1 Introduction
The 2-Ga-old West African Craton (WAC) is surrounded by deformed margins of different types and ages (Fig. 1). Limiting our study to the northern half of the craton, we find to the east the Pan-African Trans-Saharan collisional belt, formed around 620–600 Ma (Bosch et al., 2016; Caby, 2003). To the west, the Pan-African units are incorporated in the Variscan Mauritanide Belt, built around 300 Ma during the Pangean collision (Bea et al., 2016; Gärtner et al., 2014; Le Goff et al., 2001; Montero et al., 2017; Villeneuve et al., 2006) and extending northward up to latitude 28°N. This poly-orogenic border has been partly superimposed by the structures of the West African passive margin, formed basically during the Triassic–Liassic breakup of Pangea at ca. 190 Ma (Labails et al., 2010).

The northwestern limits of WAC and WAC-related Variscan terranes. A. Atlantic margin geomorphology and salt basins, after Biari et al. (2017), modified. Salt basin from Tarfaya to Dakhla, after Davison and Dailly (2010). Main geological boundaries around the WAC after Michard et al. (2010). B. Geotectonic map of the northwestern Africa–Iberia contact zone, showing the relationships of the Atlantic margin with the Azores–Gibraltar–West Mediterranean transform zone. Background map after Mascle and Mascle (2012). Triassic evaporite basins in pink. Offshore Triassic basin after Hafid et al. (2008).
The northern border of the WAC is currently defined by the South Atlas Fault (SAF, Fig. 1), i.e. the southern limit of the High Atlas intracontinental Alpine belt at some distance north of the Pan-African suture zone of the central Anti-Atlas (Ennih and Liégeois, 2001, 2008; Frizon de Lamotte et al., 2008). However, the SAF is mostly inherited from the Variscan South Meseta Fault (SMF; Michard et al., 2010), which limits the Meseta orogen from its Anti-Atlas foreland. A Paleoproterozoic and Neoproterozoic crust of Gondwanan affinity has been recently recognized in the Meseta domain (El Houicha et al., 2018; Letsch et al., 2017; Ouabid et al., 2017; Pereira et al., 2015). The Meseta Paleoproterozoic crust was rifted off the WAC before colliding against it during the Neoproterozoic “WAC-Cadomian” event of the Pan-African cycle (Hefferan et al., 2014). During the Paleozoic, the Meseta domain formed the distal passive margin of northwestern Gondwana before being amalgamated along the WAC Anti-Atlas border during the Variscan collision (Hoepffner et al., 2017; Michard et al., 2010). Thus, the most distal margin of the WAC-related terranes corresponds to the northern limit of the Atlas–Meseta domain beneath the thrust units of the ENE-trending Rif–Tell Alpine orogen.
The present paper aims at defining the east-trending limit of northwestern Africa inside the Rif domain and to recognize its connection with the northeast-trending Atlantic margin. The Moroccan Atlantic margin is currently described as preserving its overall northeast trend up to latitude 35°N (Biari et al., 2017; Hafid et al., 2008; Klingelhoefer et al., 2016), where it disappears beneath the accretionary prism of the Gulf of Cadiz (Crutchley et al., 2011; Gutscher et al., 2009). The implicit suggestion is that the Moroccan Atlantic margin should be crosscut there by the Newfoundland–Azores–Gibraltar fracture zone (Olivet et al., 1984; Sallarès et al., 2011), which broadens and coincides farther to the east with the Tell–Rif orogen (Galindo-Zaldívar et al., 2003; Meghraoui and Pondrelli, 2012). In contrast, we argue in the following that the Liassic Atlantic margin curved eastward at about latitude 35°N, entered the future Rif domain and connected there with the ENE-striking North African Transform (NAT) fault south of the Alpine Tethys domain (Frizon de Lamotte et al., 2011; Lemoine et al., 1987). This argument is based on a new, robust U–Pb zircon age obtained from an oceanic gabbro massif that crops out in the External Rif belt. This massif belongs to the so-called “Mesorif Suture Zone” (MSZ; Fig. 2; (Benzaggagh et al., 2014; Michard et al., 2007, 2014). Based on the new radiometric date, we propose the working hypothesis that rock material from the Atlantic margin could in fact constitutes most of, if not all, the oceanic elements of the Mesorif suture tectonic lineament. Moreover, we emphasize that the Kimmeridgian–Berriasian tholeiites and carbonates breccias described in the Mesorif (Ben Yaïch et al., 1989; Benzaggagh, 2011; Michard et al., 2007, 2014) define a second lineament, here labeled the “Mesorif–Basalts–Breccias” lineament, which could represent a segment of the Jurassic NAT. Therefore, transported elements of the Central Atlantic-Alpine Tethys connection zone would be exposed in the External Rif domain.

A. Location of the Rif mountain belt within the Maghrebide Belt (Durand-Delga, 1980). B. Simplified geological map of the Rif Belt after Suter (1980b) and Benzaggagh et al. (2014), modified. Miocene directions of transport after Frizon de Lamotte (1987), Chalouan et al. (2006, 2014), and Poujol et al. (2017). MBB: Mesorif Basalts-Breccias lineament; MSZ: Mesorif Suture Zone.
2 Geological setting
The Rif mountain belt extends from north to south over a maximum width of ∼150 km between the Mediterranean coast and the Atlas–Meseta domain, which forms its autochthonous foreland (Fig. 2; Chalouan et al., 2008, and references therein). The Rif Mountains belong to the Maghrebide belt, which extends to the east up to the Algerian Kabylies (Kabylias), Peloritani Mountains (NE Sicily), and Calabria (Durand-Delga, 1980). Every Maghrebide segment and the Rif as such consist of imbricated nappes with metamorphic Internal Zones forming the backstop of the tectonic prism. The Rif Belt shares its Internal Zones with the Betic Cordilleras of southern Spain. These common internal zones are labeled the Alboran Domain, which is thought to have been rifted off eastern Iberia together with the other Maghrebides internal terranes (“AlKaPeCa”; see Fig. 5 below) during the Late Eocene–Oligocene northwestward subduction of the Alpine Tethys lithosphere (e.g., Frizon de Lamotte et al., 2000, 2011; van Hinsbergen et al., 2014, and references therein). The Alboran Domain units (from bottom to top, the Sebtides, Ghomarides, and Dorsale calcaire units) are thrust over the Maghrebian Flyschs nappes, which consist dominantly of Lower Cretaceous-Early Miocene turbiditic, deep-water deposits (El Talibi et al., 2014; Guerrera and Martín-Martín, 2014; Zaghloul et al., 2007). The Maghrebian Flyschs have been interpreted as the detached infilling of the Liguro-Maghrebian Tethys (Durand-Delga et al., 2000, and references therein), although they likely onlapped the most distal Dorsale margin during the Middle Jurassic (Chalouan et al., 2011, p. 36; Olivier et al., 1996). They are associated with Upper Jurassic ophiolites and high-pressure/low-temperature metamorphism in Calabria. Ophiolites are also known south of Lesser Kabylia (Petite Kabylie, Algeria) associated with the Flyschs (Bouillin et al., 1997). In the Rif Mountains, only tholeiitic pillow basalts are found as tectonic slivers associated with the Flyschs nappes south of the Bokoya Internal units (Durand-Delga et al., 2000). These basalts are associated with radiolarites and micrites paleontologically dated from the Middle–Late Jurassic. The Flyschs nappes overlie in turn the most internal units of the External prism, namely the Intrarif (Ketama and Tanger units). The root zone of the Maghrebian Flyschs between the Alboran Domain units and the Intrarif is considered to be the Tethyan suture.
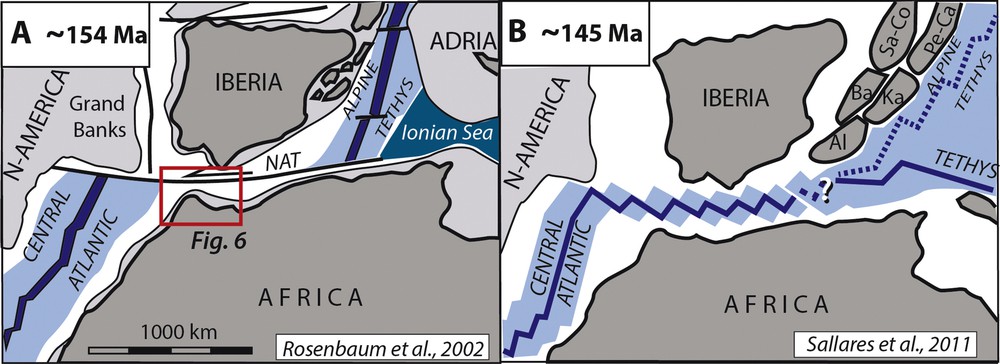
Two published reconstructions of the connection between Central Atlantic and Tethys oceans during the Late Jurassic. A. Classical view involving a sinistral North African transform zone (NAT), after Rosenbaum et al. (2002). B. Alternative reconstruction involving a narrow, segmented oceanic corridor between Africa and Iberia, after Sallarès et al. (2011). In both proposals, the Central Atlantic northeast-striking ridge is sharply interrupted by the east-trending transform or oceanic corridor.
The External Zones have been described for long as duplex-type imbricated units (Ketama and Tanger–Loukkos Intrarif units; Mesorif and Prerif units) and gravity nappes detached from the imbricated ones (Ouezzane, Aknoul, Tsoul), altogether derived from the African passive margin (Chalouan et al., 2008; Favre, 1992; Favre et al., 1991; Frizon de Lamotte, 1985; Leblanc, 1979; Suter, 1980a, 1980b; Zaghloul et al., 2005). A balanced cross-section of the tectonic stack of basement and cover units of the External Zone has been ultimately presented by Frizon de Lamotte et al. (2017).
In the eastern Rif, the Intrarif Ketama unit overlies the Beni Malek serpentinite massif, which exhibits a thin calcareous cover including detrital clasts of serpentinite and spinel. This remarkable massif was interpreted as derived from an exhumed mantle segment at the foot of the (hyper-extended) North-African margin (Michard et al., 1992, 2007). The Beni Malek massif and its hidden continuation at shallow depth (Elazzab et al., 1997) are the easternmost elements of what has been described as the “Mesorif Suture Zone”, which extends westward up to the Taounate region of central Rif and includes the Bou Adel gabbro here dated (Benzaggagh et al., 2014; Michard et al., 2014). The Mesorif suture zone consists of a string of kilometer-size slivers of oceanic crustal rocks with thin oceanic sedimentary cover rocks pinched between the Intrarif and Mesorif domains. The Beni Malek massif displays serpentinized spinel lherzolite including occasional pyroxenite layers. The other massifs display varied gabbro facies (cumulate troctolite, ferrogabbro) with E-MORB tholeiitic affinities crosscut by plagiogranite/trondhjemite veins or pockets. Altered basaltic flows and breccias including fragmented variolitic pillow lavas occur at Bou Adel and next to the Beni Malek serpentinites (Skifat metabasites). The sedimentary sequences that overlie these slivers are characterized by gabbroic/basaltic breccias, marbles with ophiolitic clasts, and locally radiolarites (Benzaggagh et al., 2014). We attempted to obtain a paleontological age from radiolarite samples, but the radiolarian fossils were poorly preserved and the age remained indeterminate (M. Chiari, Florence University, Italy, pers. comm.).
3 Dating the Bou Adel gabbro massif
3.1 Sampling and method
The Bou Adel gabbro massif (Fig. 3A) consists of cumulate troctolite and ferrogabbro crosscut in several places by veins, sills or pockets of plagiogranite/trondhjemite (Benzaggagh et al., 2014). Sample BA07 here dated has been collected in a several-meter-thick trondhjemite pocket cropping out along the Oued Azrou stream (Fig. 3B) 300 m to the southeast of the swimming pool of the Bou Adel touristic site (coordinates 34°32’2.85”N, 4°30’12.15”W). In thin section, the sampled rock shows large euhedral plagioclase crystals with minor interstitial quartz; it strictly compares with the trondhjemite illustrated by Benzaggagh et al. (2014) in their fig. 10C.

A. Location of the dated sample (red star) in the Bou Adel massif (schematic geologic map from Benzaggagh et al., 2014, after the Geological map of Morocco, scale 1:50,000). B. Aspect of the sampled trondhjemite outcrop. C. Cathodoluminescence images of the analyzed zircon grains. D. Concordia age of the BA07 trondhjemite sample in a Wetherill diagram (analyses corrected from common lead).
Zircons were separated from 1 kg of sample using standard heavy liquid and magnetic separation techniques. Seven grains were selected and handpicked to be mounted in an epoxy resin and then polished. Cathodoluminescence (CL) images (Fig. 3C) were obtained by Scanning Electron Microscopy (SEM) to reveal their internal structures. The selected zircon grains are generally broken and range from 50 to 100 μm in size. Only grain #1 is well faceted, prismatic, and pyramid-topped, and displays a core–rim structure.
In situ U–Pb isotope analyses of zircon grains were performed using an ICP-MS ELEMENT XR spectrometer coupled with a CETAC EXCITE 193-nm laser at the GEMOC laboratory facilities at Orsay (University of Paris-Sud). The zircon mount was located in a two-volume cell, with helium as the carrier gas. The laser was operated at a repetition rate of 8 Hz, with an ablation spot size of 40 μm and a fluence of 3.5 J·cm−2. Calibration for zircon analyses was carried out using the geostandard 91500 zircon (Wiedenbeck et al., 1995), and quality control was achieved using the Plésovice standard (338 ± 1 Ma, Slama et al., 2008).
The sensitivity of the machine permits recording 204Pb to check for possible lead contamination of the zircon grains. As the 204 mass could be influenced by the presence of 204Hg, the 202Hg (only candidate for this mass) was recorded in order to correct this interference. Then, the corrected mass 204 supposed to only represent common lead was used to correct the sample's analyses. The percentage of common lead revealed by the 204Pb measurement in each analysis is given in Table SM 1. Five analyses among eleven were shown to be weakly contaminated by common lead (spots 3, 4, 7, 8 and 11) with f204Pb < 0.4%. Only those five analyses were used to calculate a concordant age. Data reduction was carried out with the GLITTER software package (van Achterbergh et al., 2001), but the common lead correction was performed with an in-house built program. The results are presented in Fig. 3D with common lead corrected data and generated using the ISOPLOT/EX (v. 3) software by Ludwig (2003).
3.2 Results
Eleven ablations were performed on seven zircon grains (Table SM 1). Analyses 1 and 2 were performed on inherited zircon grain #1, whose significance will be discussed further. These analyses were not used in the set of data to calculate the age of the sample. Analysis 6 was performed on a chemically disturbed zircon grain #4 (see ESM image), and analyses 5 and 9 were performed on the heterogeneous part of zircon grains. Spot 10 has been performed on an apparently fairly homogeneous zircon, but some evidence of cracks on grain #6 could have disturbed the corresponding measurement. Those four analyses, performed on heterogeneous areas, display too much common lead contamination to be effectively corrected; they are plotted as dotted ellipses in Fig. 3D. The five other analyses (spot 3, 4, 7, 8 and 11) were performed on apparently homogeneous areas showing a similar luminescence on each grain. Those five analyses are less contaminated with common lead, and the corrected analyses provide a concordant age of 190 ± 2 Ma on the Wetherill diagram (Fig. 3D, full line). The fit of the analyses vs. the Concordia diagram provides robust constraint on the age of crystallization of these zircon grains.
Analyses 1 and 2 performed on grain #1, i.e. the inherited, well-faceted prismatic and pyramid-topped zircon grain revealed 204Pb content and could have suffered lead loss. On the Wetherill diagram of Fig. 4, these two analyses after 204Pb correction plot onto a straight line passing through the origin (75 ± 60Ma) without any constraint (for comparison, the analyses without common lead correction are plotted as dotted gray ellipse). This could reveal that the lead-loss process was recent. Accordingly, this grain cannot be dated with precision, but an age of crystallization at about 2 Ga is likely.

Plot of analyses 1 and 2 on grain #1 in the Wetherill diagram. The straight blue line was poorly defined by the two common lead corrected analyses going through close to the origin without being forced. The same analyses without lead correction plot as the two dotted gray ellipses.
4 Discussion
4.1 The previous interpretation of the Mesorif suture zone might be abandoned
In line with the early interpretation of the Beni Malek serpentinites as exhumed mantle from the Ocean–Continent Transition (OCT) of the Late Jurassic North-African margin (Michard et al., 1992), the curved alignment of gabbros massifs with similar oceanic sedimentary cover that forms the main part of the Mesorif suture zone (Fig. 2) has been regarded as the trace of the same OCT, displaced by the Miocene thrust tectonics (Benzaggagh et al., 2014; Michard et al., 2014). In other words, the gabbro slivers of the Mesorif suture zone were regarded as emplaced in the framework of the Jurassic opening of the Maghrebian–Ligurian Tethys, which is known to be basically Callovian–Oxfordian to Tithonian in age (ca. 166–150 Ma; Lemoine et al., 1987; Li et al., 2013) although the earliest isotopic dates are Bajocian (ca. 170 Ma; Bill et al., 2001). This hypothesis was consistent with a K–Ar whole-rock age of 166 ± 3 Ma (Bathonian–Callovian) obtained on the Bou Adel gabbro by J.-M. Cantagrel at the Clermont-Ferrand Laboratory, and published by Asebriy (1994, p. 51). However, the limits of the K–Ar method when applied to low-grade metamorphic gabbros such as those of the Mesorif suture zone are well known (Ducrot and Lancelot, 1977). For this reason, we decided to obtain a robust, U–Pb zircon age from the Bou Adel trondhjemite veins that were emplaced by the end of the gabbro crystallization. Now, the Liassic age that we obtained contradicts our previous interpretation.
4.2 The Mesorif Basalts–Breccias (MBB) lineament and the new proposal for the MSZ
In the External Rif belt, an arcuate alignment of basalts and carbonate breccias, i.e. the Mesorif Basalts–Breccias lineament, almost parallels the MSZ in a more external position (Fig. 2). Along this lineament, the Kimmeridgian–Tithonian/Berriasian sequences of the external Mesorif–internal Prerif units are typified by tholeiitic lava flows and/or calcareous breccias, including tholeiitic basalt, dolerite, and fine-grained gabbro elements (Benzaggagh, 2011; Benzaggagh et al., 2014). This mix of carbonate and magmatic formations occurs everywhere on top of a kilometer-thick Callovian–Oxfordian turbidite formation (the “ferrysch”) that seals the Lower–Middle Jurassic tilted-block and half-graben structures of the North-African paleomargin (Suter, 1980a; Favre, 1992). Most of the elements of the breccias are shallow water carbonates reworked into deep waters by mass flows and turbidity currents at the expense of a more external carbonate platform. The oldest of the associated magmatic rocks are pyroclastic layers emplaced by the end of the Oxfordian, and the latest are disrupted basalt flows included in the Upper Tithonian–Berriasian breccias. The chaotic breccias also contain pebbles or boulders of ferrysch-like sandstones, whose abundance and size increase upward. Large olistoliths of typical ferrysch facies occur locally in the Berriasian sequence (Benzaggagh et al., 2014).
The tectonic significance of the reported magmatic–sedimentary association has been underestimated up to now, although in their study of the western Mesorif (Ouezzane area), Ben Yaïch et al. (1989) noticed that it would indicate an abrupt thinning of the North-African margin. We think it necessary to interpret the association at the scale of the entire Mesorif considering two significant observations, (i) the parallelism of the Basalts–Breccias lineament with the Mesorif suture, and (ii) the slightly diverging thrust transport directions of the External Rif tectonic units during the Miocene (Fig. 2). These directions are typically oriented to the southwest in the eastern and central Rif, whereas they progressively turn to the WSW and the west in the western Rif (Chalouan et al., 2006, 2014; Frizon de Lamotte, 1985, 1987; Poujol et al., 2017).
Keeping in mind the above remarks, and based on the 190 ± 2 Ma age of the Bou Adel gabbro, we may explore a new avenue to explain both the Mesorif suture and Basalts–Breccias lineaments in the framework of the Central Atlantic–Alpine Tethys relationships. The connection between these two oceanic domains implies classically the activity of a broadly latitudinal North-African Transform (NAT; Fig. 5A) during the Jurassic–Early Cretaceous times (Lemoine et al., 1987; Rosenbaum et al., 2002) or that of an equivalent, east–west system of densely segmented oceanic ridges (Fig. 5B; Frizon de Lamotte et al., 2011; Sallarès et al., 2011). Schettino and Turco (2011) combine a North African transform-equivalent labeled the “Gibraltar Fault” with a narrow, segmented oceanic corridor in their geodynamic reconstruction for the Late Jurassic period. However, the opening of the Central Atlantic began at ca. 190 Ma (Sinemurian–Pliensbachian; Labails et al., 2010), whereas the Alpine Tethys opened not before 170 Ma (Bajocian) at the earliest (Bill et al., 2001; Lagabrielle and Lemoine, 1997). This diachronism, seldom considered up to now, makes less simple the interpretation of the crustal movements at the Rifian corner of North Africa. Moreover, in the studied area, the strike and kinematics of the major faults depend on the movement of the Iberian plate with respect to Africa and Europe, which is still discussed (Barnett-Moore et al., 2016; Vissers and Meijer, 2012). Plate tectonic reconstructions indicate that the North African transform was a sinistral transform zone during the Late Jurassic, but that it was dextral during the Aptian–Albian, due to the anticlockwise rotation of Iberia (Vissers and Meijer, 2012, and references therein).
We suggest that the magmatic-sedimentary dramatic events recorded along the MBB lineament during the Late Jurassic–Berriasian are linked to the Late Jurassic activity of the North African transform, either along the main transform zone or along some synthetic fault. The interpretation of the broadly parallel Mesorif suture zone lineament is more problematic in the present state of knowledge. One of the gabbroic bodies (Bou Adel) has been dated from the Early/Middle Liassic, but its oceanic-type cover has not been dated yet, nor the low-grade metamorphism observed in these exotic bodies. The association of basaltic breccia, radiolarites, marbles and ophiolitic sands offers a clear Ligurian affinity, and thus can be hypothetically ascribed to the Late Jurassic. At this step of our interpretation, we may propose two alternative hypotheses: (i) the Early–Middle Liassic Bou Adel gabbro and its equivalents would have been exhumed at the northern tip of a NNE-trending Atlantic OCT, next to the distal Continent-Ocean Boundary (COB; see Klingelhoefer et al., 2016), then transported along a dextral shear zone during the Middle Jurassic before being overlain by their sedimentary covers, or (ii) the Mesorif suture gabbros would underline the eastward curvature of the Atlantic margin, and they were exhumed during the Middle Jurassic in the vicinity of the North African transform. The first hypothesis cannot be maintained, as no major dextral movement is documented there during the Middle Jurassic by plate tectonic reconstructions. Therefore, we favor the hypothesis of a curvature of the Atlantic margin that would have surrounded the NW Meseta promontory (Sehoul Block; Michard et al., 2010) to connect eastward with the South Tethyan margin (Fig. 6).
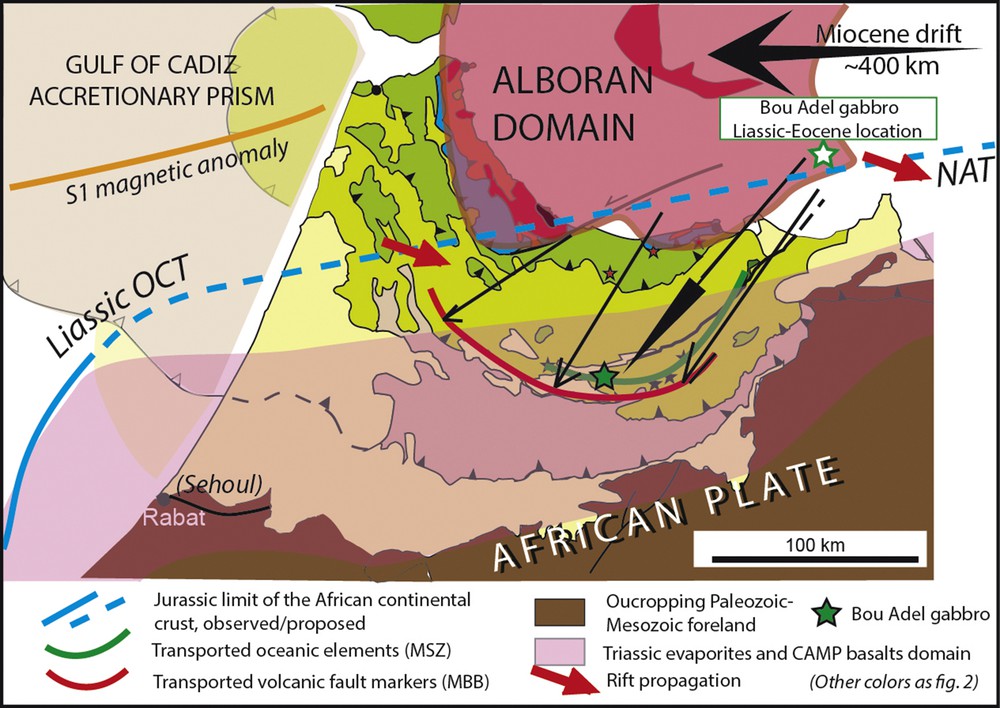
New proposal for the Central Atlantic-Alpine Tethys connection involving an eastward curvature of the Atlantic margin around the Moroccan Meseta promontory (this work). This proposal is based on the Liassic age of the Bou Adel gabbro of the Mesorif Suture Zone (MSZ). The curved segment of the Liassic Atlantic margin would have connected due to rift propagation with the Middle Jurassic North African Transform (NAT) whose transported elements form the Mesorif Basalt-Breccia (MBB) lineament. During the Miocene, the Bou Adel massif would have reached its present-day location due to the shortening and SW-ward transport of the External Rif accretionary prism.
The latter figure is intended to make visible the displacements that the varied tectonic units of the Rif suffered with respect to the Atlas–Meseta foreland. During the Miocene, the movement along the North African transform was sinistral, as this shear zone allowed the westward displacement of the Alboran Domain due to the Gibraltar slab retreat (Jolivet and Faccenna, 2000; van Hinsbergen et al., 2014). The Alboran Domain displacement along the Miocene North African transform is estimated by the latter authors in the range of 400–500 km. The elements belonging to the southern, North African compartment of the Miocene transform were also affected by a westward simple shear deformation (demonstrated in the Temsamane massif of the Mesorif; Frizon de Lamotte, 1985, 1987), but they were certainly less displaced than the Alboran Domain itself. At the same time, Africa–Iberia convergence resulted in the building of the External Rif accretionary prism and its tectonic transport toward the foreland. As a conservative hypothesis, we may estimate a southwestward tectonic transport of about 100 km for the Bou Adel-type massifs of the Mesozoic suture. Thus, “unfolding” the External Rif tectonic prism allows us to restore the plausible initial location of the Mesozoic Basalts–Breccias and Suture Zone lineaments along the Jurassic North African transform (Fig. 6). The 30 Ma delay between the emplacement of the Bou Adel gabbro in the MSZ and the MBB fault zone activity would correspond to the propagation of the Central Atlantic rift both eastward (toward the Alpine Tethys) and southward (encroaching the proximal African margin). The Central Atlantic rift would have propagated eastward over about 2000 km in about 20–30 Ma. The rift propagation would have thus occurred at about 10 cm/yr, which is a propagation rate consistent with rates found for other rifts and fault zones worldwide (e.g., Hubert-Ferrari et al., 2003; Manighetti et al., 1997, and references therein).
Two observations support our interpretation. First, the Triassic evaporitic basins that record the earliest rifting of Pangea along the Atlantic margin, culminating with the CAMP magmatism at 201–195 Ma (Leleu et al., 2016; Verati et al., 2007), also surround the NW Moroccan Meseta promontory and are well developed in the Prerif–Mesorif domain itself. Second, the “Slope anomaly” S’ (i.e. the oldest magnetic anomaly on the African side, also labeled “West Atlantic Margin Anomaly”) adopts an ENE trend west of Tangier, beneath the accretionary prism of the Gulf of Cadiz (Klingelhoefer et al., 2016). Martínez-Loriente et al. (2014) also draw a curvature of the Central Atlantic ridge in their reconstruction of the 180 Ma stage (their fig. 10), but their subsequent 155 Ma reconstruction shows a rectilinear Central Atlantic ridge sharply crosscut by the Gibraltar Fault. On the other hand, the presence in the Bou Adel trondhjemite of a Proterozoic (ca. 2 Ga) xenocrystic zircon reveals the existence of an ancient continental crust, possibly inherited from the WAC, beneath the locus of emplacement of the trondhjemite as it is the case for the trondhjemite from the Balagne nappe in Corsica (Rossi et al., 2002) or in the Mauritius trachyte (Ashwal et al., 2017). This is consistent with the MSZ gabbro emplacement in the OCT domain along the northern Meseta. Indeed, the Rabat granite of the Sehoul Block yielded 2-Ga-old inherited zircon grains (Tahiri et al., 2010), and farther to the south, the Meseta Coastal Block displays a shallow, and by place outcropping Paleoproterozoic basement (Letsch et al., 2017; Pereira et al., 2015).
5 Conclusion
At the northwestern corner of the African plate, the ultimate limit of the WAC and WAC-related domains corresponds nowadays to the diffuse Iberia–Africa plate boundary that extends from the Gulf of Cadiz to the Rif–Tell orogen. This plate boundary is inherited from the sinistral Miocene transform zone, active during the westward displacement of the Alboran Domain. The Miocene transform is itself inherited from the Jurassic transform zone, active during the Pangea breakup and the diachronic opening of the Central Atlantic and Alpine Tethys oceans. The present work suggests that a deformed trace of the Jurassic transform or of some synthetic fault can be found in the Mesorif Basalts–Breccias lineament. Our work also suggests that the arcuate Mesorif Suture Zone, adjacent to the MBB, would represent the ENE-striking continuation of the Atlantic margin.
This preliminary hypothesis is based on the U–Pb zircon dating at 190 ± 2 Ma of one of the gabbro massifs of the Mesorif zone, which appears to be coeval with the early opening of the Central Atlantic. The chaotic formations of the nearby Basalts–Breccias lineament, mainly Kimmeridgian–Tithonian in age, would delineate the border of the North-African margin along the Jurassic transform zone. If correct, this new interpretation can provide the impetus for performing additional field and laboratory studies of the exhumed samples of the up-to-now conceptual Jurassic transform zone.
Acknowledgments
Logistic support for field studies was granted by the Ministry of Energy and Mines, Water and Environment, Rabat. We warmly thank Prof. Marco Chiari for his kind tentative to determine radiolarian remains in four of our samples, collected with the help of our friends Faouziya Haissen and Najib B. Zaghloul. We warmly thank our colleagues Philippe Olivier and Marc-André Gutscher, who achieved thorough and constructive peer reviews of the submitted manuscript. We also thank Isabelle Manighetti who kindly organized the reviewing process and suggested useful improvements to our manuscript.