1 Introduction
The Deccan Volcanic Province (DVP) is one of the remarkable geo-events in the geologic history of the Indian plate that holds a prominent position in global tectonic models, particularly in the context of the assembly and break-up of southern Gondwanan landmasses, uplift of continental landmasses, magma generation in the deep mantle, climate change and biotic evolution (Courtillot, 1990; Khosla and Sahni, 2003; Subbarao and Courtillot, 2017). It had a significant impact on the tectonic and biotic evolution of the northward drifting Indian plate and its occurrence near the Cretaceous–Paleocene transition is thought to have played a significant role in the biotic mass extinctions at the K–Pg boundary (Courtillot et al., 1986; Courtillot and Fluteau, 2010, 2014; Khosla and Verma, 2015; Verma et al., 2017). A huge amount of research on the DVP has been done over the last four decades, and a wealth of information has been generated on its volcanology, structures, tectonics, stratigraphy, petrology, geochemistry, duration, paleomagnetism, biotic assemblages of the associated sediments, and its links to the K–Pg boundary, Chicxulub impact, Réunion plume, and global climate crisis (Courtillot et al., 1986, 2015; Richards et al., 2015; Verma et al., 2017). The enormous amount of data available on various aspects of the DVP is crucial to obtaining key insights into the chemistry and dynamics of the earth's interior and the environmental consequences of the huge volcanic eruptions on the atmosphere and biosphere.
The DVP forms one of the world's most important Large Igneous Provinces (LIPs) that records a massive accumulation of tholeiitic magmas in a relatively short time span (e.g., Chenet et al., 2007, 2009) near the K–Pg boundary, when lavas poured out through numerous fissures that broadly settled down in terrestrial to lacustrine environments (Nair and Bhusari, 2001). The main objective of this paper is to provide an overview of the current-state-of-knowledge of the stratigraphic aspects of the DVP, and to highlight the gaps that need future research attention.
2 Aerial extent, volume and sub-provinces
The Indian plate witnessed three continental flood basalt provinces during the Cretaceous: Rajmahal, Sylhet and Deccan traps. Among them, the Deccan traps are the most extensive; best exposed LIP, covering a vast tract of the Deccan plateau in the western and central parts of India. It has a large aerial extent, currently occupies an area of about 500,000 km2 in peninsular India, and is made-up of simple and compound pahoehoe flows (Fig. 1A). There is a wide variation in the estimates of the aerial extent and volume of the province. Its original aerial coverage was much broader than that of its present day extent, which would have been diminished by various prolonged erosion processes operating on it since its formation (Sen, 2001). The estimates of the original aerial extent of the province vary across the researchers own calculations, ranging from 1,000,000 km2 to 1,500,000 km2 to over 1,800,000 km2 (Sen, 2001). However, some workers estimated it from 1,000,000–2,000,000 km2 to as much as 2,600,000 km2 (Hooper, 1999).
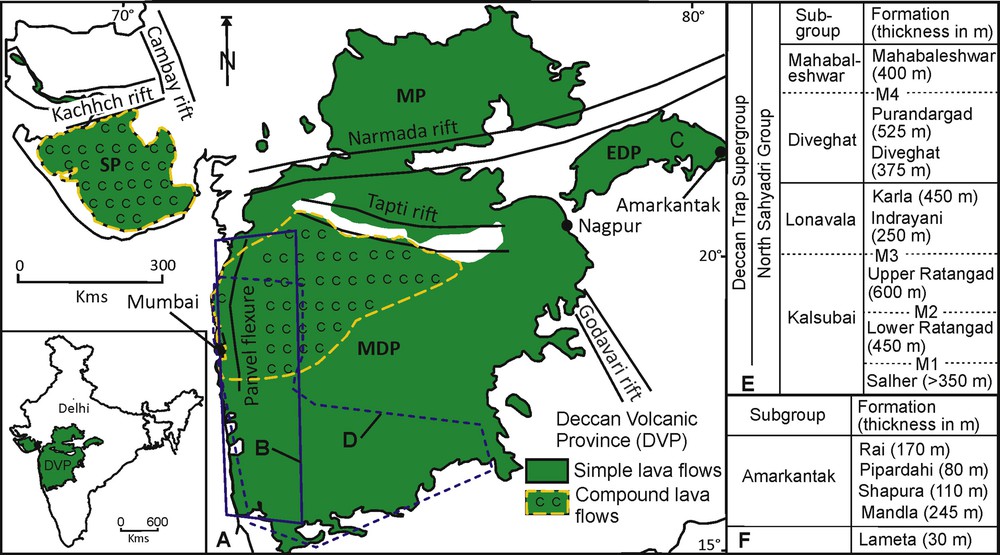
(Color online) A. Geological map of the Deccan Volcanic Province (DVP). The inset is a map of India showing the location of the DVP. B–C indicate the spatial coverage of the areas for which lithostratigraphy is available and the same is given in E (for Western Ghats) and F (for EDP), respectively. The open circle (deep blue) marked by D represents the area for which chemostratigraphy is well established and given in Table 1. MDP, Main Deccan Plateau; EDP, Eastern Deccan Plateau; MP, Malwa Plateau; SP, Saurashtra Plateau; M, Marker horizons (modified after Khosla and Verma, 2015).
The volume estimates of the province also vary, ranging from 250,000 km3, 1,300,000 km3, 9,000,000 km3 to over 10,000,000 km3 (Sen, 2001). However, estimates of 1,000,000 km3 and 3,000,000 km3 have been widely considered as conservative estimates for the present day volume and erupted volume, respectively, of the province (Courtillot et al., 1986). The estimates of the original aerial coverage and volume of the province still remain highly speculative because of a lack of data on some parts of the DVP (e.g., Sen, 2001). It includes data on the magma that may have been lost by its reaction with the crust-mantle during eruption, flows erupted on the ocean floor in the coastal area of the Western Ghats in the Arabian Sea, and flows submerged under off-shore sediments due to post-rift subsidence (Mahoney, 1988; Sen, 2001).
The DVP is bounded by four prominent tectono-structural units. These are the north-south trending arcuate Panvel Flexure to the west of the Western Ghats, the east-west trending Narmada-Tapti Rift System to the north, the north-south trending Cambay Rift System to the northwest, and the northwest-southeast trending Godavari rift to the southeast of the main DVP (Fig. 1A). It is divided into four sub-provinces (Fig. 1A): Main Deccan Plateau (MDP), Eastern Deccan Plateau (EDP), Malwa Plateau (MP) and Saurashtra Plateau (SP). The MDP alludes to the main DVP, lies south of the Narmada-Son rift valley, having extensive aerial coverage and a maximum thickness of ca. 3.5 km. The EDP is 900 m in thickness, covers an area of around 29,400 km2 and lies in the northeastern part of the MDP in central India. The MP represents the northernmost part of the DVP and lies to the north of the Narmada valley, having an aerial extent of ca. 80,000 km2 with an average thickness of 500 m. The SP lies northwest, covering an area of about 61,000 km2 and occurs in the Saurashtra and Kachchh regions of the western Gujarat. The breaks present within the lava pile commonly occur between two flows and are marked by the presence of intertrappean beds, paleosols, and scoriaceous material. Both infratrappean (~Lameta Formation) and intertrappean beds have yielded a diverse range of biotic elements that have profound implications for unraveling the biotic patterns during the volcanic episode (Kapur and Khosla, 2018; Khosla and Sahni, 2003; Khosla and Verma, 2015; Verma et al., 2016, 2017).
3 Lithostratigraphy
The earlier work on establishing stratigraphy of the DVP can be traced back to the nineteenth century, when the Deccan traps were divided into three groups: Lower, Middle and Upper traps, based on the distribution of intertrappean and volcanic ash layers sandwiched between the lava flows in relation to their height (Medlicott and Blanford, 1879). Later, Ghose (1976) classified the Deccan traps into two groups: Lower and Upper traps, based on chemistry and the nature of the volcanic eruptions. The Lower traps encompass the southern and eastern portions of the province, and the Upper traps occupy the western Coast (Gujarat) and the Narmada valley. These stratigraphic classifications clearly indicate that the traps are older in the eastern part while they are younger towards the western region. The recent radiometric and paleomagnetic investigations of the DVP questioned the validity of these classifications, as these studies have identified the oldest lava flows in the western portion of the province (e.g., Mahoney, 1988).
Godbole et al., 1996 presented a detailed account of the lithostratigraphic classification for a nearly 150,000 km2 aerially exposed lava pile of the MDP, located in western Maharashtra (Fig. 1A). It is bounded to the north by the Tapti River, to the south by Belgaum, to the west by the West Coast, and to the east by Aurangabad. They used observable physical features of lava flows comprising the presence of compound and simple flows, regional gradients of flows in undisturbed lava sequences, and occurrences of readily identifiable marker (M) horizons (Giant Plagioclase Basalts (GPBs) at various stratigraphic levels in the field to classify the lava pile. These workers have divided the lava pile of western Maharashtra, representing nearly one-third of the DVP, into eight formations belonging to four subgroups: Kalsubai, Lonavala, Diveghat and Mahabaleshwar (Fig. 1B, E). They also assigned the status of a supergroup for the whole of the DVP and a North Sahyadri Group to the lava pile of western Maharashtra (Fig. 1B, E).
On the basis of lithofacies, types, and the long distance continuity of well exposed lava flow outcrops of the EDP, Solanki et al., 1996 divided this lava pile into four formations: Mandla, Shahpura, Pipardahi and Rai, in ascending order (Fig. 1C, F). All formations are characterized by an independent event of volcanic eruptions, and their boundaries are marked by a period of non-volcanic activity, which led to the development of intertrappean beds at the top of each formation, except the Rai Formation. These formations are thick in the centre and thin out towards the peripheral regions. The lithostratigraphy of other parts of the DVP, comprising lava piles exposed in the central region of the main DVP south of the Tapti River, Satpura Range, and MP, has been proposed based on geological quadrangle maps prepared by the Geological Survey of India. The lava piles of these areas have been classified into various formations, out of which, many formations lack formal description, but were concisely presented by Nair and Bhusari (2001). Surprisingly, the correlation among the various lithostratigraphic units proposed for various areas of the DVP (Western Ghats, Mandla, Satpura or Malwa) is not worked out at a finer scale. Therefore, the lithostratigraphy of one part may not be applicable to other parts of the province. The proposed lithostratigraphic schemes have some limitations as far as the code of stratigraphic nomenclature is concerned. For example, the hierarchy of the various lithostratigraphic units is inconsistent. The marker horizons (M1 to M4) are used to mark the contact between formations as well as among the subgroups (Fig. 1E). Most of the above mentioned lithostratigraphic schemes did not provide data about type sections with locations, sampled heights and lava flow boundaries for each formation. The long distance correlation difficulties of an individual lava flow or a group of flows of the DVP pose a serious problem in establishing a reliable lithostratigraphic scheme.
4 Chemostratigraphy
The systematic geochemical mapping in parts of the lava pile coupled with magnetostratigraphy and radiometric dating studies offer the most reliable results that led to the establishment of a detailed chemostratigraphy, particularly of the Western Ghats area (Figs. 1D and 2). Najafi et al. (1981) were the first to record geochemical changes in flow packages in India with height and to classify a lava pile into distinct geochemical units. They studied the geochemistry of a lava pile containing 47 flows, situated between Mahad and Mahabaleshwar in the Western Ghats. Based on major and trace elements analysis, they divided the Mahad-Mahabaleshwar section into three groups: Lower, Middle and Upper. Subsequently, Mahoney et al. (1982) noted systematic changes in major and trace elements as well as Sr-Nd ratios in the Mahabaleshwar lava pile, and, based on Sr-Nd ratios, they divided the lava pile of the Ambenali Ghat (northwest of Mahabaleshwar) into Lower and Upper groups. Cox and Hawkesworth (1984), based on trace elements (Sr, Ba, Rb, Zr, and Nb), divided a 1200-m-thick lava pile of the Mahabaleshwar area into three formations: Poladpur, Ambenali and Mahabaleshwar, in ascending order. These authors found that these formations are geochemically similar to the three units established by Najafi et al. (1981).

Summary of litho-, chemo-, chrono- and magnetostratigraphy of the DVP. Lithostratigraphy compiled after Godbole et al., 1996; chemostratigraphy after Beane et al., 1986; Vanderkluysen et al., 2011; chronostratigraphy of the MDP after Richards et al. (2015), MP after Schöbel et al. (2014) and the EDP after Shrivastava et al. (2015), and magnetostratigraphy of the MDP after Chenet et al. (2009), Richards et al. (2015), the MP after Schöbel et al. (2014) and the EDP after (1) Vandamme and Courtillot (1992) and (2) Shrivastava et al. (2015). Refer to Table 2 for ages.
Subsequently, Cox and Hawkesworth (1985) presented a chemostratigraphic framework of the Mahabaleshwar lava pile based on whole-rock geochemistry and divided the lava pile into five formations: Bushe, Lower Poladpur, Upper Poladpur, Ambenali and Mahabaleshwar, from base to top. Meanwhile, Bodas et al. (1984) carried out systematic geological and geochemical mapping of the stratigraphically older lava pile that has been situated between Nasik and Junnar area (north of Mahabaleshwar) as compared to the lava pile that lies farther south. Four distinct chemo-units: Jawhar, Igatpuri, Thakurvadi, and Bhimashankar, in ascending order, were recognized (Bodas et al., 1984). Later, Beane et al., 1986 presented a comprehensive account of the chemostratigraphy of MDP, covering an area of about 7000 km2, lying between 18° 12′ N and 19° 15′ N latitudes, bounded to the north by Igatpuri and to the south by Mahabaleshwar in the northern Western Ghats. Based on geochemical characteristics (major and trace element analysis, rare-earth and 87Sr/86Sr ratio) and field markers, Beane et al., 1986 divided the lava pile into three subgroups viz. Kalsubai, Lonavala and Wai, and ten formations such as Jawhar, Igatpuri, Neral, Thakurvadi, Bhimashankar, Khandala, Bushe, Poladpur, Ambenali and Mahabaleshwar, in ascending order, respectively (Table 1). While presenting geochemical and isotopic investigations of the lava pile from the southernmost part (near Belgaum) of the MDP, Lightfoot and Hawkesworth (1988) recognized two youngest chemo-units: Panhala and Desur, which lie above the Mahabaleshwar Formation (Table 1). In a nutshell, a well-defined chemostratigraphic framework consisting of three subgroups and 12 formations is now available for the lava pile having a thickness of around 3.5 km in the Western Ghats situated between Igatpuri and the southern edge (i.e. Belgaum area) of the DVP (Fig. 1D; Table 1). The GPB flows are well developed from the Pune to Igatpuri traps in the northern Western Ghats; thus, both GPBs and geochemical criteria have been used to establish a chemostratigraphy. The GPBs are almost absent to the south of Pune; as a consequence, chemical units of the southern part of the Western Ghats are largely established based on geochemical characteristics of the lava flows.
Chemostratigraphy of the Deccan Basalt Group, Western Ghats (after Beane et al., 1986; Peng et al., 1994; Vanderkluysen et al., 2011, and references therein).
Group | Sub group | Formation (thickness in m) | Member or Chemical type (CT) | (87Sr/86Sr)t | Av. Mg No. | TiO2 | Av. Ba/Zr | Av. Zr/Nb |
Deccan Basalt | Wai | Desur (~ 100 m) | – | 0.7072–0.7080 | 48 | 1.6–1.9 | 1.2 | 11.4 |
Panhala (> 175 m) | – | 0.7046–0.7055 | 52 | 1.6–2.3 | 0.5 | 14.8 | ||
Mahabaleshwar (280 m) | – | 0.7040–0.7055 | 47 | 2.5–4.3 | 0.5 | 11.4 | ||
Ambenali (500 m) | Ambenali CT | 0.7038–0.7044 | 49 | 1.9–3.1 | 0.3 | 14.4 | ||
Poladpur (375 m) | Upper | 0.7061–0.7083 | 52 | 1.8–2.3 | 0.4 | 12.7 | ||
Lower | 0.7053–0.7110 | 56 | 1.5–2.0 | 0.7 | 14.0 | |||
Sambarkada | 0.7075 | 43 | 2.2–2.3 | 0.9 | 12.6 | |||
Valvhan | 0.7068 | 42 | 2.5–2.6 | 0.9 | 12.3 | |||
Ambavne | 0.7056 | 45 | 2.0–2.1 | 0.4 | 14.0 | |||
Lonavala | Bushe (325 m) | Pingalvadi | 0.7127 | 61 | 1.1–1.2 | 0.8 | 17.2 | |
Bushe CT | 0.713–0.720 | 55 | 1.0–1.3 | 1.1 | 15.3 | |||
Shingi Hill | 0.7180–0.7181 | 49 | 1.3–1.4 | 2.0 | 16.2 | |||
Harishchandragad | 0.7078–0.7079 | 46 | 2.0–2.1 | 1.0 | 12.9 | |||
Karla Picrite | 0.7147–0.7150 | 68 | 1.1–1.2 | 0.8 | 19.7 | |||
Bhaja | 0.7120–0.7164 | 58 | 1.3–1.5 | 0.6 | 18.2 | |||
Khandala (140 m) | Rajmachi | 0.7093–0.7102 | 44 | 2.1–2.5 | 1.2 | 14.6 | ||
Khandala Phyric CT | 0.7085 | 41 | 2.4–2.8 | 1.3 | 13.6 | |||
Khandala Aphyric 3 | 0.7107 | 49 | 1.7 | 3.1 | 12.9 | |||
Madh | 0.7095 | 58 | 1.6 | 2.8 | 14.0 | |||
Boyhare | 0.7102 | 63 | 1.2–1.3 | 2.1 | 13.7 | |||
Khandala Aphyric 2 | 0.7124 | 57 | 1.2 | 3.9 | 13.5 | |||
Khandala Phyric CT | 0.7077 | 41 | 2.5–2.8 | 1.3 | 13.6 | |||
Khandala Aphyric 1 | 0.7094 | 61 | 1.0–1.1 | 1.8 | 15.4 | |||
Dhak Dongar | 0.7071–0.7072 | 40 | 2.9–3.1 | 1.0 | 13.1 | |||
Khandala Coarse Grained | 0.7098 | 48 | 1.4–1.6 | 1.5 | 18.7 | |||
Monkey Hill GPB | 0.7073–0.7075 | 41 | 3.1–3.4 | 1.1 | 12.2 | |||
Giravli GPB | 0.7068–0.7074 | 45 | 2.8–3.1 | 0.8 | 12.1 | |||
Kalsubai | Bhimashankar (140 m) | Bhimashankar CT | 0.7067–0.7076 | 47 | 1.9–2.6 | 0.8 | 11.7 | |
Manchar GPB | 0.7075–0.7077 | 42 | 2.9–3.1 | 0.5 | 13.2 | |||
Thakurvadi (650 m) | Thakurvadi CT | 0.7073–0.7080 | 58 | 1.8–2.2 | 0.8 | 12.0 | ||
Water Pipe | 0.7099–0.7112 | 59 | 1.4–1.6 | 1.5 | 12.3 | |||
Member | 71 | 1.0–1.1 | 1.3 | 12.0 | ||||
Paten Basalt | 0.7224 | 58 | 1.0 | 1.3 | 15.2 | |||
Thakurvadi CT | 0.7067–0.7070 | 58 | 1.8–2.2 | 0.8 | 12.4 | |||
Ashane | 0.7068 | 62 | 2.0–2.1 | 1.2 | 9.6 | |||
Thakurvadi CT | 0.7080–0.7084 | 58 | 1.8–2.2 | 0.8 | 11.6 | |||
Jammu Upper | 0.7112 | 34 | 2.7 | 1.6 | 9.8 | |||
Patti Middle | 0.7099 | 46 | 2.2–2.3 | 1.5 | 8.4 | |||
Member Lower | 0.7066–0.7067 | 56 | 1.7–20 | 1.1 | 11.2 | |||
Neral (100 m) | Tunnel Five GPB | 0.7082–0.7083 | 36 | 3.3–3.5 | 1.0 | 10.8 | ||
Tembre Basalt | 0.7084 | 43 | 2.8–3.0 | 1.1 | 10.6 | |||
Neral CT | 0.7062–0.7073 | 62 | 1.5–1.7 | 1.2 | 12.3 | |||
Ambivil Picrite | 0.7104 | 67 | 1.4–1.5 | 1.7 | 18.6 | |||
Igatpuri (> 200 m) | Kashele GPB | 0.7102–0.7122 | 40 | 2.6–3.1 | 1.1 | 11.5 | ||
Mg-rich Igatpuri | – | 59 | 1.4–1.9 | 1.0 | 13.3 | |||
Igatpuri Phyric | 0.7107–0.7124 | 49 | 1.9–2.2 | 1.1 | 14.3 | |||
Jawhar (> 700 m) | Thal Ghat GPB | 0.7108 | 36 | 3.6 | 0.9 | 10.7 | ||
High field strength element-poor Jawhar | – | 51 | 1.3–1.6 | 1.5 | 13.0 | |||
Plagioclase Phyric | 0.7085 | 38 | 3.0 | 1.0 | 11.7 | |||
Mg-rich Jawhar | 0.7128 | 59 | 1.4–1.9 | 1.0 | 12.7 | |||
Kasara Phyric | 0.7091 | 39 | 2.8–3.0 | 1.0 | 10.8 |
4.1 Kalsubai subgroup
It is the basal subgroup, consists of the oldest lava flows, and is largely exposed around the Kalsubai Mountain in the northern parts of the Western Ghats. It is about 2000 m in thickness and marked by the occurrence of GPBs that are commonly present at the formation boundaries. It is mainly composed of more Mg-rich amygdaloidal compound flows as compared to the flows of the two overlying subgroups, and picrite basalts (>10% MgO) as well as picrites (>18% MgO) are common in the Mg-rich flows (Beane and Hooper, 1988). The basal Jawhar Formation is largely exposed between Trimbak and Malsej Ghat. It represents the first phase of volcanic eruptions and is > 700 m in thickness. It consists of flows of aphyric, microphyric, porphyritic to GPBs nature and shows a southerly dip (Bodas et al., 1988). The upper boundary of the formation is discernible by the presence of the Thal Ghat GPB. The Igatpuri Formation overlies the Jawhar Formation and occurs in the northwestern margins of the Western Ghats around Igatpuri. It includes compound flows ranging from microphyric to evolved plagioclase phyric types. It is about 200 m in thickness and characterized by having a high 87Sr/86Sr ratio (>0.709). The base of the formation shows an increase in MgO and CaO concentrations, with a marked decrease in TiO2, P2O5, Y, NB and Zr concentrations as compared to the top of the Jawhar Formation (Bodas et al., 1988). The Kashele GPB flow marks the upper boundary of the formation (Beane et al., 1986). The Neral Formation rests over the Kashele GPB member and is well developed on the northwestern margins of the Western Ghats around the villages of Neral and Matheran. It has attained a maximum thickness (ca. 145 m) in the Bivpuri area, and decreases northwest and south towards Neral and Bor Ghat, respectively (Beane et al., 1986). It mostly yields diverse, coarse-grained, Mg-rich compound and amygdaloidal flows characterized by mafic-rich and plagioclase-devoid. The Tunnel Five GPB forms the topmost member of the formation and is underlain by the Thakurvadi Formation. This formation derives its name from the Thakurvadi railway station on the Bombay-Pune line, where it preserves a thick pile of compound and picrite basalt flows (Beane et al., 1986). It represents a thick and extensive lava pile of fine to coarse grained, aphyric to micro-phyric, compound and amygdaloidal flows. Its chemical compositional range comprises TiO2 1.3-3.3%, MgO 3.5-17%, P2O5 0.12-0.30%, and CaO 7.47-12.5%. The Bhimashankar Formation is the youngest formation of the subgroup, overlies the Thakurvadi Formation and usually consists of compound flows. It has a variable thickness; a maximum thickness of ca. 140 m occurs between Bivpuri and Damdamia and a minimum thickness of ca. 20–60 m in the Neral-Bor Ghat region. It is characterized by possessing an intermediate position between the magnesia-rich Thakurvadi Formation and ferrous oxide to incompatible-element enriched overlying Khandala Formation, TiO2 ranges from 1.9 to 2.5%, MgO from 5.0 to 6.3% and Ba/TiO2 is typically less compared to the flows situated just below and above the formation (Beane et al., 1986).
4.2 Lonavala subgroup
It rests above the Bhimashankar Formation, is around 525 m in thickness, and is characterized by the presence of simple flows, high Ba/Sr and Ba/Ti values, and also by a lower MgO for any given TiO2 content (Beane et al., 1986). The Khandala Formation is its basalmost formation and is chiefly composed of simple flows of wide-ranging geochemistry and petrology. Overlying the Khandala Formation is the Bushe Formation that largely covers the northwestern Pune region and comprises coarse-grained, aphyric, amygdaloidal compound lava flows of distinctive chemical range. It shows very high 87Sr/86Sr values (>0.713), relatively constant concentration of TiO2, Zr, and Sr, a high Mg concentration and low concentrations of high-field-strength-incompatible elements (Beane et al., 1986; Cox and Hawkesworth, 1985).
4.3 Wai subgroup
It is the youngest subgroup, lies above the Lonavala Subgroup and constitutes more than 50% of the total thickness of the Deccan basalts with five formations (Beane et al., 1986; Peng et al., 1994). The boundary between the Lonavala and Wai subgroups is clearly recognized in the field by predominantly simple flows of sharp isotopic and elemental composition of the Wai Subgroup. The simple flows of the Wai Subgroup largely consisting of minute phenocrysts of plagioclase, are more evolved than the oldest flows. The Poladpur Formation rests above the Bushe Formation and mainly occurs west to the Pune and north to the Mahabaleshwar. It is chiefly made up of simple flows having porphyritic and finer groundmass texture. Based on the chemical characteristics, Cox and Hawkesworth (1985) divided this formation into Lower Poladpur and Upper Poladpur units, and it has been argued that the discrimination between these units is not possible in the field (e.g., Beane et al., 1986). The contact between the Bushe and Poladpur formations is marked by a sharp decrease in 87Sr/86Sr values (i.e. < 0.713) and by abrupt changes in TiO2 and Mg values (Beane et al., 1986; Cox and Hawkesworth, 1985; Devey and Lightfoot, 1986). A complete section of the overlying Ambenali Formation is exposed in the Sinhagad area, and its other exposures occur in the vicinity of the Varandha Ghat, Kamshedi Ghat and Wai–Panchgani areas (Cox and Hawkesworth, 1985). It is mainly composed of moderately to highly porphyritic simple flows and characterized by having low concentrations of Large-Ion-Lithophile (LIL) elements, moderate to high concentration of High-Strength-Field (HSF) elements, small LIL/HSF elemental ratios and lower 87Sr/86Sr value (Beane et al., 1986). The succeeding Mahabaleshwar Formation forms a lid to the Mahabaleshwar Plateau. The base of the formation is marked by a sharp change in elemental and isotopic content in the Kelghar and Wai-Panchgani areas, where a small increase in the 87Sr/86Sr ratio, Ba content and Ba/Y ratio has been recorded (Cox and Hawkesworth, 1985). The formation is composed of simple flows, which progressively become more porphyritic from base to top and its lower portion shows a high content of Ba, Sr, Nb, Rn and K2O as compared to that of the underlying Ambenali Formation. The Panhala Formation mainly occurs in the Belgaum area, Karnataka. The flows of this formation are characterized by high Zr/Nb values, low concentrations of Sr, Rb, Ba and TiO2 as well as high 87Sr/86Sr ratios and a lower concentration of LIL elements than the Ambenali Formation (Devey and Lightfoot, 1986). The youngest Desur Formation lies above the Panhala Formation, and is confined to a few flows that occur in the southern and southwestern regions of the Belgaum area. The basalt of the formation is fine to medium grained, moderately porphyritic and largely showing Mahabaleshwar-type eruptions. It shows high TiO2 content, moderate MgO concentration, low values of K2O, Mg number and Zr/Nb ratio, absence of Nb and rich concentration of LIL elements.
Numerous researchers have extended this work to the other parts of the DVP and recorded the existence of some of the chemo-units of the Western Ghats to its eastern, northeastern (Mandla lobe), central and northern (MP) regions (Fig. 3). The geochemical and isotopic work on the DVP strata lying to the north and south of the Narmada valley recognizes the presence of some of the chemo-formations belonging to the Lonavala and Wai subgroups. The lava piles, exposed at Chikaldara, Behram Ghat, Akot-Harisal, Tornamal, and Bijasan Ghat to the south of the Narmada valley have been investigated by various researchers. On the basis of major and trace elements analyses of flows, the chemical signatures for the presence of the Poladpur and Ambenali formations have been found in the Chikaldara, Behram Ghat and Akot-Harisal areas (Deshmukh et al., 1996). The chemical and isotopic studies of a ca. 870-m-thick lava pile, exposed near Toranmal (Fig. 3) reveal the wide occurrence of the Ambenali Formation (Mahoney et al., 2000). The various flows having geochemical affinities with those of the Bushe and Mahabaleshwar formations have been recorded from Toranmal. The major and trace element analyses of a lava pile exposed around the Bijasan Ghat indicate the widespread distribution of the Poladpur Formation and the absence of the Ambenali Formation.
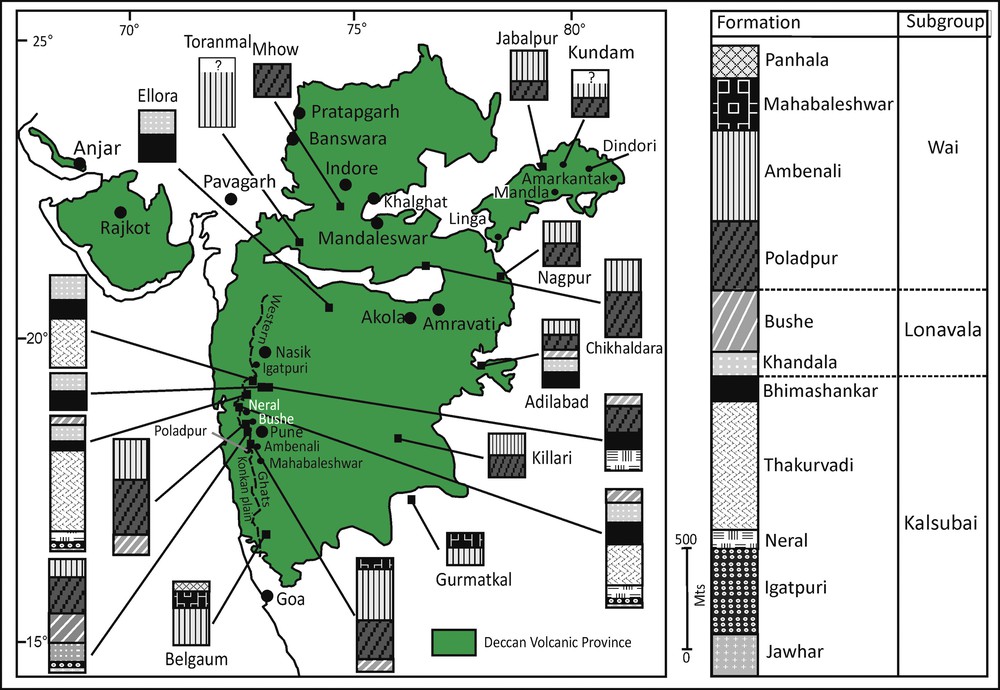
(Color online) Sketch map showing the chemostratigraphic units of the DVP (compiled from Beane et al., 1986; Schöbel et al., 2014; Vanderkluysen et al., 2011).
An extensive geochemical study of a 620-m-thick lava section, located north of Chikaldara, shows that flows similar to the southwestern Ambenali chemical-type occur at the top of the section, the flows beneath the Ambenali chemical-type are similar to the Poladpur Formation and there are several flows interspersed at the lower level of the section showing closer affinities with the Khandala Formation (Peng et al., 1998). Another work of Peng et al. (1998), on a 690-m-thick lava pile, situated south of the Mhow, reveals the presence of flows similar to the Khandala and Poladpur formations of the Western Ghats. However, the middle and top portions of the section have yielded several Bushe-like flows (Peng et al., 1998). Little work has been carried out to establish the chemostratigraphy of the MP. Rao et al. (1985) carried out a chemostratigraphic study in the two lava sections of the MP, viz: Khalghat–Mhow and Mandaleswar–Pipaljopa, exposed along the northern and southern banks of the Narmada River, respectively. Based on major and trace element analysis, they divided the Khalghat–Mhow section into three formations: Narmada, Manpur and Mhow, and the Mandaleswar–Pipaljopa section into four formations, viz. Narmada, Manpur, Mhow and Satpura, in ascending order. Very recently, Subbarao and Courtillot (2017) have correlated the Narmada Formation and the overlying Manpur Formation with the Kalsubai Subgroup, and the Mhow and Satpura formations with the Wai Subgroup. Khadri et al. (1999) examined a 471-m-thick lava pile of the MP, exposed in the Mograba area of the Burimandaw plateau, Madhya Pradesh and recognized three subgroups comprising four formations: Bhimashankar, Khandala, Bushe, and Poladpur. The Kalsubai Subgroup has a limited occurrence, and is mainly composed of coarse-grained, amygdaloidal, phyric, compound flows of the Bhimashankar Formation. The Lonavala Subgroup has a wide spatial coverage and comprises the Khandala and Bushe formations. Among them, the Khandala Formation is made of aphyric to phyric simple flows and has a wide aerial extent compared to the aphyric amygdaloidal simple flows of the Bushe Formation. The youngest Wai Subgroup is represented by the presence of only the Poladpur Formation, consisting of phyric, compact, massive and simple flows. In the EDP, only a few efforts have been made up to now to establish the chemostratigraphy of the lava pile. Yedekar et al., 1996 and Deshmukh et al., 1996 geochemically analyzed basaltic flows of the Mandla lobe and correlated them with chemo-units of the Western Ghats. Their study demonstrated the possible equivalence of the Poladpur and Ambenali formations in the EDP. Peng et al. (1998) analyzed lava flows from the south and southwest of Jabalpur and inferred the presence of Ambenali-like flows in upper parts of the lava pile and underneath the Poladpur Formation. One flow similar to the Khandala Formation is known from the Jabalpur area. In a nutshell, it appears that Poladpur and Ambenali formations are the dominant and spatially most widely spread chemo-units of the EDP.
5 Magnetostratigraphy
The DVP is one of the most intensively studied units in terms of paleomagnetic investigations. Earlier studies date back to the 1950s, when some workers (e.g., Deutsch et al., 1958; Sahsrabudhe, 1963) carried out pioneering works to document the number of magnetic reversals within the lava pile (Fig. 4). Some of the intriguing findings of these paleomagnetic works are: (i) the paleomagnetic examination of lava flows at Amba and Nipani carried out by Deutsch et al. (1958) revealed that underlying flows are of reversed magnetic polarity and overlying flows are of normal magnetic polarity, and (ii) the extensive paleomagnetic analysis of lava flows and dykes of the MDP exposed at Mahabaleshwar, Nipani, Amba and Khandala illustrated that flows lying below the altitude of ca. 600 m above mean sea level have reversed polarity and those occurring above this altitude show normal polarity. The later has very crucial implications as it favors a two-fold magnetostratigraphy for the DVP, with the lower formation (lies below ca. 600 m height) characterized by reversed polarity and the upper formation by normal polarity, only one geomagnetic field reversal during whole period of eruption and a short time span for the Deccan volcanism.

(Color online) Map of the DVP showing the areas and traverses in which paleomagnetic data was collected. A is an area studied by Deutsch et al. (1958); B by Sahsrabudhe (1963); C by Wensink and Klootwijk (1971); D–E is a traverse of Vandamme et al. (1991), F (area studied) by Chenet et al. (2008, 2009); G-J (traverse) of Rao et al. (1985), K (area studied) by Khadri (2003), L–Q (traverses) of Schöbel et al. (2014), R–S (traverse) of Vandamme and Courtillot (1992) and T–U (traverses) of Pathak et al. (2016).
Subsequently, numerous workers did paleomagnetism studies on lava flows and dykes of various sub-provinces and inferred the presence of more than one reversed polarity, with reversed and normal polarity occurring at various altitudes within the vertical successions of the DVP (Pal, 1969). These studies were largely focused on the understanding of drift of the Indian plate during the Deccan volcanism and their magnetic chrons were not correlated with radiometric ages and relative dating of the DVP, but significantly pointed out a short duration of the DVP, usually less than 5 Ma. The important paleomagnetic observations of these classic studies include: the lava pile of the DVP has more than one reversed polarity, magnetic chrons cannot be correlated with the elevation of the lava pile, a normal-reverse-normal (N–R–N) magnetostratigraphy, and paleomagnetism is a useful tool for knowing the duration of the DVP and for demarcating the K–Pg boundary in conjunction with radiometric age data. As a consequence, numerous researchers across the globe felt the need for more detailed paleomagnetic investigations of the DVP (e.g., Courtillot, 1990; Courtillot et al., 1986; Vandamme et al., 1991).
Wensink and Klootwijk (1971) carried out paleomagnetic studies on five sections: Katraj Ghats, Kambatgi Ghats, Ghats west of Bhor, Ghats west of Mahabaleshwar and Amba Ghats, of the lava pile situated south of Pune (Fig. 4). They found normal polarity from the lava flows of the Katraj and Bohr Ghats areas. Reversed polarity was recorded from the lava flows that occur at an altitude of 1000 m in the Mahabaleshwar and Kambatgi outcrops, and at an altitude of 600 m from the lava flows of the Amba section. This study concludes that natural remnant magnetization of the MDP lava pile (Western Ghats), is predominantly reversed. Afterwards, Courtillot et al. (1986) and Vandamme et al. (1991) carried out extensive investigations on the thick lava pile of the DVP using paleomagnetic, paleontological and radiometric data, and, also presented a detailed review of published paleomagnetic and radiometric data sets of the DVP. These authors collected samples from 27 sites, from three traverses, viz. Nagpur to Dongargaon, Nagpur to Bombay and Bombay to Pune (Fig. 4). Initially, Courtillot et al. (1986) presented preliminary results, which later on were elaborated by Vandamme et al. (1991). These authors established a three chron: N–R–N magnetostratigraphy for the entire DVP. It consists of a basal thin 30N polarity lava pile, mostly in the north that began with the Abathomphalus mayaroensis foraminiferal zone (known from the infratrappean sediments of Rajahmundry area, southeastern coast of India underlying the DVP) of Maastrichtian age, followed by a thick intermediate 29R polarity pile, largely covering much of the MDP and overlain by a topmost 29N polarity pile, occurring in the southwest. These authors did radiometric studies and obtained ages that range from 66 to 55 Ma ago by employing the 40K/40Ar method for dating flow samples. While reviewing the early published radiometric age data set, they found 67 to 60 Ma ago is a reliable age range. Further, paleomagnetism work revealed that the reversal rate was high at 66 Ma ago, when nearly 70–75% total volume of eruption took place within 29R (Vandamme et al., 1991). And, the total duration of eruption was not more 3 Ma, or much less (<1 Ma), with eruption starting close to 30N-29R chron boundary, and volcanic activity lasting throughout chron 29R. It has been recorded that the 29R/29N boundary level is traceable over much of the DVP at different heights and their interpolation assigned a 29R/29N boundary contour map across the lava pile (Fig. 5), that has profound implications for understanding the structural architectural features (Vandamme and Courtillot, 1992). The observations made by Courtillot et al. (1986) that much of the lava was emplaced during chron 29R underlines the definite role and link of the DVP to the K–Pg mass extinction.
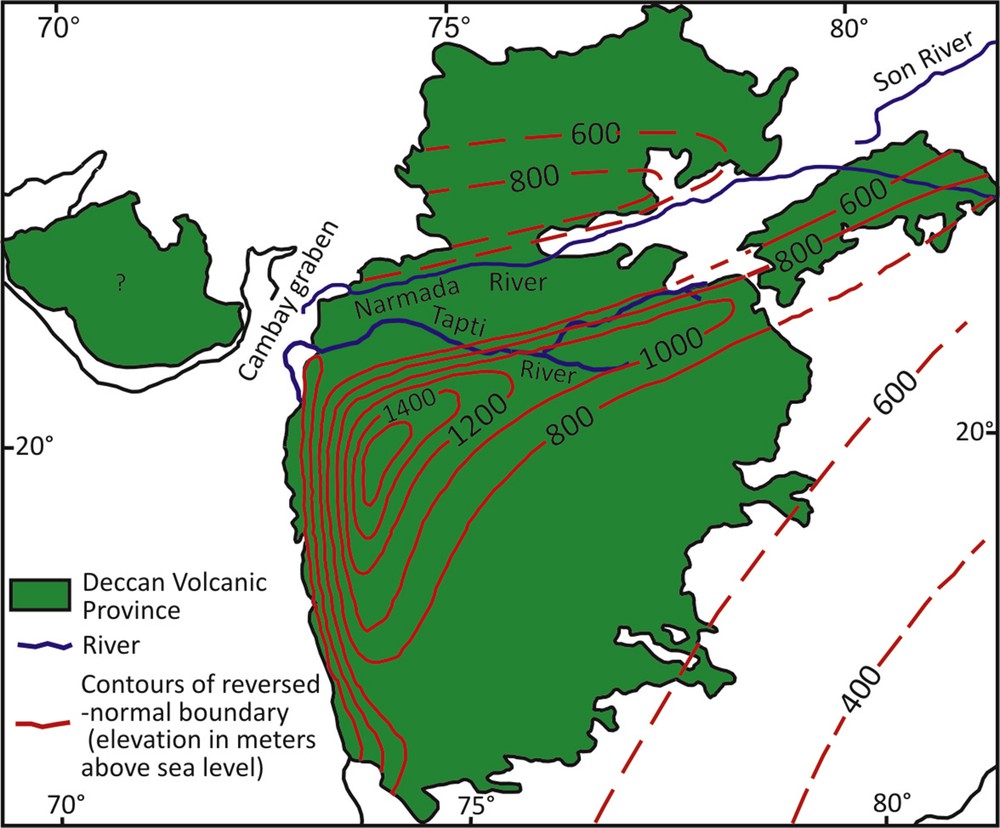
Map of the DVP showing interpolated contours of the 29R/29N boundary (after Vandamme and Courtillot, 1992).
In seminal works, Chenet et al. (2008, 2009) thoroughly re-studied and carried out paleomagnetic analysis of a 3.5-m-thick lava pile exposed along the Western Ghats, in order to know the number of short eruptive events, reconstruct their eruptive history and estimate the amount of greenhouse gases (SO2 and CO2) released into the atmosphere during volcanic eruption that might have altered climate at the K–Pg boundary (Fig. 4). These workers analyzed a large dataset of samples collected from 148 sampling sites, belonging to the different chemo-units of the lava pile. Chenet et al. (2009) presented paleomagnetic results of lava samples collected from eight sections (Val River-Trimbak, Mokhada-Khodala, Khodala–Igatpuri, Shahapur–Igatpuri, Devale–Ghoti–Arthur hill lake, Matheran-Neral, Khandala–Khopoli and Bushe), largely consisting of the oldest part of the pile belonging to the basal Kalsubai and overlying Lonavala subgroups. In the meantime, Chenet at al. (2008) also carried out a detail paleomagnetic analysis of lava samples of the topmost Wai Subgroup, collected from the Mahabaleshwar-Poladpur Ghat section. These studies of paleomagnetism in conjunction with radioisotopic and paleontological data have indicated that volcanic eruption occurred in three distinct phases (Chenet et al., 2007, 2009), where each phase is marked by a magnetic polarity chron and thus, favor an 30N–29R–29N magnetostratigraphy for the Western Ghats lava pile. The first phase of volcanic eruption began at or prior to chron 30N, the second phase started with 29N and ended at the K–Pg mass extinction, and the third phase started in the uppermost 29R and was completed during early 29N. A recent study by Sprain et al. (2019) did not favor three phases of eruption of the DVP based on high precision argon–argon dating of volcanic ash. The record of 30N/29R is probably absent, and the boundary between the Ambenali and Mahabaleshwar formations might coincide with the 29R/29N transition in the Western Ghats (Chenet et al., 2009).
The magnetostratigraphy of the MP and traps of the Satpura Range received considerably less attention. Rao et al. (1985) presented paleomagnetic analysis of two lava sections: Khalghat–Mhow and Mandaleswar–Pipaljopa of the MP (Fig. 4). The lowermost seven flows of the Khalghat–Mhow show normal polarity and its upper 13 flows yield reversed polarity. The Mandaleswar–Pipaljopa section has normal polarity for the basal flows of 21 m in thickness, overlain by 476-m-thick flows of reversed polarity and capped by the upper flows of 52 m in thickness of normal polarity. Thus, an N–R and N–R–N magnetostratigraphy was proposed for lava piles of the Khalghat–Mhow and the Mandaleswar–Pipaljopa region, respectively, of which the N–R sequence is the oldest. Further, it was interpreted that a vertical displacement took place, probably along the Narmada River, which threw up the northern side relative to the southern side of the Narmada River (Rao et al., 1985). While examining lava pile exposed to the southwest of Mhow (northern side of the Narmada; Fig. 4), Khadri (2003) observed lower normal and middle reversal polarity chrons from the Jamdarwaza–Mandaleswar area, and the upper normal polarity zone from the top of Singarchori hills. Thus, an N–R–N magnetostratigraphy was also inferred for the lava pile occurring to the north of the Narmada River. Among them, lower normal and reversed chrons are thought to be linked to 33R and 29R, respectively. An N–R magnetostratigraphy has also been inferred for the Mograba area, Madhya Pradesh (Khadri et al., 1999).
Recently, Schöbel et al. (2014) thoroughly investigated the western margin of the MP covering the areas of Pratapgarh, Banswara, Dhar, Mhow, and Indore. They took three traverses: A–A′, B–B′, and C–C′, along the northern margin lying to the west of Pratapgarh, westernmost part of western plateau to the southeast of Banswara and southern part to the south of Mhow, respectively (Fig. 4). The two magnetic polarity chrons (30N and 29R) and a 30N/29R transition have been recorded from these areas. They used 40Ar/39Ar dating of the lava pile for assigning ages to the magnetic chrons. It is worth mentioning that Schöbel et al. (2014) favor a 30N–29R magnetostratigraphy for the lava pile of the western MP. They also noted that the lower normal chron of Khadri (2003) cannot be correlated with 33R, this being due to the lack of precise radiometric data available at the time. The presence of a 30N/29R transition in the MP and its absence in the Western Ghats show that the lava of the MP started erupting before the flows of the Western Ghats. The magnetostratigraphic correlation of the MDP and the MP clearly shows that the main volcanic activity took place during chron 29R in both these parts of the DVP (Schöbel et al., 2014).
The magnetostratigraphic studies of the EDP have been undertaken by sundry workers (e.g., Courtillot et al., 1986; Pathak et al., 2016; Vandamme and Courtillot, 1992). The significant efforts were carried out in establishing a magnetostratigraphy of the EDP during the early 1990's, when Vandamme and Courtillot (1992) presented a detailed account of the paleomagnetic investigation of the lava flow samples taken along two transects: Jabalpur–Dindori and Jabalpur–Seoni road (Fig. 4). These workers also analyzed the samples of the Upper Cretaceous Lameta Formation collected in the vicinity of Jabalpur. Their analysis shows a few flows showing normal polarity are present at the base of the lava pile near the Jabalpur area, which are further overlain by a thick flow sequence of reversed flows. Finally, flows of normal polarity found at the top of the lava pile of the EDP (Vandamme and Courtillot, 1992). A reversed polarity is detected for the underlying beds of the Lameta Formation. They preferred an N–R–N magnetostratigraphy of the EDP and correlated it with the well-established chrons 30N–29R–29N of the Western Ghats. This study also revealed that the flows of the EDP lava pile are not perfectly horizontal; instead, possessing either a synform–antiform structure that runs parallel to the Narmada–Son rift system. More recently, Pathak et al. (2016) attempted to establish a magnetostratigraphy of the EDP. They took three transects: Rukher–Sivni, Sivni–Amarkantak, and Nainpur–Shahpura, covering the central western, northwest–southeast, and central eastern parts, respectively, of the EDP (Fig. 4). They carried out flow-by-flow paleomagnetic analyses and have provided multiple polarity zones for the lava pile, in which the majority of the flows have reversed polarity. As a result, they established an N–R–N magnetostratigraphy for the EDP that can be correlated with 29N–28R–28N magnetic chron following the 40Ar/39Ar dating of some of the flows carried by Shrivastava et al. (2015). Strangely, it is clearly visible that the magnetostratigraphic framework (i.e. 29N–28R–28N) given by Pathak et al. (2016) cannot be correlated to a 30N–29R–29N classification (Fig. 2) assigned to the EDP by Vandamme and Courtillot (1992). Therefore, more paleomagnetic and radiometric studies involving thorough and extensive sampling are to be required to know the compatibility of these two magnetostratigraphic classifications. A few paleomagnetic investigations have been undertaken on the lava pile of the SP, which favor a 30N–29R–29N magnetostratigraphic framework (Paul et al., 2008).
6 Geochronology and chronostratigraphy
Absolute dating of the DVP has been the focus of numerous geochronologic studies because of its possible link to the Chicxulub impact and the K–Pg mass extinctions (Courtillot et al., 1986). As a result, 40K/40Ar, 40Ar/39Ar, 187Re/187Os and U/Pb techniques have been used to obtain absolute ages of its various sub-provinces (e.g., Schoene et al., 2015; Vandamme et al., 1991, Fig. 2; Table 2). The radiometric age database of the DVP generated by various workers, mainly employing the 40K/40Ar and 40Ar/39Ar dating techniques, have incessantly been subject to compilation, re-calculation and revision from time to time (e.g., Chenet et al., 2007; Courtillot et al., 1986; Pande, 2002). A comprehensive compilation of the existing absolute age database indicates (i) an episodic nature of eruption, (ii) that volcanic activity occurred between a range from 69 to 64 Ma ago, and (iii) that a maximum eruptive volume (~70%) occurred in a very short time span (<1 Ma), straddling the K–Pg boundary (Chenet et al., 2007; Renne et al., 2015). This age database is subject to many biases, such as analytical procedures, sample alteration, sample location, ages of standards (e.g., MMhb-1), plateau quality, potassium and calcium contents, argon recoil and whether ages are performed on whole rock samples or on mineral separates (Chenet et al., 2007).
Summary of published 40Ar/39Ar ages of the DVP.
Sample | Flow no. /Formation / Location | Age (Ma) | Error bar (Ma) |
Ages of the EDP (Shrivastava et al., 2015, error bar ±2σ) | |||
PLB F12/S12 | Flow no. 37 | 64.08 | 0.51 |
SK F10/S10 | Flow no. 31 | 64.41 | 0.65 |
NL F2/S2 | Flow no. 4 | 63.99 | 1.21 |
MK6 | Flow no. 4 | 65.18 | 1.22 |
MK2 | Flow no. 1 | 63.51 | 1.18 |
Age of the Western Ghats of the MDP (Richards et al. 2015 and references therein, error bar ±2σ) | |||
AM83-7 | Mahabaleshwar | 63.1 | 1.0 |
MB81-24 | Mahabaleshwar | 63.4 | 0.8 |
RA99.06 | Mahabaleshwar | 64.1 | 1.1 |
RA99.14 | Mahabaleshwar | 64.9 | 0.4 |
RA99.23 | Mahabaleshwar | 65.3 | 0.4 |
MAP-057 | Mahabaleshwar | 69.7 | 2.4 |
RA99.11 | Ambenali | 64.3 | 0.8 |
MB81-10 | Ambenali | 65.1 | 1.0 |
RA99.2 | Ambenali | 65.33 | 1.13 |
RA99.1B | Ambenali | 65.6 | 1.6 |
RA99.02 | Ambenali | 66.4 | 0.5 |
Mur 2 | Poladpur | 65.84 | 0.58 |
Ma 1 | Poladpur | 65.93 | 0.60 |
MB81-3/A | Poladpur | 68.04 | 0.8 |
JEB127 | Bhimashankar | 67.23 | 0.6 |
IG82-27 | Thakurvadi | 67.5 | 0.8 |
IG82-39 | Thakurvadi | 67.8 | 0.6 |
IG82-34 | Thakurvadi | 68.6 | 0.6 |
JEB-339Q | Neral | 66.4 | 1.0 |
TEM-004 | Neral | 68.0 | 1.4 |
JW7 | Igatpuri | 66.5 | 2.0 |
JW4 | Jawhar | 65.7 | 1.5 |
JW2 | Jawhar | 66.1 | 1.3 |
JW5 | Jawhar | 66.1 | 1.6 |
JW6 | Jawhar | 66.4 | 1.3 |
IGA-009Q | Jawhar | 66.4 | 1.2 |
IG82-4 | Jawhar | 67.8 | 0.6 |
Age of the MP (Schöbel et al., 2014, error bar ±1.96σ) | |||
S32p | 23.2111/74.4559 | 67.12 | 0.44 |
The lava pile of the Western Ghats has been intensively investigated (Fig. 2; Table 2). Out of 12 chemostratigraphic formations, the ages of the Khandala, Bushe, Panhala, and Desur formations are poorly assigned. Whereas the ages of the Kalsubai Subgroup are not very precise, the Poladpur, Ambenali and Mahabaleshwar formations of the Wai Subgroup have received maximum attention based on their relationship to the K–Pg boundary (Renne et al., 2015). The two 40Ar/39Ar ages of the Western Ghats lava pile carried out by Kaneoka (1980) and Duncan and Pyle (1988) suggested age ranges from 68 to 63 Ma and 68.5 to 66.6 Ma ago, respectively. Prior to 2000, it was concluded that ages of the MDP cluster at 65.5 ± 2.5 Ma (Hofmann et al., 2000; Vandamme et al., 1991). The most recent absolute age data obtained by applying unconventional high-precision 40Ar/39Ar and U/Pb (using zircon crystals) dating techniques has considerably enhanced the understanding of the onset, age and duration of volcanism. Hofmann et al. (2000) and Chenet et al., 2007 favored a short duration for the main volcanic eruption. Hofmann et al. (2000) carried out 40Ar/39Ar dating on mineral separates (plagioclase), whose samples were collected from the lava flows of the lower (Jawhar and Igatpuri formations) and a dyke present in the Poladpur Formation of the upper parts of the lava pile. They obtained mean ages of 65.4 ± 0.7 Ma and 65.2 ± 0.4 Ma for the lower and upper parts, respectively, of the lava pile. Following this, Chenet et al., 2007 used the 40K/40Ar dating technique to date mineral separates of the lava flows of the Jawhar, Poladpur, Ambenali, and Mahabaleshwar formations. While dating mineral separates, they noted that the mean ages range from 64.9 to 64.1 Ma ago from the basal Jawhar to the upper Mahabaleshwar formations with a span of at most 0.8 Ma. One of the analyzed samples, i.e. NA03 collected from the base of lava pile, has yielded an intriguing mean age of 67.4 ± 1.0 Ma. The NA03 sample-bearing lava unit is cumulatively 100 m in thickness and its horizontal flow field possesses a typical transitional magnetic polarity, peculiar flow morphology and lies below the Jawhar–Igatpuri formations. Further, a recognizable difference in erosion and the age of lava flows located below and above the NA03, together with the presence of faults, are also noted, which led Chenet et al., 2007 to place NA03 lava unit into a new Latifwadi Formation and to assign it to chron 31N/30R or 30R/30N. The presence of the Latifwadi Formation in the Western Ghats is to be considered as an indicative of an initial phase of volcanic eruption that took place ca. 2 to 3 Ma ago, prior to the main volcanic eruption, which may be correlated with the 30N polarity-bearing lava unit of the MP (see Schöbel et al., 2014). The ages obtained by 40Ar/39Ar (Hofmann et al., 2000) and 40K/40Ar (Chenet et al., 2007) dating methods cluster at 65.8 Ma and 64.8 Ma, respectively, and clearly show that a vast thickness of lava pile corresponds to chron 29R, and erupted in a short time span (<0.8 Ma) close to the K–Pg boundary. The U-Pb zircon geochronology data obtained after analyzing samples collected from the Jawhar, Ambenali and Mahabaleshwar formations shows an age range from 66.288 ± 0.027 to 65.535 ± 0.027 Ma ago for the trap rocks (Schoene et al., 2015). Based on this age range, Schoene et al. (2015) interpreted that the main volcanic phase started nearly 250 thousand years (kyr) prior to the K–Pg boundary, and a major portion of total eruptive volume (~70%) was emplaced in <1 Ma. While performing high-precision 40Ar/39Ar dating on mineral separates of the Jawhar, Igatpuri, Neral, Thakurvadi, Bhimashankar and Ambenali formations, Renne et al. (2015) obtained age ranges from 66.38 ± 0.05 to 66.20 ± 0.13 Ma ago. They combined their high precision 40Ar/39Ar age data with the U/Pb data of Schoene et al. (2015) and concluded that the main volcanic phase was not an isolated event, but an event genetically related to the Chicxulub impact. Further, they noted that the main phase of volcanic eruption took place within 50 kyr of the Chicxulub impact and the K–Pg boundary, thus, the seismic waves due to the impact would have suddenly accelerated the eruption rate of the Deccan volcanism.
The geochronologic data based on 40Ar/39Ar dating technique of the MP, yielding an age of 67.12 ± 0.44 Ma ago, favors the commencement of the Malwa flows during chron 30N (Schöbel et al., 2014). Using a similar dating method (40Ar/39Ar), Shrivastava et al. (2015) obtained an age range from 65 to 63 Ma, with a calculated mean age at 64.21 ± 0.33 Ma of the EDP. Further, they did not find any significant age differences from the base to the top of the EDP lava flows and, thus, concluded that the main portion of the EDP lava pile is significantly younger than the MDP and erupted after the K–Pg boundary. While carrying out 40Ar/39Ar dating of the Deccan basaltic-type dykes, exposed in the southernmost part of the DVP in Goa, Widdowson et al. (2000) obtained an age of 62.8 ± 0.2 Ma. The above age dates of Widdowson et al. (2000) and Shrivastava et al. (2015) clearly provide an indication of continuing volcanic activity both in the southern and northern margins of the DVP at some 1–2 Ma after the K–Pg boundary. The absolute mean ages of the lower (65.0 ± 1.1 Ma) and upper (64.5 ± 0.8 Ma) traps of a volcano-sedimentary sequence of the Rajahmundry estimated by 40Ar/39Ar methods also favored rapid eruption of the traps with the late phase of eruption post-dating the K–Pg boundary (Knight et al., 2003). Venkatesan et al., 1996 obtained 40Ar/39Ar ages of the Anjar flows that range from 67 to 63 Ma and inferred that the peak volcanic activity occurred prior to the K–Pg boundary by at least 2 Ma and also preceded it in the SP. A comprehensive summary of litho-, chemo-, chrono- and magnetostratigraphy is presented in Fig. 2.
Finally, the infra- and intertrappean beds associated with the DVP also provide an important relative age control for the duration of volcanism (Khosla and Sahni, 2003; Khosla and Verma, 2015). The presence of dinosaur taxa including skeletal material and nesting sites from the Lameta Formation shows that the DVP is not older than Maastrichtian. It is also supported by the occurrences of Maastrichtian foraminiferal assemblages recovered from the infratrappean well samples of Narsapur, Palakollu-A, Modi-A and Elamanchili-A, located on the southeastern coast of India. Biostratigraphicaly, the K–Pg boundary time constraints for the DVP have recently been obtained from the Upper Cretaceous to Lower Paleocene Deccan intertrappean beds exposed at Jhilmili, Central India, which have yielded a mixed biota comprising ostracods (Zonocypris viriensis, Neocyprideis raoi) and planktic foraminifers (Subbotina triloculinoides, Woodringina hornerstownensis, Parasubbotina pseudobulloides, Hedbergella cf. holmdelensis etc.) of freshwater to brackish-marine environments (Keller et al., 2009; Khosla, 2015). Additionally, the shallow marine intertrappean beds of Upper Cretaceous to Lower Paleocene age containing ostracods (Bairdia beraguaensis, Cytheridella rajahmundryensis), planktic foraminifers (A. mayaroensis, Globotruncana arca, Rugoglobigerina rugosa), and calcareous nanoplanktons (Hornibrookina sp., Cruciplacolithus primus) have also been recorded from the Krishna–Godavari basin, southeastern India (Keller et al., 2008).
Deccan volcanic eruptions may have assumed a noteworthy job in its second phase (uppermost Maastrichtian), which commenced in 29R, and finished with the K–Pg boundary mass elimination (Keller et al., 2009, 2010; Khosla, 2015). Schulte et al. (2010) suggested that the Late Cretaceous extinctions were credited to a lone event and have recorded impact spherules in the Maastrichtian deposits in Texas and Mexico, The short-term destruction circumstance recorded by Schulte et al. (2010) has not stood up to the unlimited examinations of how marine and terrestrial vertebrates creatures fared toward the end of Cretaceous. Courtillot and Fluteau (2010) contradicted the viewpoint of Schulte et al. (2010) as it does not give reasonable idea of the volcanic hypothesis. They measured that total SO2 released by the Deccan lavas at approximately 10,000 Gigatons, which is of a smaller amount by a factor of 50 than that in work of Schulte et al. Nonetheless, Courtillot and Fluteau (2010) additionally showed that imbuement of SO2 by a lone basaltic pulse may have had a climatic effect like the Chicxulub affect. Based on biostratigraphical, magnetostratigraphical, and iridium proxies, Keller et al. (2004) reported enough evidence from a core (Yaxcopoil-1), bored inside the Chicxulub hole, demonstrating that this effect originated before the K–Pg boundary by 300,000 years and along these lines did not cause the end-Cretaceous mass eradication, as ordinarily accepted. Further, it is expressed that there is no record of iridium inconsistency in union with the Chicxulub ejecta, and impact spherules have never been perceived in the iridium supplemented the K–Pg layer in Mexico (Keller et al., 2010). Ongoing work on Deccan volcanism obviously demonstrates that the principle mass termination is connected with the real period of the Deccan emissions in C29R (Keller et al., 2010).
7 DVP as a prominent geodynamic feature
The DVP is an unique geodynamic feature of the Indian plate that preserves significant clues to understand the earth's internal dynamics and movement of the tectonic plates. A majority of flood basaltic provinces across the globe usually occur in intraplate settings and their origins are thought to be associated with deep mantle plumes (Courtillot and Renne, 2003; Courtillot et al., 2015; Richards et al., 1989). These plumes are supposed to be thermal features starting from the transition zone in the lower mantle above the core–mantle boundary (Courtillot and Renne, 2003; Courtillot et al., 2003). The plumes are characterized by a mushroom-shaped head and a long, thin centrally located tail. Their immobile lower mantle upwelling sites on the surface constitute the hotspots that usually preserve time progressive volcanic trails as lithospheric plates crossing over them. In this context, the DVP is special as it is related to the Réunion hotspot of the African plate (Hooper, 1999). The massive basaltic eruption of the DVP resulted when the Indian plate was located at the today's position of Réunion island in the Indian Ocean. It is recorded that as the Indian plate progressed after isolating from the Seychelles, it left behind a track of the Réunion hotspot constituting the Chagos–Maldive–Laccadive islands, Laccadive Ridge, the Mascarene plateau, and the islands of Mauritius in the western Indian Ocean. The Réunion hotspot is still active and age data of track favor a time progressive age relationship between the Réunion island and the DVP, which invariably increases from 0.00 to around 67 Ma ago, respectively (Mahoney, 1988).
Numerous studies strongly favor a deep plume origin of the DVP related to the Réunion hotspot because it possesses high helium isotopes; a large volume of eruption took place relatively in a very short time span and the existence of a linear chain of age progressive hotspot track from the Réunion to the DVP (Courtillot et al., 2003; Sen, 2001). It has been observed that the Ambenali Formation of the DVP is the most widespread and the least contaminated chemo-unit closely related to the Réunion lavas, and its isotopic and chemical signatures favor derivation of magma by extensive partial melting of the Deccan-like basaltic rocks by the deep mantle plume (Mahoney et al., 2000; Peng et al., 1994; Sen, 2001). The topographic map of the 29R/29N boundary indicates a domal structure having an amplitude of more than 1500 m, fragmented by rift structures, and the arrangement of contours show the presence of antiforms parallel to the Narmada–Son rift system and north–south antiforms parallel to the Western Ghats (Fig. 5). These are pre-existing structures of trap flows, which got reactivated by the impingement of the Réunion plume when the Indian plate passing over it and hence, provide a line of evidence favoring the Réunion plume origin to the DVP (Vandamme and Courtillot, 1992).
8 Conclusion
The DVP has a prominent place in geology, since it has been serving as a natural field laboratory to propose, test, and understand hypotheses useful for knowing the hidden mechanisms of various processes related to earth system dynamics and their interrelations with the biosphere and the atmosphere. Its spatial extent and volume are still a matter of debate. The older lithostratigraphic classification proposed way back in the 1875, showing older traps to the east and younger to the west, is no longer considered valid. During the late 1900s, separate lithostratigraphic classifications, each for the MDP and the EDP, have been formulated. Many workers have been casting doubt on the validity of these classifications. A rapid advancement has been seen in the chemostratigraphy of the MDP; as a consequence, a sound chemostratigraphic classification consisting of 12 distinct formations belonging to three subgroups has been established for the Western Ghats. Attempts have also been made to find chemo-units in other sub-provinces and to correlate them with the standard units of the Western Ghats. It was found that, to a large extent, a precise correlation is untenable due to various reasons like horizontal flows, kinds of tectonic control, nature of the magma, and content of crustal contamination. A 30N–29R–29N magnetostratigraphy for the MDP lava pile is well established. The magnetostratigraphic framework of the EDP, MP and SP needs more efforts. Absolute age data favor a 69 to 64 Ma ago duration of the DVP with maximum lava emplaced at chron 29R, coinciding with the K–Pg boundary. The geotectonic, geochemical, and paleomagnetic data strongly reflect that the DVP is a product of the Réunion mantle plume.
It has been seen that a majority of work on the DVP has been carried out in isolation, focusing on the set objectives of the research. Nonetheless, a majority of research articles associated with the DVP always highlight its concern in the context of mantle plumes, drift of the Indian plate, K–Pg boundary, end-Cretaceous mass extinction, climate alteration, and biotic evolution. Therefore, future research work requires a focused attention where multiple aspects of any lava pile consisting of its age, stratigraphy, biotic evolution, paleoenvironments of trap sediments and environmental impact of flows should be examined holistically.
Dedication
We are pleased to dedicate this paper to Prof. Ashok Sahni, an eminent leader of Indian Geology, especially in the fields of paleontology and stratigraphy and an enthusiast of the Deccan volcanism. Prof. Sahni spent his whole life working on Late Cretaceous biota of peninsular India, Eocene biota of Gujarat, and Cenozoic vertebrates of the Himalaya. Numerous researchers have benefited greatly from his scholarly works and expert advice, which has been invaluable and globally, deeply acknowledged. The authors have two generations link with him, one of the authors (AK) is his doctoral student and the other (OV) is a doctoral student of one of his earlier PhD students.
Acknowledgements
We are thankful to Prof. Vincent Courtillot for showing interest and inviting us to contribute this article for C. R. Geoscience. One of us (Ashu Khosla) is grateful to Vincent Courtillot for the for initial scrutiny of the manuscript and comments with linguistic improvements to the manuscript, the necessary help, and for guiding him regarding the stratigraphic aspects of Deccan Traps. We also thank the reviewers: S.G. Lucas (America) and two anonymous reviewers for constructive criticism and insightful remarks that helped us in improving the manuscript. The Department of Science and Technology, Govt. of India is acknowledged for funding to OV (grant number SR/FTP/ES-33/2008), and to AK (grant SR/S4/ES-382/2008 and Purse Project, Panjab University, Chandigarh).