1 Introduction
The intense urban and industrial development in coastal zones of emerging countries has increased the inputs of anthropogenic trace elements in rivers and estuaries and resulted in the loss of important intertidal ecosystems as mangrove wetlands (Bayen, 2012; FAO, 2003; Lewis et al., 2011; Marchand et al., 2016).
In tropical intertidal zones, trace elements tend to accumulate in the sediments (e.g., mud flat and mangrove sediments) due to adsorption onto solid phase surfaces (e.g., clay minerals and oxyhydroxide fine-grained particles), complexation by organic molecules, (co-) precipitation with sulfides or formation of their own sulfidic minerals (Huerta-Diaz and Morse, 1990; Machado and Lacerda, 2004; Morse and Luther, 1999). In the sedimentary environment, these elements may be remobilized between the different sediment phases, from the oxide form to the organic and sulfide forms, by diagenetic processes, frequently involving bioturbation and rhizosphere processes (Machado and Lacerda, 2004). Thus, coastal sediments play an important role as biogeochemical barriers for potentially toxic anthropogenic contaminants transported across the interface between terrestrial and marine environments (Machado and Lacerda, 2004; Marchand et al., 2016). However, depending on the physicochemical conditions in the sediment–water interface (pH, Eh, salinity, etc.) toxic trace elements can become bioavailable, implying potential risks to human and biota health (Du Laing et al., 2009; Marchand et al., 2016).
Since intertidal zones play important roles in supporting food webs and supplying food for inhabitants of coastlines, our comprehension of the geochemical cycling of metals and man-induced disturbances of this cycling (e.g., coastal resources over-exploitation, pollution, and degradation of mangrove wetlands) is imperative to better manage these zones. It is estimated that mangroves are disappearing worldwide at the rate of 1 to 2% per year, due to their conversion into farming and urbanized areas (Valiela et al., 2001). The degradation of mangroves may have negative consequences such as the releasing of contaminants into the coastal systems, including toxic trace metals, which may be incorporated in the marine and terrestrial food webs.
The Sepetiba Bay is a microtidal estuarine lagoon, located 60 km west of Rio de Janeiro city (Fig. 1), which has been largely urbanized and industrialized since the 1950s. It represents a case of coastal system under severe anthropogenic pressure. This bay is in the geo-economic center of Brazil, hosting a large population of the metropolitan region of Rio de Janeiro city (estimate of 1.4 million inhabitants), an expressive industrial park, including the largest steel industry complex of Latin America, and important harbor activity, with three ports in operation. The original hydrological features of the bay have been modified since the 1950s by diversion of waters of the main river (River Guandu) to address the regional demand for water (Rodrigues et al., 2012). Consequently, the sediment load (about 28%) and fresh water inputs into the lagoon system have increased significantly (Marques et al., 2006; Molisani et al., 2004, 2006; Smoak and Patchineelam, 1999). This bay has been also heavily impacted by metal contamination from mining tailings from a Zn electroplating plant. Zinc concentrations in sediments may exceed 2000 μg·g−1 (Pellegatti et al., 2001; Wasserman et al., 2001) along the bay area, while concentrations about 396 μg·g−1 for Cd (Molisani et al., 2004 and references therein), 360 μg·g−1 for As (Magalhães and Pfeiffer, 1995), and 668 μg·g−1 for Pb (Molisani et al., 2004 and references therein) have been reported for sediments close to the major industrial source. In 2012, the mining tailings were removed from the bay. Recently, the levels of Zn in sediments have been addressed (Araújo et al., 2017a, b), but no published study has reported the current contamination levels of highly toxic elements as As, Cd, Pb, Cr, Cu, and Ni.
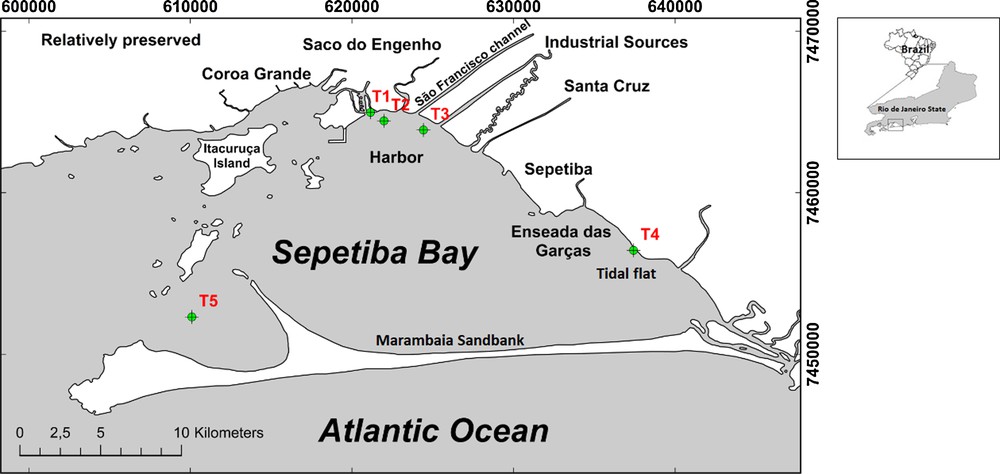
Sampling site in the Sepetiba Bay. T1 core: Saco do Engenho; T2 core: Saco do Engenho mouth; T3 core: São Francisco Channel; T4 core: Enseada das Garças; T5: Marambaia.
Over the last decade, the Sepetiba Bay has undergone economic growth with expansion of its industrial park and harbor activities, which likely caused the emergence of new potential diffuse sources of pollution. This new scenario requires an integrated comprehension of human occupation dynamics and its implications for the emergence of new anthropogenic sources, mangrove degradation and remobilization of contaminants, increasing the needs for an updated assessment of human impacts. Thus, the present study aims to: (i) perform a temporal analysis of landcover use applying Geographical Information Systems (GIS) technologies for quantification of mangrove losses and urban and industrial expansion; (ii) determine the geochemical composition of sediments (elemental and mineralogical), identifying anthropogenic sources and sedimentary biogeochemical processes occurring in sediments; (iii) assess the risks of man-induced disturbances on the coastal zones of the Sepetiba Bay, regarding the metal contamination of sediments.
2 Study area
The Sepetiba Bay is a lagoon-estuary of about 519 km2 with a mean surface area of 427 km2, a volume of 2.56 × 109 m3 and a mean depth of 6 m (Molisani et al., 2004) (Fig. 1). The local geology is formed by Quaternary sedimentary deposits of alluvial environment (fluvial, fluvio-marine, and fluvio-lacustrine), superimposed onto a Pre-Cambrian framework composed by granites, gneisses and migmatites rocks (Fig. 1, Brönnimann et al., 1981; Roncarati and Carelli, 2012).
Water circulation in the bay is controlled by tides (Leal Neto et al., 2006; Roncarati and Carelli, 2012). The seawater that enters the bay as a bottom current is relatively cold and dense. It circulates clockwise through the bay, becomes warmer, and exits between the southwestern islands and Marambaia Sandbank (Fig. 1, Borges and Nittrouer, 2016). The lagoon hydrodynamics tends to deposit most sediments in the tidal flats and mangroves along the northeastern and southeastern coastal area of the bay (Montezuma, 2012; Roncarati and Carelli, 2012).
In the southeastern region, the low energy of the lagoon promotes the accumulation of sediment and the formation of a broad tidal flat in Enseada das Garças area (Fig. 1), which extends approximately over 40 km2 and represents the transition between the marine and terrestrial environments, including a vast mangrove forest (Roncarati and Carelli, 2012). The Guandu River catchment is the main fresh water source of the Sepetiba Bay and its waters reach the inner bay mainly through the São Francisco channel, with an annual flow of 6.5 × 109 m3, representing 86% of the total freshwater runoff input (Fig. 1, Molisani et al., 2004).
In the 1960s, a large industrial park began to be implanted, inducing unstructured sprawled urbanization, drastic landscape alterations, sewage release into the environment, and metal contamination by metallurgic activities (Molisani et al., 2004). Between 1960 and 1990, an electroplating plant operated close to the mangrove of Saco do Engenho (Fig. 1). The wastes generated by the electroplating processes (estimated at 600,000 tons) were exposed to the open air and lixiviated during rainfalls, reaching surrounding mangroves and the bay through a small marine channel located in the Saco do Engenho mangrove, considered as the contamination hot-spot area (Fig. 1) (Molisani et al., 2004).
3 Methodology
3.1 Analysis of landscape use in satellite imagery
To assess the landscape changes of the Sepetiba Bay during the last 30 years, two scenes from Landsat-5 (1985) and Landsat-8 (2015) satellites were used (http://EarthExplorer.usgs.gov). The images have a spatial resolution of 30 m associated with 7 and 11 spectral bands, respectively. First, both images were calibrated radiometrically from DN (digital numbers) to radiance values in units W/(m2·sr−1·um). Then, an ortho-rectification process allowed us to geometrically correct the scenes and mitigate the deformations from topography using the Shuttle Radar Topography Mission (SRTM) digital elevation model at 1 arc second (30 m). During this step, a co-registration process between the two Landsat images was made using 20 control points between the two scenes, obtaining an adequate overlap between both rasters. Next, a supervised classification was applied using the mathematical operator “Maximum likelihood classifier”. This method considers the weighting of the average distances, using statistical parameters of distribution of pixels within a given class (Alatorre et al., 2011; Hossain et al., 2009; Meneses and Almeida, 2012). Training areas were associated with each class to evaluate natural vegetation, pasture, agriculture, mangrove, hydrography, sand extraction, urban and restinga/beach areas. Subsequently, the classes were smoothed and generalized using the Majority Filter tool. Finally, both raster classifications were converted to polygon-type vector, which were then parameterized and compared according to the area of each of the classes in the two scenes. The differences of areas in square meters between the different classes were compared for an identical spatial subset in both images and compared in relation to their percentage area. The degree of uncertainty of the model was measured during the co-registration process between the two scenes. The root-mean-square error (RMSE) between the control points over the two Landsat scenes gave a value of 5 m, which is within the range of acceptable error in the co-registration process (1.5 times the nominal value of the pixel).
3.2 Sampling
We collected sediment cores from different locations within the Sepetiba Bay (Fig. 1). Core T1 was sampled in a white mangrove (Laguncularia racemosa) stand, located into the Saco do Engenho tidal creek, representing the contamination hot spot due to the leaching process of electroplating wastes. The other cores were collected in subtidal or intertidal mud flats located along the inner bay areas. Core T2 was collected near the Saco do Engenho channel mouth, while core T3 was taken near the mouth of the São Francisco channel. Core T4 was collected in Enseada das Garças, in a tidal flat located in the northeastern bay area, close to an ecological reserve with mangrove vegetation, but under the influence of anthropogenic activities, such as the discharge of untreated domestic sewage. Finally, the T5 core was collected near the Marambaia sandbank and the main communication of the bay with the open sea. All sediment cores were collected using acrylic tubes (6 cm in diameter and 60 cm in length), and sectioned in the field at 5 cm intervals. Each sub-sample was stored in polyethylene bags and kept frozen in thermal boxes with ice packs.
3.3 Sample treatment and geochemical analysis
The sediment samples were dried at 40 °C, crushed and sieved at 63 μm. This grain-size fraction (< 63 μm) was used for elemental and mineralogical analyses. For trace element analysis, different aliquots of 20–100 mg of sample were digested separately in Savillex® Teflon beakers on a hot plate using a multiple-step acid procedure with HF, HNO3, and HCl.
All chemical procedures for trace elements were performed under clean-air conditions and evaporations were conducted in clean boxes. All reagents were prepared using < 18.2 MΩ H2O (Nanop System®) and ultra-pure acids (Merck®) distillated by sub-boiling in Teflon stills, except for major elements analysis of sediment samples. For major elements analysis, sediment aliquots of 20 mg were weighted in platinum crucibles and digested by alkaline fusion with lithium metaborate followed by dissolution in 2 M HCl. Blanks and certified reference materials (BCR-2 and BHVO-2 basalts, the USGS 1646a estuarine sediment) were processed in each batch of sediment samples.
The elements Al, Fe, Ti, Ca, Mg, P, Mn, Zn, Cu, Ni, and Cr were analyzed using inductively coupled plasma optic emission spectrometry (ICP-OES, Spectro Ciros Vision, Spectro, Laboratory Campus®, Paracatu, MG, Brazil), while K was analyzed by flame absorption atomic spectrometry (FAAS, Analyst 200, PerkinElmer, Laboratory of Geochemistry, University of Brasilia). The Al, Fe, Mn and Zn data were previously published by Araújo et al. (2017a). Here, we complete the dataset with other elements.
The trace metals Cd, Pb and As elements were analyzed by inductively coupled plasma mass spectrometry (ICP-MS, Thermo Fisher Scientifics, Xseries 2) with In and Re as internal standards following Aries et al. (2000).
The mineralogical composition was determined by X-ray diffraction (XRD), using a RIGAKU Ultima IV diffractometer (at the University of Brasilia, Brazil). The XRD patterns were obtained for powders (0–2 mm fraction) and air-dried, using a Ni-filtered Cu Kα radiation and a graphite monochromator with an operating voltage of 45 kV, a beam current of 15 mA, and a scanning speed of 2°/min between 2 and 80° 2θ. For elemental analysis of minerals phases, an energy-dispersive spectroscopy (EDS) analysis was done using an electron microprobe (Jeol JXA-8230), with scanning electron microscopy (SEM). The sediment samples were deposited in double-sided tape over laminar glass. The samples were coated by gold sputtering using the coating system of Quorum Q150R ES.
3.4 Enrichment factor
The Enrichment Factor (EF) of trace elements was used to identify and quantify anthropogenic impact:
where [X] is the concentration of the element of interest and [Al] is the reference element concentration with no significant anthropogenic source, whereas ([X]/[Al]sample) represents the concentration ratio in the sample, ([X]/[Al])reference the concentration ratio using values reported for the Upper Crust by Wedepohl (1995).
3.5 Multivariate statistics
The dataset of sediment cores, a total of 16 variables and 52 samples, was interpreted through a multivariate approach (Principal Component Analysis [PCA]) using SPSS® (IBM). PCA analysis is a statistical technique used to examine and identify the main interrelations among a set of variables (e.g., elemental concentrations) (Voudouris et al., 1997). A correlation matrix with varimax rotation was used to calculate the factor loads and the sample factor scores (Mar da Costa et al., 2016; Mulholland et al., 2012). The principal components (PCs) that had eigenvalues higher than one were selected for discussion (Voudouris et al., 1997). The communalities values of all variables equal or higher than 0.5 were used as criterions for PCA results acceptance.
4 Results and discussion
4.1 Sediment geochemistry and multivariate statistics for the identification of sources and biogeochemical processes
The mineralogical assemblage of sediment consists of different proportions of primary minerals, i.e. quartz, feldspar, and amphibole, and of secondary minerals, i.e. smectite, illite, kaolinite, and gibbsite. In minor proportions, our XRD analysis detected the presence of pyrite, ankerite, and calcite. Ankerite (Ca(Fe,Mg,Mn)(CO3)2) can be related to diagenetic formation, and the presence of Fe(II) and Mn (II) can be associated with reducing conditions (Gueiros et al., 2003).
Scanning electron microscope (SEM) images show a mixture of pyrites, diatom shells, organic matter, and clay minerals (Fig. 2). Chemical analysis using Energy Dispersive Spectrometry (EDS) evidenced sediments predominantly composed of clay minerals (SiO2 and Al2O3 > 70 wt %), quartz particles (SiO2 > 72 wt %), and organic matter (associated with inorganic particles). The occurrence of pyrite evidences sulfate-reduction stimulation in mangrove, mud, and tidal flats. As pyrite formation corresponds to the last stage of a sequence of reactions involving the crystallization of Fe sulfides, its presence is indicative of intense sediment diagenesis (Huerta-Diaz and Morse, 1990; Morse and Luther, 1999).
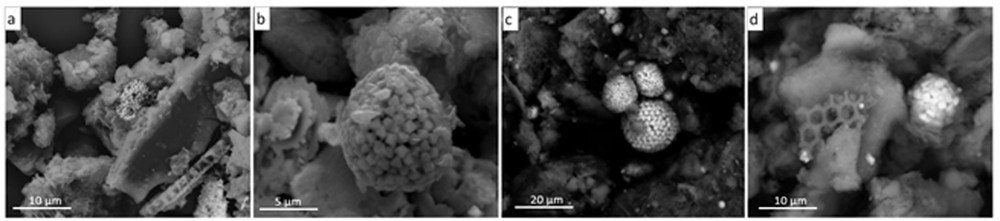
Scanning electron microscope (SEM) images of sediment cores from the Sepetiba Bay: (a) framboids and diatoms; (b) framboids in details; (c) association of many framboids; (d) framboids coated by clay minerals.
PCA explained almost 73% of the total data variance. Principal component 1 (PC1) accounts for 34% of the variance, including the elements Ca, Mg, Mn, Ni, and Cr, while principal component 2 (PC2) accounts for 27% of total variance, and includes Zn, Cu, As, Pb, and Cd. PC3 accounts for 12% of the total variance, and includes Si, Ti, and K. The plots displaying the variable factor loads and the sample factor scores of PC1 and PC2 are shown in Figs. 3a and 3b, respectively. The association of Si, K and Ti represents the terrestrial detrital material reaching the bay, i.e. primary and secondary minerals originated from weathering and erosion of silicate rocks and soils.

The PCA analysis of sediment cores from the Sepetiba Bay: in (a) and (b) are shown the main components, while (c) and (d) display the factor scores of sub-samples of sediment cores.
Core T3, located in the São Francisco estuary, displays the largest factor score for these elements, suggesting a major contribution of terrigenous materials (Fig. 3d) at this region. These results are in line with the great content of primary minerals and the presence of 2:1 phyllosilicate found at this sampled site, evidenced by XRD analysis. The association of As, Cd, Cu, Pb, and Zn is linked with the waste produced by the electroplating industry from the 1960s to the end of the 1990s. The T1 sampled site presented the highest factor associated with these elements (Fig. 3c), supporting the assertion that the Saco do Engenho regions is the main hot spot of metal contamination.
The T5 core (collected close to the bay's connection to the sea; Fig. 1) is strongly associated with marine carbonate-derived elements, i.e. Ca and Mg. It presented high Mn concentrations along its entire profile (> 1000 μg·g−1, Table 1, Supplementary material). These three elements can be associated with chemically formed or biogenic carbonates deposited in the sediments. The diagenetic processes in sediments under anoxic conditions promote Mn(IV)-oxyhydroxides reduction to Mn(II), which is mobilized into the pore water (Burdige, 1993; Emerson and Hedges, 2004; Neumann et al., 2002). Part of the Mn liberated into pore water may later precipitate as Mn-oxides or carbonates scavenging trace metals of the water column (Burdige, 1993). These processes likely explain the association of Ni and Cr with Mn, Ca and Mg in the PC1 (Fig. 3). This interpretation is supported by the investigations on Mn behavior on tidal flats and mangrove sediments of the Sepetiba Bay performed by Gueiros et al. (2003). These authors suggest a significantly diagenetic remobilization of Mn and, potentially, of other trace metals, to pore water, and its posterior transport to adjacent environments.
Phosphorus had moderate factor loads in PC1 and PC2 (Fig. 3a, b), likely indicating a dual origin arising from anthropogenic sources (e.g., domestic wastes, industrial effluents, and farming activities) and natural processes related to the weathering of rocks and vegetation and biomass organic matter (Rodrigues et al., 2012).
4.2 Enrichment factor of trace metal profiles
The trace metal EF profiles are shown in Fig. 4. The average, minimum, and maximum EF values of sediment cores are included in the Supplementary material (Table 2). The T1 core, located at Saco do Engenho mangrove close to the old metallurgic zone, shows the highest average EF values for As (20), Cd (874), Pb (8), Zn (303), and Cu (11). As shown by PCA interpretations, these results evidence the association of these elements with the historical metallurgic waste contamination. Conversely, Cr and Ni showed low and homogenous EF values (≤ 2) in all sediment cores profiles, indicating negligible anthropogenic inputs into the bay for these elements. This result is in line with Wasserman et al. (2001) observations, which did not find any association of Cr with anthropogenic sources in the bay's sediments.
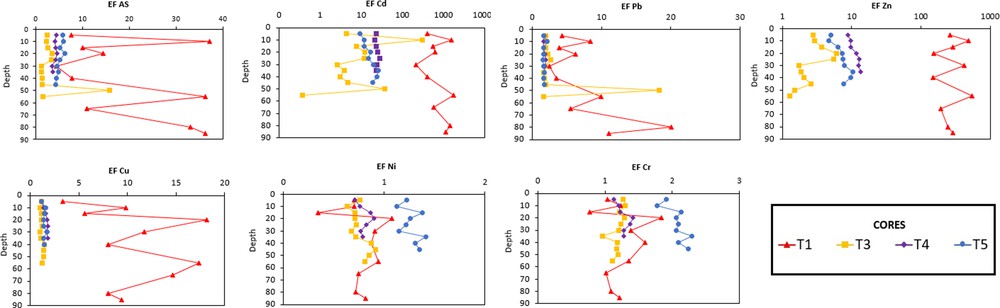
Enrichment factor of trace metals of sediment cores from the Sepetiba Bay. T1 core, Saco Do Engenho; T3, São Francisco Channel; T4, Enseada das Garças; T5, Marambaia.
While As, Pb and Cu present high concentrations in the T1 core, these elements show monotonous tendencies in EF of T3, T4 and T5 cores (except for As and Pb EF peaks in the T3 core at 45–50 cm, Fig. 4). In the T1 core, Cu EF values range from 3 to 20, while in all others cores they remained lower than 2. This indicates that anthropogenic Cu is immobilized in the Saco do Engenho mangrove system, probably due to its high affinity with organic matter or insoluble sulfide formation (Adriano, 2001; Andrade et al., 2012).
Similarly, high Pb EF values (> 2) are restricted to the Saco do Engenho mangrove area. These results suggest that new diffuse sources in the watershed of the bay related to urban sprawl and industrialization processes did not impose significant inputs of anthropogenic Pb into the inner bay. Prior analysis of Pb isotope compositions ratios (207Pb/204Pb and 206Pb/207Pb) of bottom sediments in the Sepetiba Bay showed that these ratios range between 1.188 to 1.341, and defined a linear trend between two probable end members: the oceanic water source (ratios between about 1.335 and 1.341) and the old metallurgic industry (between 1.118 and 1.190; Cunha et al., 2009). Lead occurs in significant concentrations in Zn and Cd in ore deposits (Adriano, 2001), hence, its origin should be associated with the Zn ore used in the old metallurgic industry. Thus, the high Pb EF values in T1 core (Saco do Engenho) shows that the old metallurgic wastes still constitute the main anthropogenic source of Pb even after waste removal episode.
The peak of Pb EF at the base of T3 core (at 45–50 cm) does not coincide with the Zn peak, which is correlated with the beginning of the metallurgical Zn refining in the bay in the 1960s (Gomes et al., 2009; Marques et al., 2006; Molisani et al., 2004). This Pb EF peak may be associated with the manufactured materials used in the construction of the artificial channels (São Francisco Channel).
The As EF values normalized against the Upper Crust for the T3, T4 and T5 cores are elevated, but the As concentrations in the sediment profiles (Table 2, Supplementary material) are similar to the mean As concentrations in shales (13 μg·g−1, Wedepohl, 1995). Also, the As concentrations found in the inner bay (average of 11 μg·g−1) and Saco do Engenho mangrove (average of 51 μg·g−1) in this study are significantly lower than the average concentrations of about 37 and 221 μg·g−1 reported for the same areas in a previous study (Magalhães et al., 2001). This observation may indicate an attenuation of As metallurgic contamination and a limited exportation of this element from the heavily impacted mangrove of Saco do Engenho to the bay.
Zn and Cd are exported from the hot spot to the whole bay area, as evidenced by the high EF values found in the cores T3, T4 and T5 (Fig. 4). Despite the end of metallurgical activities in 1998 and the removal of metallurgic wastes in 2012, the levels of Zn and Cd contamination are still elevated in all cores, probably due to remobilization of Zn-enriched sediments from the old metallurgic zone (Saco do Engenho mangrove) to the bay during tidal cycles. The hydrodynamics of the system continuously re-suspends sediments, leading to their long-distance transport, and remobilizing and dispersing the contaminants throughout the bay (Barcellos et al., 1997).
Isotope geochemical models of Zn have confirmed that the old metallurgic wastes continue to be the major Zn anthropogenic source, but diffuse sources may have more importance in the anthropogenic Zn loading in the future (Araújo et al., 2017a). However, the high load of Zn that is still present at the old metallurgical wastes may mask the influence of diffuse source contamination.
4.3 Landscape use changes in the Sepetiba Bay watershed in the last 30 years
Fig. 5 shows the expansion of urban and built areas throughout the study region, with consequent reduction of mangrove areas and natural vegetation, and an increase in sand extraction areas between the years 1985 and 2015.
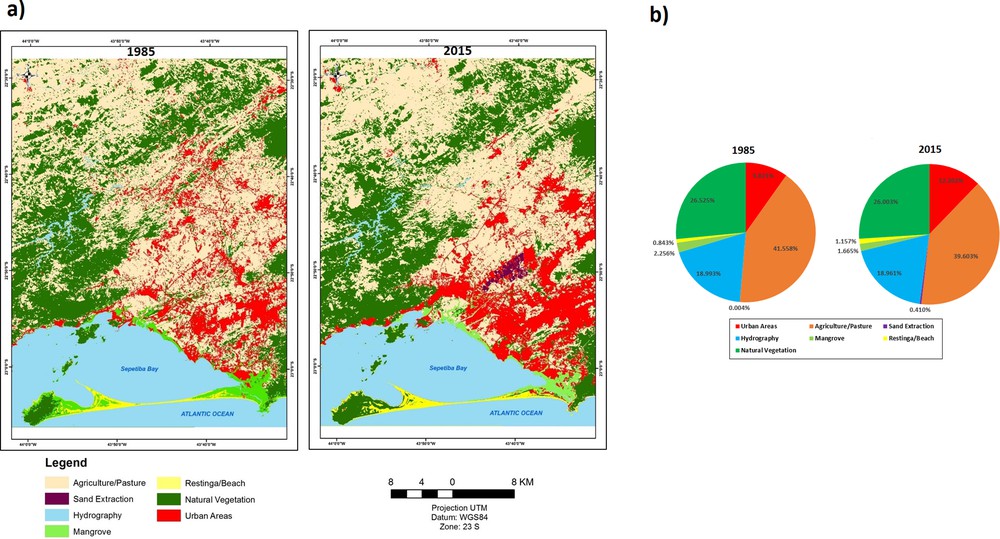
Analysis of landscape use changes in the Sepetiba Bay's watershed between 1985 and 2015.
The vegetation cover of the drainage basin reduces about 2 thousand ha between the periods analyzed, going from 99,144.99 ha (26.52% of the area) to 97,193.61 ha (26.03% of the area) (Fig. 5b). There is also a decrease in mangrove areas (reduction of 8431 to 6222 ha), corresponding to a loss of nearly 26% of the previous mangrove area (∼2% of the total area). The agriculture and pasture areas also declined from 155,331.45 to 148,025.43 ha, which represents a decreasing from 41.55 to 39.60% of the total area (Fig. 5b). The urban and constructed areas increased approximately 9000 ha (responsible for an increase of 9.82% of the total area to 12.20% of the total area). The sand extraction areas increased almost 100-fold, going from 15.12 to 1531.08 ha (i.e. from 0.004 to 0.410% of the total area of the Sepetiba Bay, Fig. 5b).
The decline of agriculture, pasture, mangrove, and vegetation areas is explained by the rapid industrial expansion and population growth in the Sepetiba Basin in recent decades. Currently, the Sepetiba watershed hosts several industrial plants encompassing different economic sectors: metallurgy, beverage, rubber, chemistry, textile, mechanics, plastic products, and clothing (Leal Neto et al., 2006). The satellite imagery analysis showed that the industrialization processes concentrate around ports and previous industrial park already installed. The mineral extraction in the region centers on sand exploitation and is an important source of the Sepetiba Bay pollution (Tubbs et al., 2011).
Considering that economic and population growth are underway in the Sepetiba Bay, there are risks of more losses of mangrove vegetation. Beyond ecological damages and loss of fauna and flora species, mangrove losses may imply an important input of metal contamination to the bay. As discussed before, the mangrove sediments (particularly those from the Saco do Engenho) are contaminant sinks. Considering the high metal concentrations on these sediments, possible mangrove removal or its conversion into urban and/or industrial areas could increase the risks of a large releasing of toxic elements to waters of the Sepetiba Bay.
5 Conclusion
Multivariate statistical analysis of concentrations of chemical elements in the sampled sediments discriminated their anthropogenic (Zn, Cu, Cd, Pb, and As), terrestrial (Si, Ti, K, Fe, and Al), and marine/biogenic or diagenetic (Ca, Mg, Mn, Ni, and Cr) origins. Sediment core profiles of trace elements evidenced records of former environmental impacts from old metallurgical wastes. Metal inputs associated with the emergence of new diffuse sources associated with urban and industrial expansion were not detected. It is possible that the high load of metals arising from the old metallurgic zone masks the presence of such sources.
Mineralogical analyses showed the presence of ankerite and pyrite, evidencing an intense redox cycling of Fe and Mn in mud flats and mangroves during diagenetic processes involving carbon–iron–sulfur interactions and microbial metabolism. The diageneses and subsequent modification on sedimentary mineralogy may affect the trace metal retention within sediments.
The last 30-year changes in human occupation in the Sepetiba Bay was assessed, which indicated a severe growth of the urban areas, especially in the western zone of the Rio de Janeiro city. This feature has been associated with significant advances of urbanization over mangrove and vegetation areas. There was a loss of ∼26% of the previous mangrove area in the last 30 years, corresponding to an average loss rate of 0.9% per year, which is similar to the global trends. Since mangroves are an important sink of metals in the Sepetiba Bay, which attenuates trace element inputs into its aquatic system, their suppression may produce greater susceptibility of biota and humans to toxic contaminants. Moreover, their suppression, mainly in areas impacted by the old metallurgical activity, could cause the release and remobilization of significant loads of metal into the inner bay.
Future studies must focus on speciation analysis to better understand the bioavailability and toxicity of the trace elements, especially in the mangroves close to the old metallurgic plant (Saco do Engenho), where this condition is more critical.
Acknowledgments
This work was financially supported by CNPq (D.F. Araújo PhD grant) and MCTI 400029/2015-4 (post-doctoral grant), and by the different scientific projects listed hereafter: Marie Curie International Research Staff Exchange Scheme Fellowship within the 7th European Community Framework Program (NIDYFICS, No. 318123) and CNPq universal 445423/2014-5, CNPq–PQ 310750/2014-8, FAP-DF 193.000.932/2015. This work also benefited from the Ciência Sem Fronteiras program (C. Quantin and Patrick Seyler) PV: 406288/2015-1 and PVE 400329/2014-0. The authors thank the assistance of Luciana Pereira in the SEM/EDS analysis and of Timothy Mulholland for revising the English grammar.