in the northern Arabian peninsula (northeastern Iraq) and northwestern Iran within the Zagros Belt.
5 Conclusions
The Sirstan granitoid body crops out in the northern Sanandaj–Sirjan Zone in northeastern Iraq. Zircon U–Pb dating shows that these rocks crystallized at 110 Ma. Chemical compositions and initial isotope ratios show that they are calc-alkaline granitoids that were generated in an active continental margin regime. Although the distribution of the Mesozoic granitoid bodies in the SSZ has shown that the main granitic bodies were crystallized during the period from 140 to 160 Ma, the SG granitoid with typical calc-alkaline magma in the active margin confirms the extending of granitoid bodies from the Middle Jurassic to the Middle Cretaceous in the northern SSZ. This clarifies a long period of Neo-Tethys subduction beneath the Iran. The whole-rock Rb–Sr isochron from fresh samples with 52 Ma probably suggests the heating of these rocks due to the collision of the Arabian and Iranian plates in the Cenozoic.
Acknowledgements
This research was financially supported by the Ministry of Higher Education and Scientific Research in Iraq and by JSPS KAKENHI grants No. 25303029 in Japan. I. Kadhim Abdulzahra would like to thank Nagoya University in Japan for supporting him as a Special Research Student during the period from November 2014 to October 2015 and the Iraq Geological Survey for support during the field trips. We also thank Ms. Masumi Nozaki (Nagoya University Museum) for SEM analysis, Prof. Makoto Takeuchi (Nagoya University) for zircon separation and Mr. Yoshikazu Kouchi (Toyama University, Japan) for advice about U–Pb age calculation.
We are grateful to Marc Chaussidon, Associate Editor of the journal, and the reviewers Philippe Boulvais and Ahmad Jahangiri for their constructive comments.