1 Introduction
Coastal areas now host more than half the world's urban population and most population growth now occurs in these areas (e.g., Barragán and de Andrés, 2015) because of the combination of favourable conditions such as easy access and transport and lower cost of infrastructure (e.g., Nouri et al., 2008). This rapid and intense socio-environmental transformation is significantly modifying fluxes of surface waters and groundwaters, in both quantity and quality, as shown by examples in southern California (Townsend-Small et al., 2013) and in the Recife area, NE Brazil (Bertrand et al., 2016), respectively. The combined consequences of climate change and multiple forms of anthropization are affecting urban coastal areas at all latitudes, but semi-arid regions are particularly at risk.
Today, climate change primarily takes the form of an increase in temperature, which induces a global rise in sea level, estimated at about 1.7 mm per year over the last century (Church and White, 2011). The rise in sea level particularly threatens low-lying coastal areas. According to forecasts by global atmospheric circulation models, climate change could also increase rainfall variability, which is already high in semi-arid areas. In their present state, these models cannot reflect the marked heterogeneity of today's climate in semi-arid areas. Their reliability in forecasting more numerous extreme events should consequently be considered with caution, but potential risks should be taken into consideration, whatever the uncertainty on forecasts. More frequent storms would endanger shore bars already weakened by urbanization like near Alicante, SE Spain (Pagán et al., 2016). In addition to the increasing number of extreme events, a decrease in monsoon precipitation could occur in West Africa, especially in the Sahel region (Defrance et al., 2017), thereby threatening groundwater recharge.
The combination of climatic and anthropogenic pressures affects all components of coastal socio-environmental systems. The movement of the interface between seawater and freshwater in aquifers is driven by the sea level rise and by the increased exploitation of water resources, as shown by Sefelnasr and Sherif (2014) in the Nile Delta, northern Egypt, and Jorreto et al. (2009) in southeastern Spain. Another example of combined pressures is the increase in surface runoff, which could be aggravated by more violent rainfall events, as well as the increase in impermeable urban surface areas (Scalenghe and Marsan, 2009). Hydraulic works in catchments and along the seashore can modify the process and the location of coastal sedimentation, leading to significant changes in the coastline over time, which can reinforce or counterbalance the impacts of climate change like in the case studied in southeastern France by Bony et al. (2011). An increase in the vulnerability of coastal areas in the coming decades, notably in semi-arid areas, is highly probable, but its fine assessment is largely uncertain due to the rapid environmental and social changes and rearrangements in these regions (Hinkel et al., 2014).
Even more than in other West African countries, in Mauritania, the coastal fringe plays a fundamental role in the national economy. The capital Nouakchott is home to about one third of the country's population. The anarchic expansion of the city has covered dunes and interdune depressions and drastically changed fluxes of both surface water and groundwater (Nouaceur, 2013). The city is now threatened by excess water, which is rather a paradox in an arid region. Nouakchott groundwater dynamics reflects its urban expansion, with its associated changes in land use, losses from the water distribution networks, infiltration from sewage systems, and evaporation of groundwater in the topographic depressions. A major change is related to the Nouakchott water supply. Between 1960 and 2011, the city water was pumped from the Trarza aquifer in the Idini well field, 60 km away in the southeast. Since 2011, this supply has been replaced by the transfer of surface water from the Senegal River through pipes.
We reconstructed the different steps leading to the present state of the Quaternary water table by analysing former reports, which were compared with and complemented by present measurements. We interpreted these measurements at different scales in space (from the piezometer to the region) and over time (from the day to the decade) to identify the different climatic and anthropogenic drivers of changes in the quantity and quality of groundwater, and their relative importance in the different areas of Nouakchott. We used several independent approaches to analyse the different sources of water present in the aquifer: hydrodynamics, focused on variations in the water table level; geochemistry (major ions, Br−); stable isotopes (18O and 2H).
2 Socio-environmental context
2.1 Environmental context
The city of Nouakchott is mostly built in the Aftout Es Sahli geomorphological depression, which runs parallel to the coastline. The depression depicted in Fig. 1 has an elevation ranging from 1 m below sea level (−1 m asl) to 1 m above sea level (+1 m asl), and it extends 200 km south from the Senegal delta to Sebkha N’Darmcha, 40 km north of Nouakchott. Before the construction of dams to control the flow of the Senegal River and to limit the seasonal inflow of seawater to a distance of 200 km upriver, this depression allowed extreme floods, like those that occurred in 1889 and 1950, to reach Nouakchott (Duchemin, 1951).
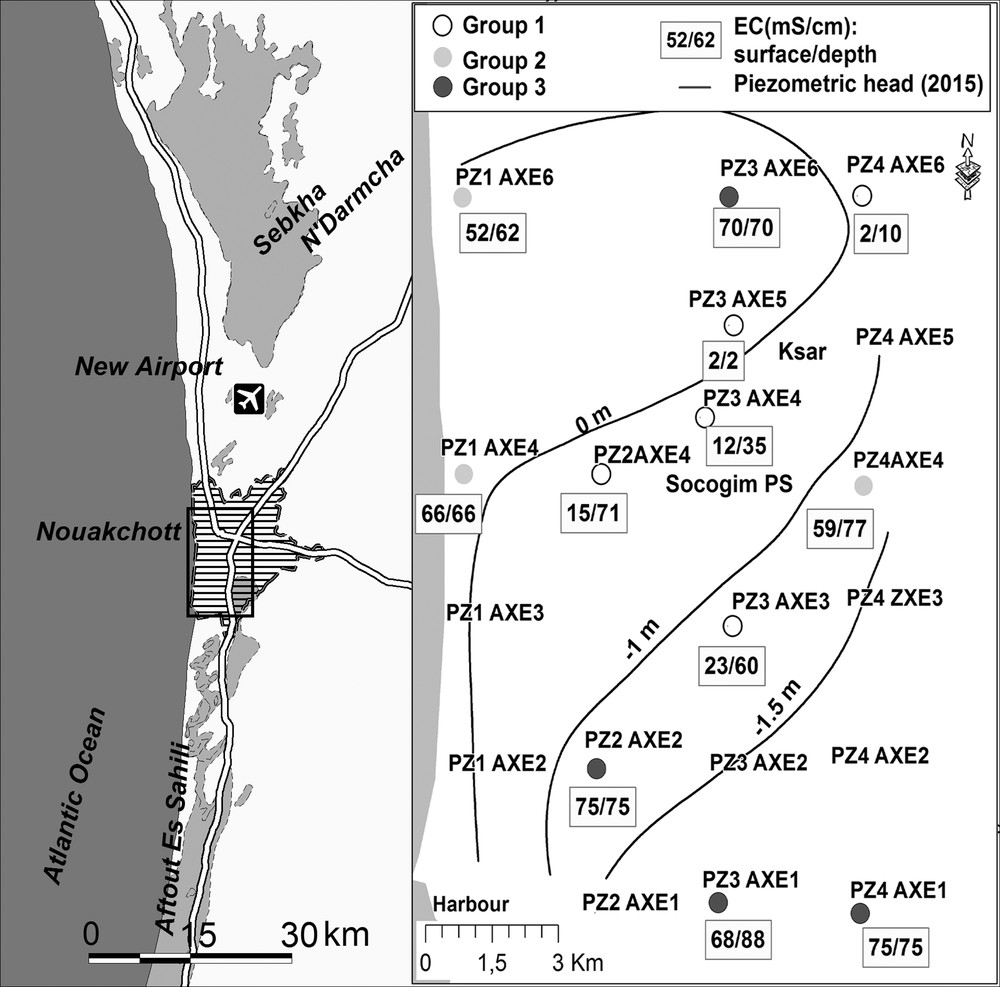
Left: location of the study area. Right: field campaign in March 2015, piezometric contour lines (m asl), and electrical conductivity (EC) of groundwater at the top and bottom of wells. The mention of groups 1, 2 and 3 refers to the geochemical classification detailed in § 4.2.
The Nouakchott area is a part of the large Senegalese–Mauritanian sedimentary basin, whose present morphology has been shaped by a series of dry and humid periods in the recent Quaternary. The region is comprised of, from east to west:
- • a series of NE-SW Ogolian dunes (20,000–12,000 years BP). Interdune depressions present white lacustrine deposits (Nguer and Rognon, 1989);
- • a plain with a mean width of 4 to 6 km and an elevation below, or close to, sea level. Evaporation is particularly active in the sebkhas (temporary salt lakes) which are the remains of former Nouakchottian lagoons (5500 years BP);
- • a narrow coastal dune bar, which protects the plain from the sea. The elevation of these Tafolian dune deposits (4000–2000 years BP) is less than 6 m amsl. Their width is 150 m on average, with extreme values of 300 m and nearly 0 m.
The climate is typically Sahelian, with a short rainy season between July and October, and a long dry season. Average annual rainfall in Nouakchott between 1931 and 2016 was 106 mm, with extreme values of 3 and 207 mm, and a standard deviation of 65 mm (source National Office of Meteorology). Like everywhere in the Sahelian zone (e.g., Lebel and Ali, 2009), daily rainfall is highly variable, and intense rainy events are not rare (e.g., 183 mm on August 15, 1932; 76 mm on August 23, 1958). Consequently, the urban area of Nouakchott regularly suffers from severe floods like those in 1932, 1950, 1995, 1999, 2000, 2004, 2006 (Thénot, 2007), and more recently in 2010 and 2013, due to high runoff and stagnation of water in the lower parts of the city.
In Nouakchott, groundwater is found in the Trarza Quaternary aquifer, which extends south over about 40,000 km2 from the Senegal River and north to Sebkha N’Darmcha, and from the Atlantic Ocean to the west to the Mauritanides mountain range to the east. The aquifer is made of vertical and horizontal alternations of very heterogeneous detrital material (sand, clay, shell beds) of lacustrine, aeolian, and marine origins. In this wide aquifer, the general flow is from the south to the northeast (Mohamed et al., 2014), but, in the Nouakchott area, the proximity of the sea and the major anthropogenic disturbance significantly alter this general pattern. The water table is presently between 0 and 5 m below the ground surface, i.e. at an elevation between −2.0 and +0.4 m asl.
2.2 Socio-economic context
Hastily conceived as the capital of Mauritania in the mid-1950s, the city of Nouakchott is now an important metropolis. Its population increased from 500 inhabitants in 1957 to 40,000 in 1970, to more than a million in 2015. This rapid population growth has been fed by a continuous inflow of rural populations, especially during the catastrophic droughts that affected the whole Sahelian region in the 1970s and 1980s. The attractiveness of the city for business, education and politics remains a major feature of Mauritanian development, which explains why population growth in the capital is three times higher than the national mean. Today, the city extends over more than 180 km2. Its horizontal sprawl has been anarchic (e.g., Tanguy, 2003), and covers various areas, including the most fragile ones like those threatened by mobile dunes or hardly protected from the sea by the narrow shore bar (Nouaceur, 2013).
Since 2011, the Nouakchott water supply from the Senegal River is 120,000 m3 per day, i.e. twice the volume previously pumped from the Idini well field. This has led to an increase in the volume of water returning to the ground through losses from the distribution networks and through infiltration of waste waters. The progressive but clear rise in the water table since 2011 became particularly apparent when groundwater invaded cellars and topographic depressions in the city. Access to houses, schools and markets became much more difficult or even impossible, and the stability of buildings was threatened.
3 Available data
3.1 Historical data
Because of the high concentration of salts in the soil and deeper sediments in the Nouakchott area, groundwater has not been traditionally exploited by the sparse population, which explains the poor availability of former hydrogeological information. Unpublished academic documents, technical reports, and inhabitants’ oral accounts are thus valuable sources of information. We compiled the major former hydrodynamic information, including: (i) seven water levels measured in February–April 1966 in the area planned for a seawater desalination plant, and a detailed piezometric survey of two wells with hourly measurements over a period of two days (SIF, 1966); (ii) two water levels in the Ksar district (i.e. the city centre) in the dry and the wet season (BCEOM, 1964); (iii) 14 water levels measured in December 2012 for a geotechnical study (EDE/IRC, 2013); (iv) repeated measurements in 10 piezometers in July and September 2012 in the vicinity of the new international airport, 20 km to the north of the city limits.
We also compiled previous geochemical data. They are few and limited to analysis of the major ions in three wells in the city (SIF, 1966). Farther from the Nouakchott area, we also exploited geochemical information from the Idini pumping field, including 9 analyses of major ions between 1978 and 2012 and 19 values of δ18O–δ2H from Seméga (1995) and Mohamed (2012). We also used five analyses of major ions and nine values of δ18O–δ2H from the Senegal River (Mohamed, 2012).
3.2 New data
Over two months in 2013, the national water company (SNDE) conducted a daily survey of two piezometers in the Nouakchott harbour area. In March 2015, the National Centre for Water Resources (CNRE) installed a piezometric network of 20 piezometers in the city, among which 13 are still operational. Since that time, CNRE has conducted 20 surveys in its network, and we complemented them with three additional surveys.
We also measured the physical and chemical characteristics of groundwater (temperature, electrical conductivity [EC], pH, alkalinity) in situ during three field campaigns in March, April, and December 2015. The accuracy is ± 0.1 °C for temperature, 1% for EC and ± 0.02 unit for pH. In addition, in April 2015, we performed vertical profiles of groundwater EC in 19 wells. Measurements were made in both descending and ascending directions in the entire water column. We also collected 12 groundwater samples in March 2015 for analysis of major ions, bromide and isotopes (Table 1) by the GEOPS laboratory. The uncertainty on the ionic balance is less than 10% for all samples. Uncertainty on isotopic contents is ± 0.2 ‰ for δ18O and ± 2 ‰ for δ2H (expressed in ‰, according to the international V-SMOW standard).
Physicochemical composition of major ions and stable isotopes.
Group | Well | pH | EC | TDS | T | K+ | Na+ | Ca2+ | Mg2+ | Cl− |
NO3− | SO42− | HCO3− | Br− | BI (%) | δ2H | δ18O |
(µS/cm) | (g/L) | (°C) | mequiv/L | (‰ vs V-SMOW) | |||||||||||||
1 | PZ3AXE | 8.78 | 1530 | 0.9 | 26.60 | 0.73 | 8.99 | 1.32 | 2.24 | 9.59 | 0.88 | 4.48 | 0.75 | 0.02 | −8.4 | −32.97 | 4.65 |
PZ4AXE | 8.84 | 2200 | 1.3 | 24.90 | 0.54 | 10.35 | 8.06 | 3.01 | 12.05 | 1.24 | 6.08 | 0.50 | – | 5.0 | −27.24 | −3.42 | |
PZ3AXE | 10.10 | 10,570 | 7.2 | 25.60 | 8.41 | 86.11 | 11.23 | 5.24 | 67.30 | 13.05 | 29.15 | 0.73 | 0.07 | 0.3 | −29.57 | −3.55 | |
PZ2AXE | 7.05 | 13,950 | 9.9 | 27.10 | 7.96 | 109.11 | 17.00 | 30.66 | 100.11 | 9.24 | 47.20 | 0.77 | 0.12 | 2.3 | −27.88 | −3.32 | |
PZ3AXE | 7.84 | 22,300 | 14.6 | 26.30 | 7.68 | 171.74 | 31.27 | 37.74 | 168.72 | 18.29 | 45.48 | 0.60 | 0.32 | 3.2 | −20.78 | −1.98 | |
2 | PZ1AXE | 7.46 | 53,800 | 36.5 | 26.8 | 13 | 526.1 | 25.66 | 129.4 | 526.1 | 7.6 | 56.18 | 0.65 | 0.7 | 8.1 | 2.39 | 0.91 |
PZ4AXE | 7.57 | 53,500 | 37.2 | 28.2 | 11.4 | 484.23 | 35.91 | 105.8 | 569.7 | 0.18 | 71.66 | 0.59 | 0.7 | −0.4 | −14.05 | −0.69 | |
PZ1AXE | 7.35 | 43,100 | 36.5 | 24.5 | 10.1 | 427.83 | 19.08 | 106.1 | 585.5 | 10.7 | 65.04 | 1.48 | 0.9 | −8.1 | −1.02 | 0.35 | |
3 | PZ3AXE | 7.35 | 58,300 | 44.6 | 26.1 | 14.6 | 551.29 | 47.68 | 126.4 | 683.5 | 3.41 | 91.16 | 0.54 | 0.9 | −2.5 | −11.71 | −0.24 |
PZ3AXE | 7.63 | 58,000 | 44.7 | 27.6 | 13.8 | 530.38 | 28.11 | 136.3 | 733 | 2.93 | 74.31 | 0.56 | 1.1 | −6.7 | −3.34 | 0.38 | |
PZ4AXE | 7.51 | 64,000 | 49.9 | 29.5 | 15 | 594.42 | 66.34 | 147.4 | 754.6 | 3.31 | 115 | 0.68 | 1 | −3.0 | −6.88 | 1.06 | |
PZ2AXE | 7.66 | 62,200 | 49.3 | 25.5 | 14.9 | 642.88 | 27.13 | 151.2 | 788 | 0.32 | 74.44 | 0.57 | 1 | −1.6 | 1.25 | 0.9 |
4 Results
4.1 Hydrodynamics
The piezometric contour lines (Fig. 1) show that the main direction of the groundwater flow is from the west (the Atlantic Ocean) to the east, where the water table elevation is between −0.8 and −2 m below sea level. In the city centre (wells PZ3Axe6 and PZ3Axe5), a piezometric dome (0 to +0.4 m asl) marks an area where the unsaturated zone is particularly thin (0.6 to 1 m). The hydraulic gradient is very low, between 0.1 and 0.3 ‰. Horizontal permeability deduced from 14 short infiltration tests (duration 30 min) varied between nearly 0 and 4.2·10−4 m/s, with a median value of 1.6·10−4 m/s (EDE/IRC, 2013).
In Nouakchott, the water table level fluctuates during the rainy season. In 2015, when annual rainfall was 113 mm, the water table rose by 0.10 to 0.44 m (Fig. 2), with a median value of 0.27 m (n = 18). In 2016, when the annual rainfall was 86 mm, the water table rose by 0.04 to 0.23 m. The observation by BCEOM (1964) of a seasonal rise of 0.5 m is close to the present one in the same area (0.3 m in 2015 in the PZ3Axe4 piezometer). This is also similar to former observations by Mohamed et al. (2014) in rural areas of the Trarza aquifer, where the seasonal rise was found to vary between 0.2 and 0.4 m for a mean annual rainfall of 200 mm.
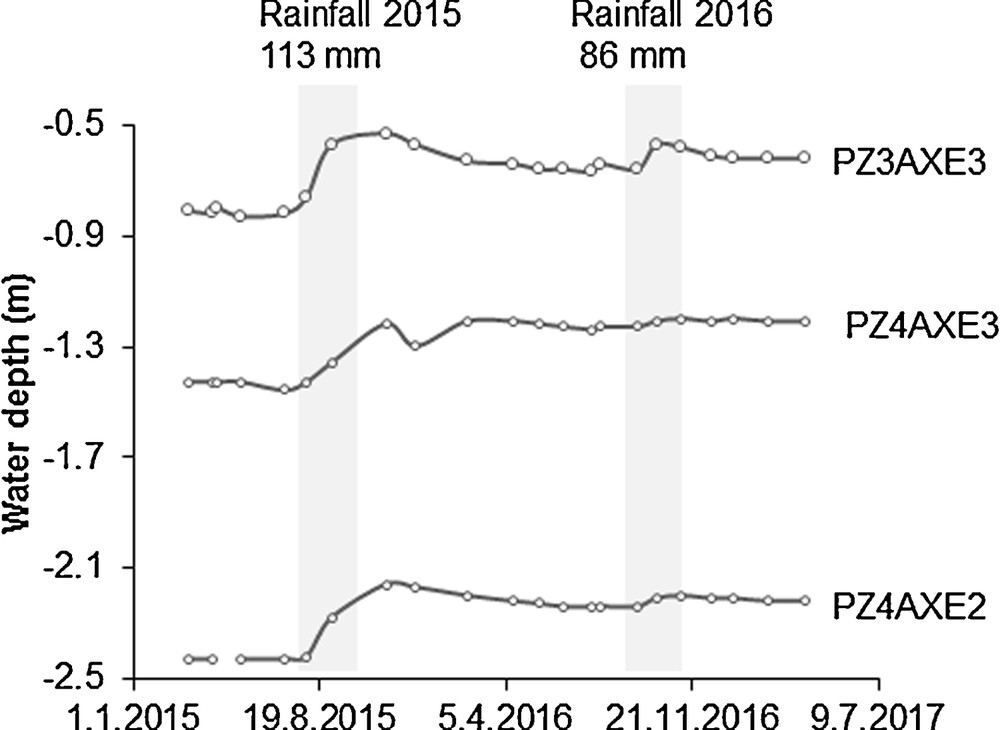
Impact of the rainy season on the water table level (asl) in three wells from 2015 to 2017 (location in Fig. 1).
The tidal amplitude (mean value of 0.93 m) is reflected by piezometric fluctuations close to the sea. The fluctuation attenuates as a function of the distance from the sea and local hydrodynamic characteristics of the aquifer. In the four piezometers surveyed at the foot of the shore dune, the daily variation of the water table was between 2 and 14 cm, with a median value of 4 cm, even in the dry season. This is similar to the 6-cm variation observed by SIF (1966) in a daily survey, and to the mean fluctuation of 8 cm measured by SNDE (2013).
An interannual rise of the water table was observed in 12 out of the 13 CNRE piezometers that are still functioning. The rise ranges between 0.05 and 0.22 m, from March 2015 to April 2017, with a median of 0.20 m (Fig. 2). The largest rise was observed in the wells of the city centre and in the southeastern part of the city. Measurements before 2015 are rare and discontinuous, and reconstruction of long-term changes in the water table levels is difficult. Near the shore bar, the water table appears to have remained relatively stable over the years: its mean present elevation (+0.15 m asl) is similar to that measured by SIF (1966). The relative stability of the water table near the sea is explained by the strong hydraulic control of the seawater on the groundwater level. Elsewhere, changes are more visible. A first example is at the eastern periphery of the city centre. In 1993, the groundwater table was deeper than 1.5 m below the ground surface, whereas its present depth is 0.3 m below the ground surface (Mohamed El-Mokhtar Ntaghry, pers. comm., 2017). A second example is the Socogim PS district, in the city centre. During the 1990s, it was one of the most expensive areas, but its value dropped dramatically after the 2013 floods. A 44 mm rainfall event combined with the rise in groundwater altered this district into an area with permanent surface waters. A third example is the area around the PZ3Axe4 piezometer. According to BCEOM (1964), the depth to the water table was 1.9 m below the ground surface in the 1964 dry season and 1.5 m in the rainy season, whereas it is now 0.7 and 0.4 m below the ground surface, in the dry and the wet season, respectively. Last, an indirect confirmation of the long-term rise in the water table level is provided by recent measurements near the new airport, 20 km north of the city limits. The airport area has a topography and hydrogeology comparable with those existing in Nouakchott in former natural conditions. The difference in the present groundwater elevation between these two areas is about 2 m (Fig. 3).
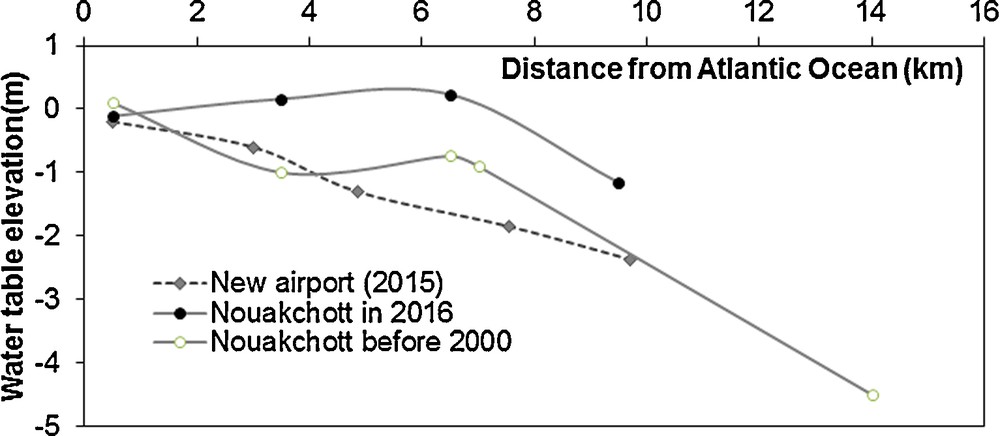
Long-term changes in the water table level (asl) in the city centre and at the new airport.
4.2 Electrical conductivity and concentrations of major ions in the groundwater
Electrical conductivity (EC) of groundwater reflects its total amount of dissolved salts. In April and December 2015, we measured EC ranging from 1.5 to 86 mS·cm−1. The lowest values were measured in the city centre and the highest in the recent suburbs in the SE and SW (Fig. 1). High EC values are frequent in the rural part of the Trarza aquifer (Mohamed et al., 2014), especially in the lower Senegal River valley, where seawater penetrated up to 200 km upstream of the present river mouth during the Nouakchottian transgression, 5500 years BP. Vertical profiles of EC provide another dimension of groundwater salinity, with various shapes of stratification, particularly in the city centre, where the interface between the upper brackish water and the lower more salty water is below a depth of 6 m (ESM 1).
All 12 groundwater samples that we collected in April 2015 are of Cl−-Na+ type. Na+ and Mg2+, Cl−and SO42−accounted for more than 90% of the ions (Table 1). According to a hierarchical classification based on major ions and stable isotopes, we ranked them in three groups (Fig. 1):
- • group 1 (5 samples) with a Cl− content < 170 mequiv/L, i.e. brackish waters. They are found in the city centre;
- • group 2 (3 samples) with Cl− content ranging between 500 and 600 mequiv/L, close the concentration in seawater. They are found near the coast line;
- • group 3 (4 samples) with Cl− content higher than that of seawater (680 mequiv/L). They are found in the sebkhas area.
The concentrations of most ions, especially Na+ and Mg2+, are correlated with the Cl− content, identifying seawater as the major source for groundwater mineralization. The conservative behaviour of Cl−allows the identification of further processes of dilution with freshwater and concentration due to evaporation (Fig. 4). However, the frequent excess of SO42− and Ca2+ with respect to the seawater dilution/evaporation line is probably due to the dissolution of gypsum. Up to now, a gypsum layer has never been mentioned in the geological description of boreholes, but gypsum is frequent in sebkhas like Sebkha N’Darmcha, where it has been exploited for the last 30 years. The only former analyses of major ions (SIF, 1966) resemble those in group 3 sampled recently in the same area, which indicates the permanence of the geochemical facies in this area near the shore bar.
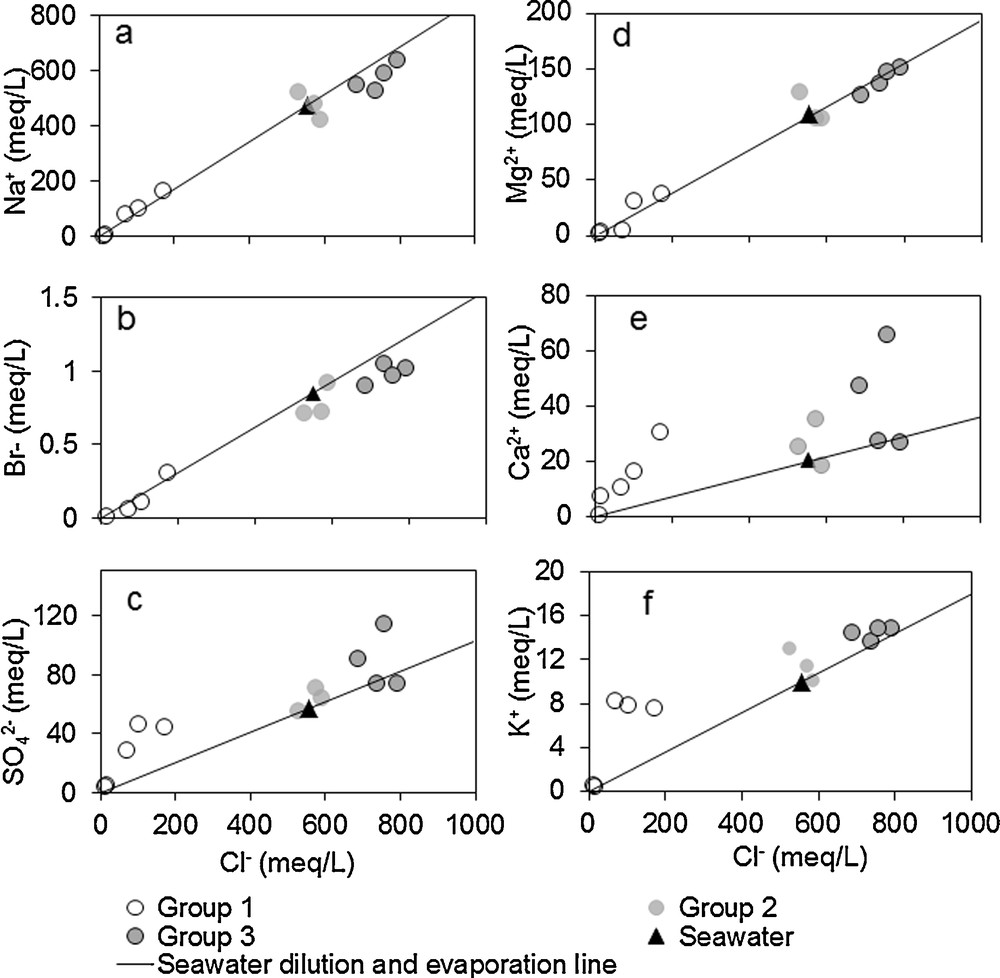
Identification of origins of groundwater by the comparison of the Cl− content vs. concentrations of other major ions and that of Br−.
4.3 Br−/Cl−ratio
The Br−/Cl−mass ratio is frequently used to identify the origin of salinity in groundwater (e.g., Louvat et al., 1999). The Br−/Cl−mass ratio of group 2, between 3.1·10−3 and 3.6·10−3, is similar to that of seawater (Fig. 4b), which suggests a major marine contribution to groundwater. group 3, which has the highest mineralization, falls near the seawater evaporation line with Br−/Cl−ratios of around 2.9·10−3. This suggests their marine origin and a later evaporation effect, without reaching halite crystallization (Zherebtsova and Volkova, 1966). Samples of group 1 are distributed around the seawater dilution line, which suggests a mixing of seawater and freshwater.
4.4 Stable isotopes
Values of δ18O and δ2H in our groundwater samples range from −4.65 ‰ to +1.00 ‰, and from −33 ‰ to −1 ‰, respectively (Table 1 and Fig. 5). These values are close to those observed by Mohamed et al. (2014) in the rest of the Trarza aquifer over a much larger area: from −5.6 ‰ to +6.9 ‰ for 18O and from −40 ‰ to +18 ‰ for 2H, with median values of −3.77 ‰ and −29.4 ‰, respectively.
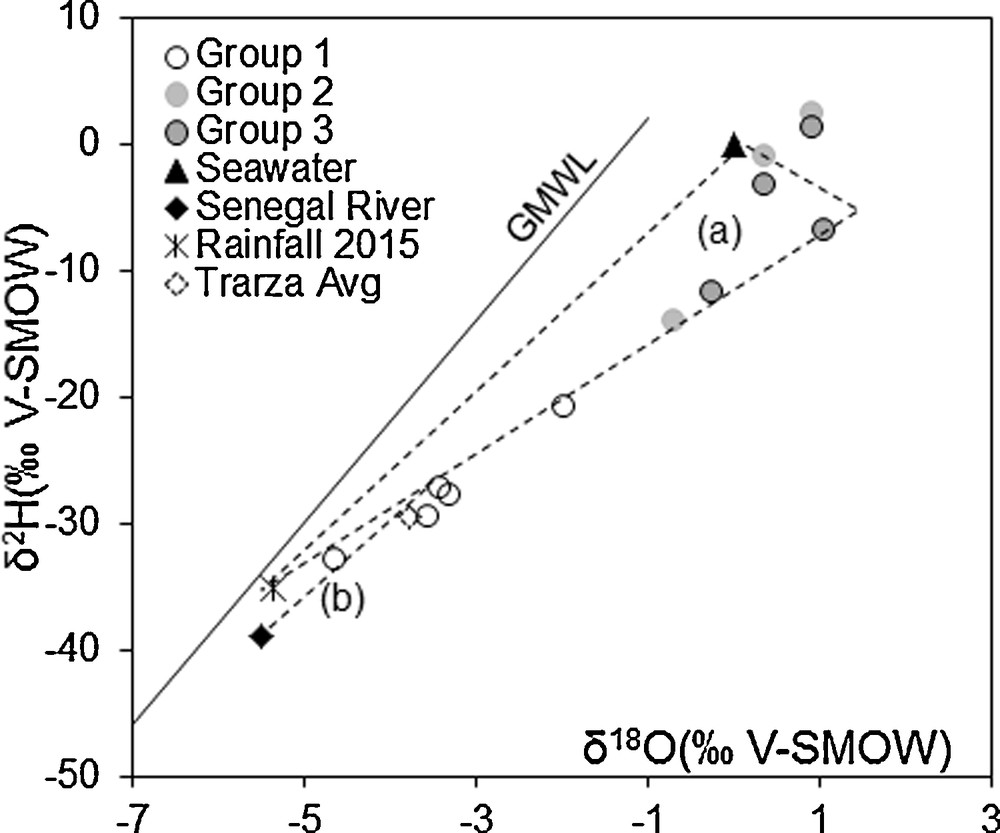
Identification of origins of groundwater by isotopic content (δ2H and δ18O). (a) represents the mixing domain between seawater or seawater affected by evaporation and rainwater. (b) is the mixing line between the Senegal river water and brackish water (variable proportion of seawater marked by evaporation and rainwater).
In the few rainfall samples that we could collect, δ18O and δ2H values range between −8.12 and −2.65 ‰, and between −56.9 and −12.8 ‰, respectively. These values are too few to define a local meteoric line. We can refer to the Global Meteoric Water Line (GMWL) defined by Craig (1961), and considered as representative of regional rainfall in the western Sahel (e.g., Gourcy et al., 2000). All our groundwater samples are below the GMWL and also below the line linking rainfall in Nouakchott and seawater, which indicates a significant evaporation uptake for many of them, especially for groups 2 and 3. The large range of values emphasizes the highly variable proportions of rainfall and seawater in groundwater. The samples from the city centre (group 1) have an isotopic content close to the mean Trarza values, but are more enriched than local rainfall (−5.35 ‰ for 18O and −35.1 ‰ for 2H) and also than the Senegal River now used for drinking water (−5.5 ‰ for 18O and −39.1 ‰ for 2H).
5 Discussion
5.1 Origin of water in the Quaternary aquifer
The Nouakchott groundwater originates from seawater, with a possible dilution by freshwater originating naturally from precipitation and runoff infiltration, and artificially from the infiltration of the water pumped in Idini and from the Senegal River. The diagram Cl−vs. δ18O (Fig. 6) clearly differentiates two families: on one side, samples of groups 2 and 3, with a high Cl−content and δ18O between −1 ‰ and +1 ‰, close to the sea values; on the other side, samples of group 1, much closer to the values of rainfall, of the Trarza aquifer, and of the Senegal River.
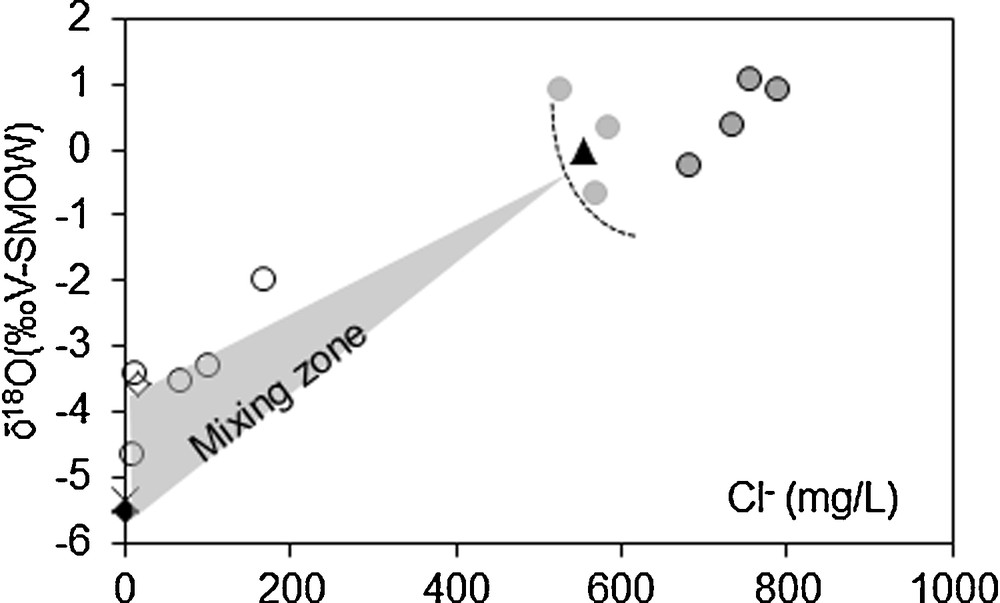
Identification of origins of groundwater by a δ18O vs. Cl− diagram (same symbols as in Fig. 5). The three geochemical groups detailed in § 4.2 are clearly differentiated.
The origin of groundwater in groups 2 and 3 is clearly seawater, with a very limited input from other sources of water, and a frequent marking by the evaporation uptake. This agrees with the geomorphological and sedimentary context, which allows a hydraulic connection between the sea and the aquifer, and with the regional hydrodynamic pattern showing a flow from the west to the east. Seawater can also invade the coastal area during temporary submersion events (see § 5.2). The presence of seawater has also been observed in other areas of the Trarza aquifer, north and south of the capital (Mohamed, 2012; Seméga, 1995).
In group 1, the proportion of seawater is much smaller, and a large part of groundwater derives from freshwater. Despite the arid climate, rainfall can recharge the aquifer during the most intense rainy events, especially when rainfall concentrates in topographic depressions. Moreover, since the beginning of anthropization of the Nouakchott area, freshwater infiltration has mainly been due to artificial recharge through water losses from the drinking water distribution networks and through the return of waste waters to the ground. Unfortunately, the differentiation of the respective origins (rainfall, Senegal River, and “natura” Trarza aquifer) is presently uneasy because of the similarity of their geochemical facies.
Many groundwater samples collected in 2015 attest to evaporation, especially group 3. Evaporation affects water before it infiltrates into the ground, but it also affects groundwater because of the shallow depth of the water table and the slow velocity of the groundwater flow. According to empirical estimates by Coudrain-Ribstein et al. (1998), the annual evaporation uptake could be 70 mm for a water table at a depth of 1 m (and 6 mm for a depth of 5 m). The high rate of evaporation uptake is also illustrated in Sebkha N’Darmcha. This salty lake is assumed to have been isolated from the sea 4200 years ago, which explains the present δ18O content of 10 ‰ in its groundwater (Fontes et al., 1967).
The EC profiles (Fig. 7 and ESM 1) add a vertical dimension to this complex distribution. Depending on places, the interface between brackish waters and saline waters is sharp or progressive. Its vertical location highly depends on the recent anthropogenic recharge (higher depth in the city centre).
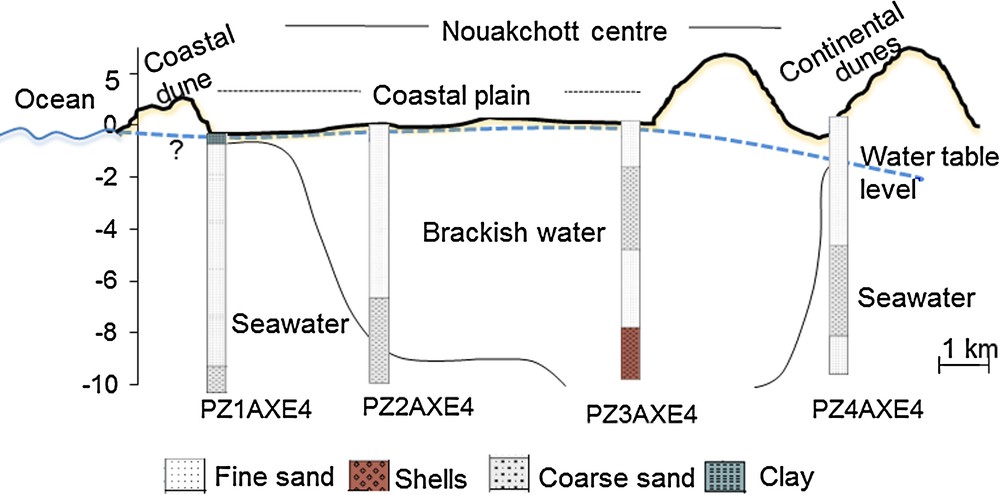
Hydrogeological cross section (west–east) and groundwater stratification (location of the wells in Fig. 1).
5.2 Sea level rise and sea submersion
Because of the hydraulic connection between the sea and the water table, the global sea level rise will cause a direct rise in the water table throughout the urban area. The extent of the districts of Nouakchott in which surface water is temporarily or permanently present will then increase significantly. This could be aggravated or attenuated by a parallel subsidence or uplift of the coastal region, but estimates of such geological changes are presently missing.
In addition to its underground influence, the sea level rise will also affect the coastal area through temporary submersion events. These can occur throughout the thin, unconsolidated dune bars, which do not always resist the most violent storms and sea surges. Anthropization has considerably weakened the shore bar through: (i) massive extraction of sand and shells for construction materials, (ii) increased coastal erosion caused by harbour works in 1987 and subsequent works up to 20 m of retreat per year in the 2005–2013 period (Ozer, 2014), (iii) intense traffic of pedestrians and vehicles over the dune. In recent decades, the main submersion events occurred (i) after a storm in 1987 when two breaks of about 20 m of width each appeared in the dune bar south of the harbour (Barry, 2003), (ii) in 1992 and 1997, when other breaks appeared north of the harbour.
5.3 Increasing urban recharge
The recent unusual repetition of floods in Nouakchott can be explained by a combination of independent factors: (i) very intense rainfall events; (ii) the proximity of the water table; (iii) the difficulty the water has draining away because of the topographical conditions; (iv) the increasingly impervious soil surface due to the expansion of urban infrastructures and intense exploitation of the most pervious layers of the aquifer like shell beds for construction purposes.
In fact, rainfall events have not increased significantly in recent decades, neither the annual total nor the number of events with more than 30 mm of rainfall (Mohamed et al., 2014), and even ‘normal’ rainfall can cause flooding in Nouakchott now (Ould Sidi Cheikh et al., 2007; Ozer, 2014). More frequent floods are thus primarily a consequence of anthropization, especially the lack of urban planning and the increased water supply since 2011. The higher pressure of water in the distribution network and the age of the network have also increased the number of leaks in recent years. According to unpublished estimates by the SNDE, about 40% of the distributed water is lost in this way, which represents a direct daily inflow of 50,000 m3 to the aquifer. Moreover, only the oldest urban districts built in the 1960s (i.e. the city centre) have a collective sewage network. This network is only 38 km long and its current state of repair is not known. The remaining 90% of the metropolis relies on individual sewage solutions in the form of sewage pits, septic tanks and cisterns that are never completely watertight. In addition, sewage tankers that collect waste water pour out their contents a few kilometres away from the city, thereby only shifting the contamination a very short distance away.
We estimated very roughly the impact of these new conditions. With an urban area of 180 km2, a daily loss of 50,000 m3 from the water distribution network and a return flow of waste water of 12 L per day per adult, the additional aquifer recharge would be of about 10 mm per year. Assuming a 10% porosity in the ground, the calculated rise in the water table would be close to the seasonal fluctuations that we observed in 2015 and 2016 (0.1 to 0.3 m).
The massive inflow of freshwater into the urban aquifer leads to a decrease in EC that can locally be as low as 1.5 mS·cm−1, while in groundwater samples, EC is often higher than 50 mS·cm−1. The brackish water/seawater stratification is also clearer in the city centre, where the interface is at a depth of about 6 m (Fig. 7 and ESM1).
5.4 Sanitary and environmental consequences
The groundwater rise also has sanitary and environmental consequences. The city now hosts numerous permanent waterbodies that did not exist in former natural conditions. They allow the multiplication of new animal and plant species. Cases of dengue have been reported recently in Nouakchott, where this disease was previously unknown (Mint Lekweiry et al., 2015). Another threat comes from the significant narrowing of the unsaturated zone. The rapid urban growth has increased sources of contamination, in number, location, and types of pollution. In places where groundwater reaches the ground surface, the direct connection with informal waste disposal multiplies the risks of chemical and biological contamination. Elsewhere, domestic waters evacuated without treatment in wells and tanks reach the saturated zone after a shortened transit time. The permanent presence of polluted water in topographic depressions and its potential exploitation for market gardening are real threats.
The geotechnical consequences of the groundwater rise are also very important. Most old houses were built, with no particular protection of their foundations against water, but the capillary fringe is now at their level. Saline groundwater actively corrodes construction materials and walls and buildings frequently collapse. The omnipresence of shallow groundwater complicates public works and requires dedicated equipment for permanent pumping. Asphalted roads are also affected by disturbances in soil stability. The desertion of private houses and official buildings in flooded areas involves considerable financial losses and redistributes the population and services in the urban area.
6 Conclusion
Like in many other semi-arid regions, anthropogenic drivers in the Nouakchott area now have a much greater influence on changes in the quantity and quality of the groundwater than climate change alone. This has already been observed in many rural regions of the Sahel, e.g., in western Niger (Leduc et al., 2001) and in northern Burkina Faso (Mahé et al., 2005), as well as in more densely populated rural areas of the Mediterranean region (e.g., Leduc et al., 2017). Urban areas undergoing rapid growth are expected to be even more affected by anthropization.
A similar situation prevails in many other semi-arid areas. For instance, in southwestern Niger, the capital Niamey is the meeting point between a groundwater rise over the whole rural surroundings and specific urban accumulations of surface water during the rainy season. The consequences are similar to those observed in Nouakchott (deterioration of houses, health issues), although to a lesser extent. Another comparison can be made with the region of Oued-Souf in Algeria (e.g., Khechana and Derradji, 2012), where the intense exploitation of deeper aquifers for drinking water and for the expansion of irrigation has brought huge amounts of water into an area with no natural drainage. The lack of control by authorities for decades has turned the oasis into a wetland in the middle of the Sahara Desert, with palm trees dying because of excess water, and accompanying sanitary and town planning problems. With different processes, but the same consequences, the case of Jeddah in Saudi Arabia is also interesting (Alsefry and Sen, 2006). The extensive distribution of desalinated water and the return of waste waters through septic pits have resulted in a rise in the groundwater level. Compared with these different examples, the Nouakchott case is aggravated by its flat topography and the presence of large areas below sea level and a very fragile coastal shore bar. In some districts, the permanent presence of water and the risk of flooding have become more problematic than the provision of drinking water, which is paradoxical in an arid region with no sources of freshwater. Economic losses linked with flooding connected with the rise in groundwater have often been neglected, despite their importance in many cities like Nouakchott (Kreibich and Thieken, 2008).
The case of Nouakchott shows how quickly the socio-environmental system can change due to complex interactions between its many components. Up to now, observations in Nouakchott are not sufficiently dense and sufficiently representative to enable a thorough understanding of all main processes at work. Future changes and adaptation measures cannot be anticipated while the city is constantly changing. The double threat of the rising levels of seawater and groundwater questions the future of the capital. Different solutions have already been proposed, including the transfer of the city further inland. In all cases, huge investments will be required, whose relevance depends to a great extent on information that is still lacking. As is often the case, the small financial costs of the needed elementary observations contrast with the future expenses of infrastructure and their social consequences.
Appendix A Supplementary data
Supplementary Fig. I ESM1. Vertical profiles of electrical conductivity (EC) of groundwater in PZ1AXE4, PZ2AXE4, PZ3AXE4 and PZ4AXE4 wells.