1 Introduction
Ozone-depleting substances (ODSs) under the Montreal Protocol on Substances that Deplete the Ozone Layer consist of long-lived (atmospheric lifetime > 0.5 years) chlorofluorocarbons (CFCs), hydrochlorofluorocarbons (HCFCs), chlorinated solvents (carbon tetrachloride, methyl chloroform), halons and methyl bromide. CFCs were developed in the 1930s as new refrigerants to replace existing toxic compounds (e.g., ammonia, sulfur dioxide) based on their excellent refrigerant capabilities and low toxicity, and began to be used by the refrigeration/air conditioning industries in accelerating amounts in the 1950s. Other uses soon followed (i.e. foam blowing and propellants in spray cans) and these applications soon dominated the CFC consumption. Earlier, the important feedstock chemical carbon tetrachloride (CCl4) found wide-spread use as a solvent and fire retardant, and later the brominated halons were introduced as very successful, non-toxic, fire extinguishing chemicals. Furthermore, methyl bromide (CH3Br) was used as a very effective soil and structural fumigant and methyl chloroform (CH3CCl3) was introduced as a degreasing solvent.
For CCl4, its dispersive applications were restricted because of concerns about carcinogenicity even before the negative ozone impact of chlorine radicals from long-lived ODSs was hypothesized by Molina and Rowland (1974). This ground-breaking publication initiated the first-ever restrictions on ODS consumption, where, for example, the use of CFC-11 (CCl3F) was banned in spray cans in Sweden and the USA in 1978. This proactive policy was well before further international restrictions were bolstered by the Farman et al. (1985) detection of significant ozone depletion during the Antarctic spring (the “ozone hole”) and the suggestion by Solomon et al. (1986) that chlorine released from ODSs was activated under certain conditions in the polar stratosphere. Coupled with the results from ground-based and airborne investigations of the Antarctic ozone hole, the causal role of ODSs in the severe seasonal thinning of the stratospheric ozone layer over Antarctica was established, as summarized in Hartmann and Watson (1988) and in Solomon (1989).
After the adoption of the Montreal Protocol on Substances that Deplete the Ozone Layer in 1987, long-term atmospheric measurements became even more essential for verifying the effect of the gradual phase-out of the production of the strongest ODSs for emissive uses and their subsequent replacement by HCFCs (hydrochlorofluorocarbons) and HFCs (hydrofluorocarbons). Currently, HFOs (hydrofluoroolefins), unsaturated HFCs, are being introduced into the market, responding to concerns about the high global warming potential of HFCs, and also in response to the recent Kigali Amendment to the Montreal Protocol, which restricts production and consumption of HFCs. This will ensure the on-going success of the Montreal Protocol in reducing not only ozone depletion but also climate change.
In addition to chlorine radicals, bromine radicals from long-lived bromocarbons were determined to contribute to the stratospheric ozone depletion (McElroy et al., 1986; Wofsy et al., 1975). Therefore, long-lived brominated halons were also included in the provisions of the initial Montreal Protocol and the time frame for control has been approximately parallel to that of the chlorinated ODSs. In addition, methyl bromide (CH3Br), used for soil and structural fumigation as well as for fumigation in shipping, was only regulated later in this process, as its atmospheric lifetime is relatively short and acceptable alternative chemicals/processes were proving elusive. Nevertheless, its industrial production for dispersive applications is now also banned, with an exemption for fumigation in international shipping.
In this publication we start by recognising to the very first measurements of CFCs in the atmosphere. This is followed by a discussion of the results from the long-term global measurement networks over nearly four decades (1978–2016), using flask and in situ measurements and remote sensing techniques. This information is then used to derive global emissions of ODSs and their replacement compounds. Finally, current issues related to on-going lingering emissions of strong ODSs and newly detected ODSs are discussed.
2 Early measurements
In 1970, James Lovelock used a gas chromatograph fitted with an electron capture detector (GC-ECD) to make the first atmospheric in situ measurements of CFC-11 at Adrigole, Ireland (Lovelock, 1971). Lovelock (1972) presented 1971 measurement data from the same location, showing the elevated levels of CFC-11 in pollution events from the neighbouring United Kingdom (Fig. 1a). The first globally representative measurements of CFC-11 and CCl4 followed, which were obtained during a ship cruise in 1971–1972 from England to Antarctica (Lovelock et al., 1973). For CFC-11, a global average of approximately 50 ppt (parts-per-trillion as a mole fraction) was found together with a pronounced interhemispheric gradient (Fig. 1b), which was related to large anthropogenic sources in the Northern Hemisphere. Atmospheric mole fractions were calculated using the detector as an absolute coulometer (electrons captured = number of molecules detected) (Lovelock et al., 1971), as adequate calibration was not available at that time. Later, McCulloch et al. (2001) revised the global production numbers for CFC-11 and separated emissions into immediate releases from spray cans and delayed emissions from foams. The theoretical value of around 60 ppt at that time (Figure 3 in McCulloch et al., 2001) comes remarkably close to the number from atmospheric observations (Lovelock et al., 1973).
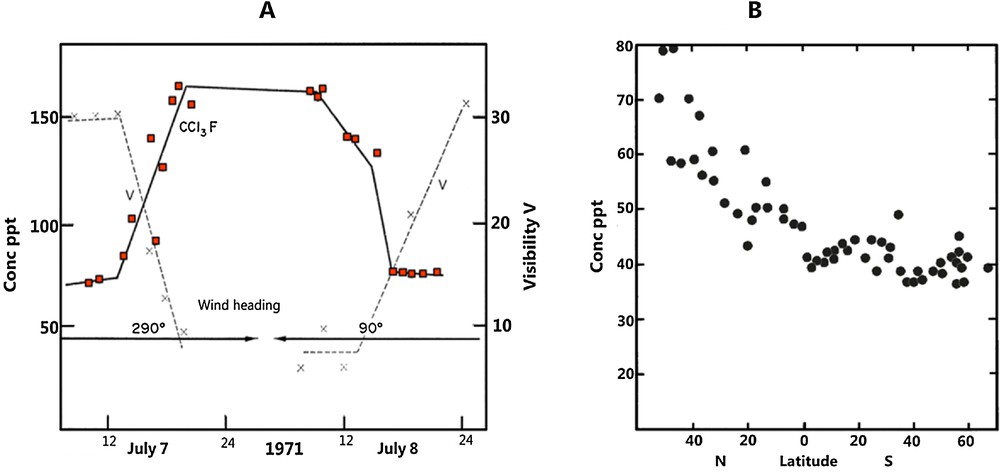
First atmospheric measurements of CFCs. A. Observations of CFC-11 at Adrigole (Ireland) in summer 1971, showing an inverse relationship with visibility (i.e. a measure for air pollution arriving at the site from the United Kingdom), adapted from Lovelock (1972). B. Latitudinal distribution of CFC-11 in surface air observed during the transect from England to Antarctica in 1971/72, adapted from Lovelock et al. (1973).
Furthermore, Lovelock et al. (1973) found a good agreement between total production of CFC-11 and its global atmospheric burden. Later, however, Fraser et al. (1983) were the first to suggest significant differences between CFC-11 emissions calculated by industry and from atmospheric data.
The earliest CFC measurements in the US were published in 1973–1974 by Su and Goldberg (1973) and Simmonds et al. (1974), at the same time when another measurement cruise covering the Southern Hemisphere was performed by Wilkniss et al. (1973). In addition, Lovelock and co-workers advanced measurements at Adrigole and other places world-wide (Lovelock, 1974; Pack et al., 1977).
The first in situ and flask measurements of CFC-11 in Australia were made at Aspendale in 1975 (Fraser et al., 1977), using a GC-ECD provided by Lovelock, and the first continuous in situ and flask measurements in the mid and polar latitudes of the Southern Hemisphere were reported by Fraser and Pearman (1978), with measurements commencing at Cape Grim (in situ), in the mid-troposphere (flask) over SE Australia and at Mawson, Antarctica (flask), in 1976.
At about the same time Zander (1975) reported the first unambiguous evidence of the transport of the CFCs up to the stratosphere by identifying the absorption of HF (hydrogen fluoride) in infrared balloon spectra recorded at 27.5 km altitude in September 1974 from Palestine, Texas, USA. This finding of the end-product of the photolysis of the CFCs, taking place in the stratosphere, was soon confirmed, using ground-based infrared spectra recorded at the Jungfraujoch station (Zander et al., 1977). Remote sensing observations of CFCs themselves were first reported from Kitt Peak National Observatory (KPNO), Tucson, Arizona (USA) in the early 1980s by Zander et al. (1983).
3 Development and current status of Global Measurement Networks
In the late 1970s the in situ and flask measurement activities evolved into two separate independent global measurements networks, operated by NOAA (US National Oceanic and Atmospheric Administration) and AGAGE (Advanced Global Atmospheric Gases Experiment; supported by the National Aeronautics and Space Administration, NASA). Both networks have developed their own calibration scales, which are now regularly compared (Hall et al., 2014), and cooperation is maintained at regular quality assurance and science meetings (biannual AGAGE Meetings; ESRL/GMD Annual Conferences).
NOAA started continuous global flask measurements at four sites in 1977. This developed into a globally representative network with a mixture of flask data and in situ measurements at a total of 14 sites by 2004 (Elkins et al., 1993) (Fig. 2A). The ALE project (Atmospheric Lifetime Experiment, supported by NASA, the U.S. National Science Foundation, and the Chemical Manufacturers Association) began in situ measurements in July 1978 also at four globally distributed sites (Prinn et al., 1983). ALE developed into GAGE and then AGAGE, with 11 current stations world-wide (including collaborative stations), where ODSs are analysed (Fig. 2B). All AGAGE stations use an identical pre-concentration device (Miller et al., 2008), which is connected to a gas chromatograph-mass spectrometer (GC-MS), to augment the earlier whole air injection gas chromatographic multi-channel instruments used at the original 5 ALE/GAGE/AGAGE stations (Prinn et al., 2000, 2018).
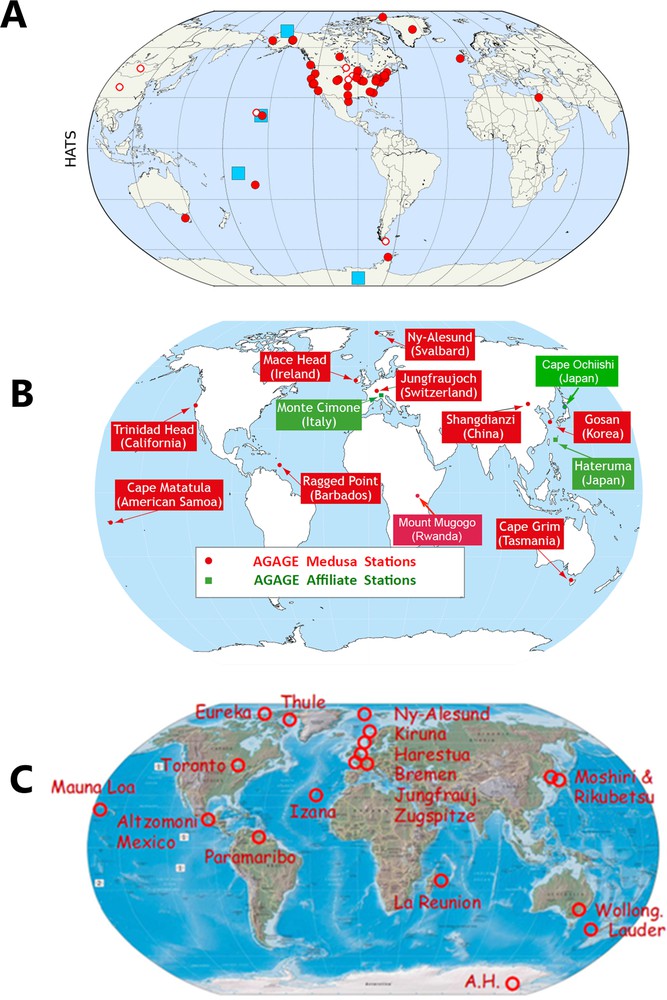
A. Sites where NOAA performs halocarbon measurements from flasks filled at Earths’ surface and from aircraft profiles (red filled circles, unfilled circles represent discontinued sites) and from on-site instrumentation. B. AGAGE and affiliated sites global measurement network (continuous, in situ). C. Remote sensing FTIR instruments affiliated to the global NDACC network. Time series of total column ozone and halogenated stratospheric reservoir chemical densities are available for these sites; for a few sites, ODSs are also retrieved.
As an example of the value of these long-term measurements for documenting the success of the Montreal Protocol, the CFC-11 data set from the Adrigole/Mace Head station is shown in Fig. 3. In the early part of the record, increasing mole fractions coincided with considerably elevated concentrations during pollution events, indicating substantial global and European emissions, respectively. Background mole fractions peaked in the 1990s as a result of a decline in global emissions. Similarly, the magnitude of pollution events began to decline in the late 1980s, indicating a decrease in European emissions.
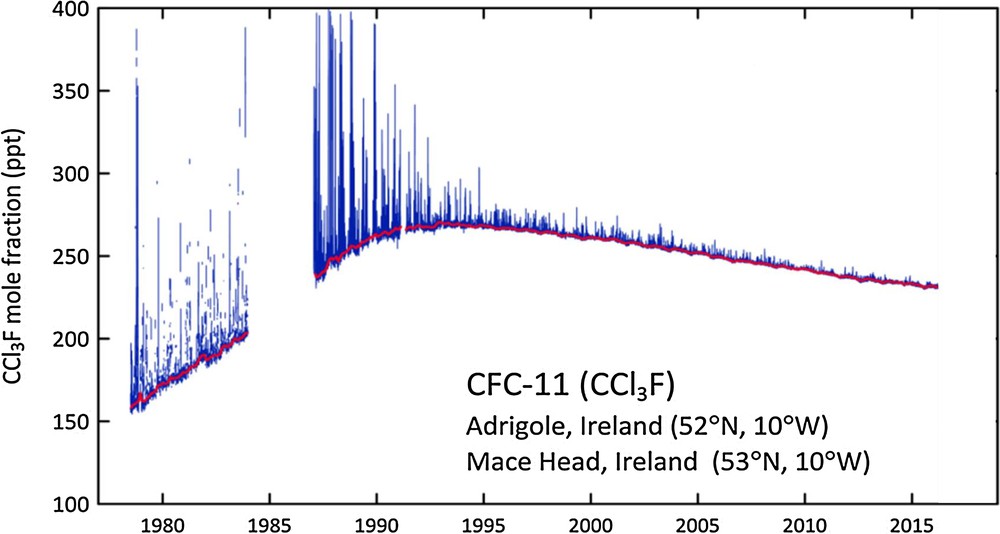
Mole fractions of CFC-11 at Adrigole and Mace Head (Ireland) measured by ALE/GAGE/AGAGE between 1978 and 2016. Concentrations measured under “background” conditions are indicated in red. The magnitude of pollution events decreased concurrently with the substance itself (AGAGE, 2017).
The instrumentation and capabilities of the NOAA and AGAGE networks have been used retrospectively to extend ODS and other halocarbon measurements back into times when analyses of these compounds were not yet possible. For this purpose, the Cape Grim (Tasmania) air archive has been resourced and maintained by CSIRO (Commonwealth Scientific and Industrial Research Organization) and the Bureau of Meteorology since 1978 and analysed by numerous laboratories worldwide. Furthermore, older records of halocarbon concentrations have also been derived from measurements using air trapped in firn using NOAA and AGAGE instrumentation (e.g., Butler et al., 1999; Sturrock et al., 2002). The large global networks of NOAA and AGAGE are further complemented by an additional global flask measurement network maintained by UCI (University of California at Irvine, USA).
In parallel to the in situ and flask measurements, column abundance measurements of halocarbons have been performed by Fourier transform infrared (FTIR) spectrometers in the global Network for Detection of Stratospheric Change (NDSC) since the mid-1980s, as documented for example in Zander et al. (2005). NDSC transitioned into the Network for Detection of Atmospheric Composition Change (NDACC, see De Mazière et al., 2018), with ground-based remote sensing measurements of ozone, halocarbons and many other compounds relevant not only for stratospheric ozone studies, but also for understanding the changing composition of the upper troposphere and stratosphere (Fig. 2C). An example of the excellent measurement records is shown in Fig. 4 with column abundances for CFC-11 (CCl3F), CFC-12 (CCl2F2), CCl4 and HCFC-22 (CHClF2) from Jungfraujoch, Switzerland. Consistent with the in situ tropospheric measurements, the column abundances of CFCs and CCl4 are declining, thereby demonstrating the positive influence of the Montreal Protocol throughout the global atmosphere.
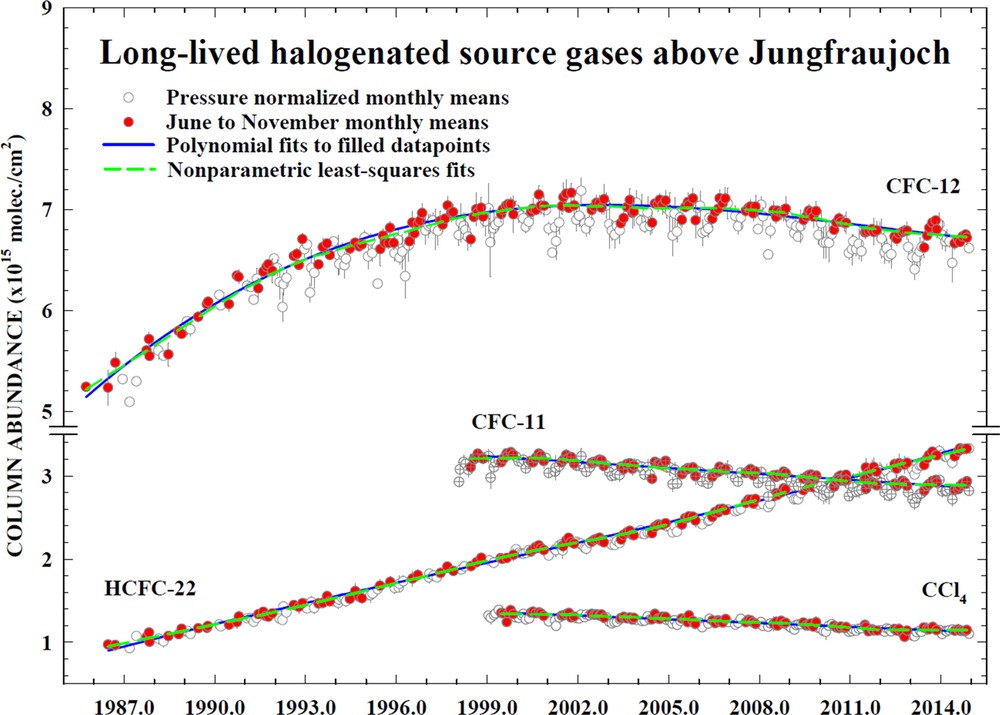
Time series of total column densities for CFC-12, CFC-11, HCFC-22 and CCl4, as measured at Jungfraujoch (Switzerland) by the FTIR remote-sensing technique. Note the discontinuity in the vertical scale. Updated from Zander et al. (2005) and Carpenter et al. (2014).
4 Measured Mole Fractions and Inferred Emissions
In this section the evolution of atmospheric concentrations for the most important ozone-depleting substances, measured by the NOAA and AGAGE networks, are discussed in relation to the legal framework of the Montreal Protocol. These data are then used to estimate global emissions using a global 12-box model (Cunnold et al., 1994; Rigby et al., 2013). Three groups of ODSs are identified for the discussion: (i) CFCs and long-lived chlorinated solvents, (ii) HCFCs and (iii) brominated species (halons and methyl bromide). For each category, the discussion concludes with highlighting current issues related to the Montreal Protocol.
4.1 Chlorofluorocarbons (CFCs) and Long-lived Chlorinated Solvents–the Original Substances Covered by the Montreal Protocol
In the Montreal Protocol production of CFCs for emissive usage was phased-out in 1996 for Non-A5 (industrialized) countries and in 2010 for A5 (developing) countries. In Fig. 5A the evolution of the measured mole fractions and inferred global emissions are shown for CFC-11 (CCl3F), CFC-12 (CCl2F2) and CFC-113 (C2Cl3F3) as well as for the long-lived chlorinated solvents CCl4 and methyl chloroform (CH3CCl3). These substances had the most widespread uses amongst the original ODSs and hence reached the highest mole fractions in the atmosphere (Carpenter et al., 2014). The highest abundance of all ODSs was reached by CFC-12, which was mostly used as a refrigerant, with peak mole fractions of more than 540 ppt around 2003. The next highest abundance was reached by CFC-11, which was used as a propellant in spray cans, as refrigerant and as foam blowing agent, with values of 260–270 ppt around 1994. Finally, CFC-113, which was primarily used as a solvent, peaked at 83 ppt around 1996.

Long-term evolution of globally averaged mole fractions and derived world-wide emissions from the NOAA (light colours) and AGAGE (dark colours) global networks and archived air samples. A. Long-lived chlorofluorocarbons (CFC-12, CFC-11, CFC-113) and the chlorinated solvents (CCl4 and CH3CCl3). B. Hydrochlorofluorocarbons (HCFC-22, HCFC-141b, HCFC-142b). C. Halons (H-1211, H-1301) and methyl bromide (CH3Br).
The differences in the timing of peak concentrations are due to a combination of substance-specific lifetimes and usages. CFC-11 was the first of the CFCs to achieve decreasing atmospheric levels due to the fact that its dominant emissive usage in spray cans was prohibited early in the process of globally restricting ODS production and consumption. However, delayed emissions from building materials associated with CFC-11 usage as a foam-blowing agent are on-going (although on a much smaller scale). For CFC-113, its dominant use as a solvent, with only small stored amounts, resulted in immediate atmospheric impacts being seen soon after production/consumption restrictions were imposed. Significant CFC-12 emissions resulted from leakages due to its use as a refrigerant (e.g., in air conditioners and refrigerators) but remaining emissions have declined considerably in the most recent years, as the amount of CFC-12 remaining in equipment becomes more and more exhausted.
Other CFCs (such as CFC-13 (CClF3), CFC-114 (C2Cl2F4), CFC-115 (C2ClF5)) were also used in industry and consumer products, although on much smaller scales. A recent review by Vollmer et al. (2018) assesses their behaviour in the global atmosphere and their small on-going emissions over the recent decades.
For the long-lived chlorinated solvents, production for emissive usage was scheduled to cease in 1996 for Non-A5 (industrialized) countries. In A5 countries, bans were introduced in 2010 and 2015 for CCl4 and CH3CCl3, respectively. CCl4 had a widespread use as solvent in metal cleaning and other industrial applications. Furthermore, it was used as a feedstock for the production of CFCs. The maximum mole fraction of ∼105 ppt appeared in the early 1990s. However, small, but non-negligible emissions are ongoing and are a matter of concern (see below). CH3CCl3 was also used in large quantities as a solvent and for metal cleaning purposes. With nearly 700 Gg/yr (kt/yr), CH3CCl3 had the highest emissions of all ODSs. However, as only short-term storage occurred for this compound, emissions fell drastically after 1990 and the maximum mole fraction of ∼130 ppt was reached shortly afterwards. As a consequence of its rather short atmospheric lifetime (5 years), atmospheric mole fractions decreased rapidly after regulation and, until recently, provided the largest contribution to the decline of chlorine in the stratosphere (WMO, 2014). However, in 2016 this influence has practically disappeared, with only 2 ppt of CH3CCl3 left in the atmosphere.
Current issues related to CFCs and long-lived chlorinated solvents: Although atmospheric concentrations and emissions of CFCs and chlorinated solvents have generally decreased as a consequence of the Montreal Protocol, remaining and even renewed emissions for some species are a point of concern. For CCl4, a long-term mismatch between the expected decrease of atmospheric concentrations and remaining small emissions could recently be partially resolved by adapting a revised, longer atmospheric lifetime and by the detection of new sources from the production of chloromethanes and chlorine (SPARC, 2016). There were, however, still 35 Gg/yr of unexplained CCl4 emissions globally between 2011 and 2014 (SPARC, 2016). In addition, Montzka et al. (2018) found a slower than expected decline in the global background concentrations of CFC-11 in recent years due in part to an increase in emissions. On the other hand, CFC-12, CFC-113 and CH3CCl3 continue to decline mostly as expected, although some small-scale emissions can still be detected on the regional scale (e.g., small but steady European and US emissions of CH3CCl3, as reported by Maione et al. (2014) and Rigby et al. (2017).
4.2 Hydrochlorofluorocarbons (HCFCs)–The original replacement compounds
HCFC-22 (CHClF2), HCFC-141b C2H3Cl2F) and HCFC-142b C2H3ClF2) are the three most widely used HCFCs (Fig. 5B). They were the initial replacement compounds for the restricted CFCs during the first phase of the Montreal Protocol. Before this period, only HCFC-22 had significant emissions of about 100 Gg/yr (from its use in stationary air conditioners). Whereas HCFC-142b had some small sources from foam blowing and refrigeration, HCFC-141b was practically non-existent in the atmosphere until its initial application in the 1990s for foam blowing and as a replacement for CFC-113.
Under the Montreal Protocol, restrictions for HCFCs were initiated by a freeze in production for non-emissive uses in 1996 for Non-A5 countries and in 2013 for A5 countries. A nearly complete ban of production and consumption for emissive uses will occur by 2020 for the Non-A5 countries and by 2030 for A5 countries, with only small exceptions for maintenance of existing equipment allowed for another decade.
HCFCs were already forbidden from usage in foam blowing in Non-A5 countries in 2003, noticeable by a short-term dip in otherwise increasing concentrations and global emissions at that time. Shortly afterwards, however, new production capacities in A5 countries led to a temporary increase in emissions, as seen most prominently for HCFC-141b and HCFC-142b (see also Montzka et al., 2009). A global cap in production came into force by an amendment of the Montreal Protocol in 2013. Interestingly, global HCFC emissions had already stabilized between 2008 and 2012, which was postulated by Montzka et al. (2015) as being an indication that industry was acting well in advance of the amendment. In the course of on-going reduction schemes, HCFC-22 in stationary air conditioners is being replaced by HFC mixtures (e.g., R-407a, R-407c) and HCFCs for foam blowing purposes are mainly substituted by HFC-245fa (C3H3F5) and HFC-365mfc (C4H5F5).
Current issues related to HCFCs: Unexpectedly, Laube et al. (2014) reported the detection of HCFC-133a (C2H2ClF3) in the global atmosphere–a substance that was apparently never used in consumer products. This finding was confirmed by Vollmer et al. (2015), who indicated that by-production during the manufacturing of HFC-134a (C2H2F4) and of HFC-125 (C2HF5) could be one of the sources. This was supported by the detection of HCFC-31 (CH2ClF) in the atmosphere by Schoenenberger et al. (2015), who related the presence of this compound to the production of HFC-32 (CH2F2). In both of these cases the fluorination step during the production appears to be incomplete, leading to the formation of small amounts of these HCFCs in addition to the desired HFCs.
4.3 Brominated ozone-depleting substances
Mole fractions and global emissions of the brominated ODSs are shown in Fig. 5C. Two groups are distinguished in terms of the Montreal Protocol; the fully anthropogenic halons and methyl bromide (CH3Br), which has both anthropogenic and natural sources.
Halons are long-lived brominated organic compounds, used in fire extinguishing systems. The most important halons are H-1211 (CBrClF2) in portable fire extinguishers and H-1301 (CBrF3) in stationary applications (e.g., computer centres and airplanes). In addition, H-2402 (C2Br2F4) was used in the former USSR largely in military applications. Production of halons for emissive uses was zeroed out in 1994 for Non-A5 countries and in 2010 for A5 countries. However, existing installations (banks) are partly exempted and therefore halon recycling and reuse is on-going, for example in airplanes and in critical stationary applications. Nevertheless, restrictions led to a decline in emissions in the 1990s and a maximum in mole fractions around 2005 for H-1211 and in the most recent years for H-1301.
In contrast to the fully anthropogenic halons, methyl bromide (CH3Br) has additional large natural sources from the terrestrial and oceanic biospheres. The most important anthropogenic source of CH3Br was its now banned application for pest management in agriculture. Although the ban was connected with a strict deadline, this deadline was extended by a few years through the granting of critical use exemptions (CUEs). This gradual phase-down was needed as replacements in some critical uses in food production only gradually had become available. Today around 99% of the controlled amounts have been successfully phased-out, with less than 0.3 Gg/yr CUE granted in 2016 (TEAP, 2017) and this usage will potentially end in 2019 (MBTOC, 2017). Although methyl bromide has partly been replaced by sulfuryl fluoride (SO2F2) for quarantine and pre-shipment applications (QPS) (Mühle et al., 2009), it is still allowed to be used for this purpose and a constant amount of nearly 10 Gg/yr has been emitted in recent years. This is however small compared to anthropogenic emissions of around 50 Gg/yr before restrictions of the Montreal Protocol (Carpenter et al., 2014).
As an effect of these restrictions mole fractions have declined from ∼9 ppt in the late 1990s to currently ∼7 ppt (Fig. 5).
Current issues related to brominated ozone-depleting substances: Large reservoirs of halons are still in use and are potentially a long-term source, albeit on a small scale (Vollmer et al., 2016). For methyl bromide, the unrestricted usage in QPS applications could threaten to offset the benefit gained by the phasing out of controlled uses, if it were to increase substantially in the future.
5 Conclusion
Continuous atmospheric measurements of ODSs have been essential for documenting and verifying the success of the restrictions imposed by the Montreal Protocol on Substances that Deplete the Ozone Layer. However, the generally positive picture–with a decreasing trend in all of the most important ODSs–should not be taken for granted. Therefore, on-going measurements are still extremely important to ensure that this trend is sustained and for identifying unresolved issues, such as detecting the location of on-going or increasing emissions of ozone-depleting substances (e.g., CCl4, CFC-11) and newly occurring substances. In addition, measurements can be used for understanding atmospheric processes (e.g., for estimating atmospheric lifetimes and transport timescales), for assessing regional and global emissions and for predicting atmospheric trends into the future.
These examples show that maintaining the observational capabilities provided by the global networks allows us to (1) assess the effectiveness of Montreal Protocol restrictions on atmospheric concentrations of controlled substances, (2) identify and track concentrations of other substances that might also impact stratospheric ozone, and (3) ultimately provide a better understand of changes in stratospheric ozone concentrations.