Version abrégée
1 Introduction
En Nouvelle-Calédonie, les sols développés sur roches ultrabasiques sont exceptionnellement riches en Fe et en métaux de transition tels que Mn, Ni, Cr et Co [9,11]. Les oxydes de Fe et de Mn sont à la fois des réservoirs et des puits de métaux [8,12], qui jouent un rôle important en contrôlant leur disponibilité [5]. Becquer et al. [1] ont observé une plus grande disponibilité de Ni dans les sols de plaine soumis à un engorgement. La réduction des oxydes de Fe et Mn par l'intervention de bactéries ferri- et mangani-réductrices [3] peut augmenter la solubilisation des métaux. Même si la gœthite est le principal minéral dans ces sols, des phases mineures comme les oxydes de Mn, plus réductibles que les oxydes de Fe, peuvent contrôler la disponibilité et la mobilité des éléments en traces.
Les objectifs de cette note sont (i) d'étudier, en conditions réductrices, la dissolution chimique et bactérienne des oxydes de Mn naturels et la solubilisation des éléments associés et (ii) d'identifier l'oxyde de Mn impliqué.
2 Matériel et méthodes
2.1 Caractérisation du sol
Un échantillon de sub-surface (4–10 cm) a été prélevé dans un Ferralsol de plaine décrit par Becquer et al. [1]. Après tamisage à 2 mm, une partie est séchée pour les analyses et une autre conservée humide (à l'obscurité et à 4 °C) jusqu'au début des expériences.
C et N total du sol sont dosés au CHN 1108 Carlo Erba. Les teneurs totales en Fe et en Mn sont déterminées par ICP–AES, après fusion de l'échantillon dans un mélange métaborate–tétraborate de lithium à 1000 °C. Les teneurs totales en Ni, Co et Cr sont mesurées après digestion diacide en four à micro-onde.
2.2 Dissolution chimique
La dissolution des oxydes de Mn a été réalisée selon la méthode de Chao [4] (1 g de sol, 25 ml NH2OH·HCl 0,1 M, pH 2, 30 min à 20 °C) et celle des oxydes de Fe a été réalisée selon Holmgren [6] (125 mg de sol, 35 ml de citrate–bicarbonate, 350 mg de dithionite, 20 °C, 5 j). Fe, Al, Mn, Ni et Co sont dosés dans les surnageants en ICP–AES.
2.3 Dissolution bactérienne
Des incubations ont été réalisées avec 50 g de sol (ou 25 g pour les témoins) et 750 ml (ou 375 ml) de solution de glucose à 1 g l−1 dans des flacons de 1000 ml (ou 500 ml) fermés hermétiquement. Le sol est incubé en présence ou absence (ajout de thimerosal à 1 g l−1) de micro-organismes autochtones pour distinguer les processus d'origine, soit microbiologique, soit physico-chimique. Les incubations sont conduites en anaérobiose, à l'obscurité à 28 °C pendant 11 semaines. Différents prélèvements sont réalisés au cours du temps ; après filtration, les métaux en solution sont dosés en ICP–AES et la spéciation de Fe et Mn est réalisée par colorimétrie [10].
2.4 Observations en MET et microanalyses
Les échantillons séchés sont mis en suspension dans de l'éthanol sous ultra-sons. Une goutte de suspension est évaporée sur une grille de cuivre et la préparation est observée en microscopie électronique à transmission (MET). L'analyse élémentaire des particules est effectuée en spectrométrie de dispersion d'énergie des rayons X.
3 Résultats et discussion
3.1 Caractéristiques du sol
Le sol est très riche en Fe, Mn, Ni, et Co (Tableau 1). Les oxydes de Fe sont dominés par la gœthite, mais des traces d'hématite ont été observées [2]. Des revêtements ferro-manganiques, visibles dans le profil de sol [1], suggèrent l'existence de processus d'oxydo-réduction. Le pH du sol est acide, la teneur en MO est voisine de 5 %.
Total amounts and percentages (% in parenthesis) of metals solubilised in the liquid medium at the maximum rate of chemical and bacterial reduction vs total metal content of the soil sample.
Quantités maximales et proportions (% de la teneur totale) de métaux solubilisés dans le milieu au cours de la réduction chimique et bactérienne.
Fe | Mn | Co | Ni | Al | |
mg kg−1 (%) | |||||
Total content | 394 000 | 5650 | 630 | 9430 | 33 000 |
Solubilised amounts | |||||
DCB | 390 300 | 4960 | 710 | 8620 | 28 390 |
(99.1) | (87.8) | (113) | (91.4) | (86.0) | |
Hydroxylamine hydrochloride | 1535 | 2715 | 335 | 86 | 216 |
(0.39) | (48.1) | (53.2) | (0.91) | (0.65) | |
Bacterial reduction∗ | 2959 | 1614 | 49 | 37 | 0.1 |
(0.75) | (28.6) | (7.78) | (0.39) | (0.00) |
3.2 Réduction chimique
Plus de 85 % de Fe, Mn, Co et Ni sont dissous par le CBD (Tableau 1). L'hydroxylamine solubilise 50 % de Mn et Co et moins de 1 % de Fe et Ni. L'hydroxylamine solubilise plus spécifiquement les oxydes de Mn, mais une dissolution très limitée des oxydes de Fe et des minéraux acido-solubles peut se manifester [4]. Ces résultats soulignent que Mn et Co sont donc présents essentiellement dans les oxydes de Mn, alors que Fe et Ni le sont dans les oxydes de Fe. On peut considérer que, dans les oxydes de fer substitués, Ni et Fe sont solubilisés de façon congruente [13]. En considérant que la plus grande part de Ni est associée aux oxydes de Fe avec un rapport Ni/Fe de 0,02, la quantité de Ni associé aux oxydes de Fe dissous par l'hydroxylamine devrait être de 37 mg kg−1, si ce réactif solubilisait les oxydes de fer. Or, cette quantité estimée est très inférieure à la quantité extraite (86 mg kg−1, Tableau 1). On peut donc considérer que 40 % du Ni extrait par l'hydroxylamine est associé aux oxydes de Fe et 60 % aux oxydes de Mn.
3.3 Réduction bactérienne
Quantin et al. [10] ont montré une forte corrélation entre la biodégradation des matières organiques du sol et la réduction des oxydes en conditions anaérobies, en soulignant que l'addition de glucose exerce un effet stimulant sur la dissolution des métaux [10]. En conditions biotiques, la solubilisation de Mn augmente pendant 24 j (97±2 mg l−1), puis se stabilise, alors qu'elle reste très réduite en conditions abiotiques (Fig. 1a). La proportion de Mn dissous atteint 28 % du Mn total. Seulement 20 % du Mn est sous forme ionique libre (Mn2+), quantité estimée par formation d'un complexe coloré [10]. Une grande part du Mn est complexée en solution par les composés issus de la fermentation du glucose (acides acétique, propionique, butyrique) et de la biodégradation des matières organiques du sol [10]. La solubilisation de Fe augmente de façon continue jusqu'à 40 j environ, pour atteindre moins de 0,8 % du fer total. La solubilisation de Co, sous influence microbienne, montre une évolution en deux étapes : une solubilisation rapide pendant 24 j, qui atteint 7 % du Co total, puis une diminution lente (Fig. 1b). Le même comportement est observé pour Ni, avec un maximum de solubilisation à 14 j. L'insolubilisation de Co et Ni correspond sans doute à des phénomènes de précipitation et coprécipitation, incluant des phénomènes de sorption et la réorganisation des phases solides.
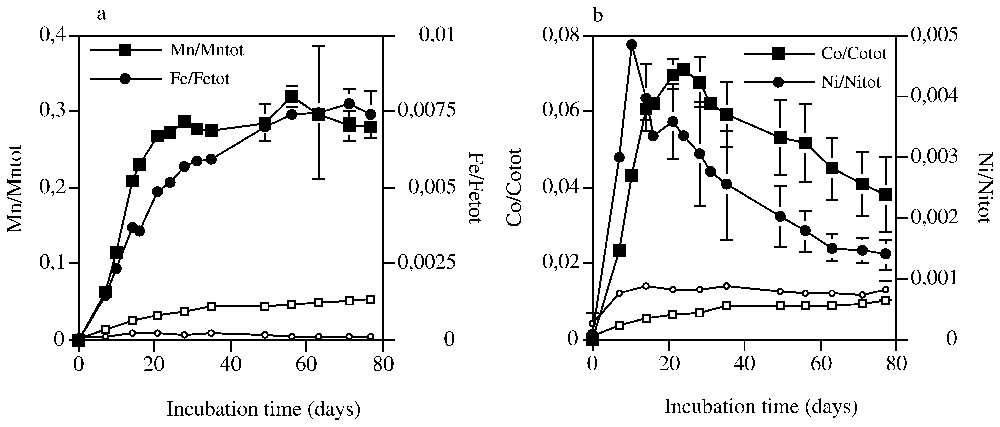
Solubilisation rates of Mn and Fe (a) and Co and Ni (b) during biotic (filled symbols) and abiotic (opened symbols) incubations. Non-visible bars of standard deviations are included in spots on the curves.
Taux de solubilisation de Mn et Fe (a) et Co et Ni (b) au cours des incubations en conditions biotiques (symboles pleins) et abiotiques (symboles ouverts). Les barres d'erreur non visibles sont inclues dans les figurés.
Ces expériences montrent que la communauté bactérienne anaérobie de ce sol est fortement impliquée dans la dissolution réductive de minéraux contenant Mn, Co, Ni et Fe. Ces résultats soulignent bien qu'un oxyde de Fe est fortement altéré par l'activité des bactéries ferri-réductrices [10], alors qu'il n'est pas dissous par l'hydroxylamine. Les bactéries ne solubilisent que 60 % du Mn dissous par l'hydroxylamine. La totalité du Co et Ni des oxydes de Mn n'est pas dissoute par les bactéries dans les conditions expérimentales adoptées, sans doute par suite de l'insuffisance de quantités d'énergie disponible sous forme de matières organiques métabolisables.
3.4 Caractérisation de la source de métaux
Des revêtements d'oxydes de Mn sont observés sur le profil de sol, mais ne sont pas détectables par DRX sur la terre fine. Néanmoins, les observations en MET confirment la présence d'un oxyde de Mn dans les échantillons de sol (Fig. 2), qui présente un aspect de papier froissé et une structure aciculaire. L'analyse élémentaire en EDXS montre que ce minéral est constitué principalement de Mn, Co et Al, avec 4,3 % de Ni (Tableau 2). Cette observation peut être rapprochée de celles de Manceau et al. [7], qui ont décrit des oxydes de Mn interstratifiés avec des feuillets de Mn(IV), Co(III) et Ni(II), Al(III) dans les horizons saprolitiques développés sur roches ultrabasiques de Nouvelle-Calédonie. Ce composé, caractérisé comme un oxyde mixte de lithiophorite–asbolane, a une composition très variable [7].

TEM micrograph of Mn-oxide particles.
Photographie MET des particules d'oxydes de Mn.
EDXS elemental analysis of Mn-oxide particles.
Analyse élémentaire en EDXS des particules d'oxydes de Mn.
Element | Atomic % |
Al | 11.9 ± 3.3 |
Si | 12.2 ± 9.9 |
Mn | 47.1 ± 1.4 |
Fe | 7.28 ± 2.75 |
Co | 15.3 ± 8.4 |
Ni | 4.30 ± 2.25 |
4 Conclusion
Les Geric Ferralsols de Nouvelle-Calédonie dérivés de péridotites présentent des teneurs exceptionnelles en métaux tels que Ni, Cr et Co. Les expériences de dissolution chimique montrent que Co est principalement associé aux oxydes de Mn et Ni aux oxydes de Fe. Au cours d'incubations en conditions anaérobies, l'activité bactérienne solubilise respectivement et au maximum 28,6, 7,8 et 0,4 % du Mn, Co et Ni total en 25 jours. Des observations en MET montrent la présence d'un oxyde de Mn porteur de Co, Ni et Al. Bien que la majeure partie de Ni soit associée aux oxydes de Fe, une phase minérale mineure d'oxyde mixte de Mn à feuillets de lithiophorite–asbolane apparaı̂t comme une source majeure de métaux biodisponibles. Dans les sols de plaine soumis à engorgement temporaire, ces oxydes de Mn, très sensibles aux variations des conditions d'oxydo-réduction, en raison du potentiel élevé du couple Mn4+/Mn2+, peuvent être réduits, à la fois par des processus chimiques et bactériens, plus rapidement et efficacement que les oxydes de Fe. Cette phase minéralogique mineure peut donc être la source principale de métaux facilement biodisponibles en conditions naturelles.
1 Introduction
In New Caledonia, soils originating from ultramafic rocks are exceptionally rich in Fe and in other transition metals, especially Mn, Ni, Cr and Co [9,11]. Fe- and Mn-oxides are major reservoirs and sinks for metals [8,12] and play an important role in controlling their availability [5]. Previous results reported a higher Ni availability in plain soils subjected to waterlogging [1]. Therefore, the reduction processes of Fe- and Mn-oxides may increase the metal release, with the possible involvement of Fe- and Mn-reducing bacteria [3]. Even though goethite is the main constituent of these soils, minor phases as Mn-oxides, that are more reducible than Fe-oxides, could control the trace element availability and their mobility in the environment.
The aims of the present paper are (i) to study the chemical and bacterial dissolution of Mn-oxide and the release of associated trace metals under reductive conditions and (ii) to identify the Mn-oxide mineral involved.
2 Material and methods
2.1 Soil characterisation
A subsurface soil sample (4–10 cm) was collected in a plain Ferralsol described elsewhere [1]. It was sieved at 2 mm, a part was air-dried for analysis and the other was kept moist (in the dark at 4 °C) until experiments were initiated.
Total C and N in the solid phase were quantified using a CHN 1108 Carlo Erba analyser. Total mineral elements of the sample were measured by ICP–AES (Jobin-Yvon 238) after dissolution in a lithium metaborate–tetraborate mixture at 1000 °C for Fe and Mn and a diacid digestion (2:1 concentrated HNO3:HCl ratio) in a microwave oven for Ni, Cr and Co.
2.2 Chemical dissolution experiments
The hydroxylamine hydrochloride procedure of Chao [4] was used for Mn oxide extractions. One gram of soil was stirred with 25 ml of 0.1 M NH2OH·HCl (pH = 2) for 30 min at 20 °C. Dissolution of iron oxides was carried out using a modification of the dithionite–citrate–bicarbonate (DCB) deferrification method of Holmgren [6]. 125 mg of soil sample were placed in centrifuge tubes with (or without) 350 mg of dithionite and 35 ml of citrate–bicarbonate solution (CB) and mixed gently in an end-over-end shaker at 20 °C for five days. Then, the samples were centrifuged and the supernatant analysed for Fe, Al, Mn, Ni and Co by ICP–AES.
2.3 Bacterial dissolution experiments
Batch incubations were performed with 50 g (or 25 g for controls) soil in hermetically sealed 1000-ml (or 500-ml) plasma bottles, supplemented with 750 ml (or 375 ml) of glucose solution (1 g l−1). Flasks with soil were incubated in the presence or absence of indigenous microorganisms to distinguish microbial from physicochemical processes. Abiotic conditions were obtained by addition of 1 g l−1 of Na-thimerosal. Incubations were carried out anaerobically, with four replicates per treatment. The batch cultures were incubated in the dark at 28 °C for 11 weeks, without shaking except just before sampling.
Metal contents in solution were monitored at various times by collecting 15 ml (or 10 ml for controls) of suspension under sterile conditions. Aliquots of supernatants were filtered (0.2 μm membranes, cellulose acetate, Sartorius) and analysed by ICP–AES for total metal content. Fe and Mn speciation in solution was studied colorimetrically as described elsewhere [10]. pH and Eh of the medium were also measured.
2.4 TEM observations and EDXS analysis
Air-dried samples were suspended in ethanol under ultrasonication. A drop of suspension was then evaporated on a carbon-coated copper grid, and the preparation was observed with a Transmission Electron Microscope (Philips CM 20) at an accelerating voltage of 200 kV. The microscope was equipped with an EDAX energy dispersive X-ray spectrometer and provided elemental analysis of particles.
3 Results and discussion
3.1 Soil characterisation
The soil was very rich in iron and relatively rich in Mn, Ni and Co (Table 1). The Fe-oxides were dominated by goethite, but hematite traces were observed [2]. The ferro-manganese coatings observed in the soil profile [1] suggested the existence of oxidation-reduction processes. However, as noticed elsewhere [2], the gleyic colour pattern of the soil is weak, indicating that reduction processes are probably slight. Soil pH was acidic. The organic matter content reached 5% and the C:N ratio was 22. Further details of the chemical and mineralogical characteristics of the soil are presented by Becquer et al. [2].
3.2 Chemical reduction of Fe- and Mn-oxides
More than 85% of total Fe, Mn, Co and Ni were dissolved by DCB reagent (Table 1). Hydroxylamine hydrochloride solubilised 50% of total Mn and Co and less than 1% of total Fe and Ni. These results suggested that Mn and Co were mainly associated in Mn-oxides and Fe and Ni in Fe-oxides, because hydroxylamine hydrochloride dissolves specifically manganese oxides and only very low and limited amount of both iron oxides [4] and acido-soluble minerals. It can be considered that Ni included in substituted ferric oxides is dissolved congruently with Fe [13]. If we considered that all the solubilised Ni was originated from Fe-oxides having a Ni:Fe ratio of 0.02 (w/w), the amount of Ni extracted with hydroxylamine hydrochloride should amount to 37 mg kg−1 according to the amount of Fe extracted by this reagent. Such a theoretically calculated amount is markedly lower than the amount of 86 mg kg−1 really extracted with hydroxylamine hydrochloride (Table 1). Therefore, it can be proposed that nearly 40% of Ni extracted with hydroxylamine hydrochloride is associated with Fe oxide and 60% with Mn oxide.
3.3 Bacterial reduction of Fe- and Mn-oxides
A previous study has shown a correlation between organic matter biodegradation and oxide reduction processes under anaerobic conditions [10] and that the addition of an easily biodegradable compound like glucose increased the dissolution of the metals [10]. In abiotic treatment, Mn solubilisation was very low (Fig. 1a) and corresponded to the Mn exchanged with Na from thimerosal. In biotic conditions, Mn solubilisation increased continuously during the first 24 days, reaching 97±2 mg l−1, then a stationary phase occurred (Fig. 1a). The proportion of total dissolved Mn reached then 28% of total Mn. Free ionic Mn2+, determined by the formation of soluble coloured complex, represented less than 20% of dissolved Mn, suggesting that a large part was complexed in solution by organic compounds (acetic, propionic, butyric acids...) originated from glucose fermentation and soil organic matter biodegradation [10]. Organic acids (acetic, propionic and butyric acids) were produced by bacteria in complement of other organic dissolved matter as fulvic-like compounds [10]. Iron reduction increased over time to reach less than 0.7% of total iron (i.e., 268±27 mg l−1) in biotic treatment, whereas no detectable reduction appeared in abiotic controls (Fig. 1a).
Co and Ni solubilisation was very low in controls (1% and 0.9‰ of Co and Ni, respectively) and attributed to metal exchange with Na of the thimerosal (Fig. 1b). In biotic conditions, Co solubilisation showed a two-step process: first an increase until day 24, when solubilisation reached 7% of the total Co, and then a slow decrease. For Ni, the same trend was observed with a maximum solubilisation occurring at day 14. The non-solubilisation of Co and Ni may correspond to an adsorption–precipitation and coprecipitation phenomenon, including sorption and reorganisation of the solid compartments.
These experiments showed that bacteria growing in these anaerobic conditions [10] are significantly involved in the dissolution by reduction of Fe and Mn-oxides containing Co and Ni. Comparison with chemical dissolution showed that more Fe is solubilised by bacterial activity than by hydroxylamine. This suggests that crystallised Fe-oxide can be weathered by Fe-reducing bacteria [10], whereas it is not reduced by hydroxylamine. However, Fe-oxide solubilisation increased relatively slower and longer than Mn solubilisation (Fig. 1a), when the Eh of the solution was very low, whereas Mn-oxide solubilisation increased rapidly, from the beginning of the incubation, during Eh decrease (results not shown). The different trends of Mn- and Fe-oxides are more obvious when the soil organic matter is the only source of carbon and energy [10]. The lack of morphological evidence in the soil profile of severe reducing conditions lead us to consider that under natural conditions, Mn-oxides are more subjected to reduction process than Fe-ones. Only 60% of Mn solubilised by hydroxylamine was solubilised by bacterial reducing activity. Therefore all the Co and Ni associated to Mn-oxide was not microbiologically solubilised under these experimental conditions. Limitation can be due to the lack in energy source available as metabolisable organic matter to microorganisms.
3.4 Characterisation of the Mn-oxide involved in Co and Ni release
Mn-oxide coatings that were observed in the soil profile were not detectable by powder X-ray diffraction on the bulk soil. Nevertheless, TEM observation revealed the presence of Mn-oxide in the sample (Fig. 2). This Mn-oxide is organised as screwed paper and acicular elementary particles. EDXS spectra showed that this mineral is composed mainly by Mn, Co and Al with 15.3% of Co and 4.3% of Ni (Table 2). Such observation can be related to the results of Manceau et al. [7], who observed Mn-oxides with large variation of their Ni, Co and Al contents in the saprolitic horizons on the ultramafic rocks from New Caledonia. They described these compounds as an irregular intergrowth of Mn(IV), Co(III) and Ni(II), Al(III) sheets, which they called lithiophorite–asbolane mixed layers Mn-oxide.
4 Conclusion
The Geric Ferralsols derived from New Caledonian peridotites are all anomalously rich in trace metals such as Ni, Cr and Co. Chemical dissolution experiments show that Co is mainly associated to Mn-oxides and Ni to Fe-oxides. During biotic incubations under anaerobic conditions promoting bacterial growth and activity, 28.6, 7.8 and 0.4% of total Mn, Co and Ni of the soil, respectively, are solubilised after 25 days. TEM observations show the presence of a CoNiAl-bearing Mn-oxide. Although the largest part of Ni is associated with iron oxides, this minor mineralogical phase called lithiophorite–asbolane mixed layers Mn-oxide appears to be the main source of bioavailable metals in the lowlands subjected to temporarily waterlogging. Mn-oxides are very sensitive to redox conditions, as expected from the high reduction potential of Mn4+/Mn2+. During waterlogging, Mn-oxides could be reduced by chemical and bacterial processes, more easily than Fe-oxides. Bacterial reduction process appears to be very prominent when energetic sources are available as biodegradable organic matter.
Acknowledgments
The authors are grateful to Dr J. Ghanbaja (‘Service commun de microscopie électronique à transmission’, University Henri-Poincaré, Nancy-1, France) for TEM observations and EDXS analysis.