1 Introduction
Theoretical and analogue modeling of orogenic wedges suggests that extensional processes may be triggered by internal wedge dynamics, being controlled by depth-dependent wedge rheology and characteristics of the basal detachment (Dahlen, 1984; Davis et al., 1983; Platt, 1986; Willet, 1999). Extension coeval with or postdating shortening is observed in a wide range of convergent margin settings (Dewey, 1988); besides exerting a major control on the structure and seismicity of numerous mountain belts, this process is well known to produce tectonic exhumation. The latter phenomenon has been extensively studied over the past several decades, and there is abundant evidence that normal faulting triggers exhumation of mid-crustal rocks in various tectonic settings. Although less studied, tectonic exhumation occurs at shallow crustal levels in fold-and-thrust belts. These relatively shallow exhumation phenomena play a fundamental role in controlling the topography and the drainage evolution of mountain belts. Their study requires the integration of geological and geomorphic information with low-T thermochronometry (Zattin et al., 2002; Mazzoli et al., 2008). In this article, we apply such an integrated approach to the Western Outer Carpathians, a classic thin-skinned orogenic wedge that developed mainly in Miocene times by off-scraping of sedimentary rocks from the hinge of the retreating downgoing lithosphere (Swierczewska and Tokarski, 1998; Behrmann et al., 2000; Oszczypko, 2004; Nemcok et al., 2006a; Doglioni et al., 2006).
Besides field mapping and structural analysis, apatite fission track (AFT) thermochronometry was performed, integrating preexisting data sets (Anczkiewicz and Swierczewska, 2008). This allowed us to obtain a comprehensive picture of sedimentary and tectonic burial, and of the cooling history of the Polish Outer Carpathians. Although thermal and thermochronometric indicators suggest that outcropping rock units suffered significant tectonic burial, with maximum paleotemperatures ranging between 75 and 200 °C (Anczkiewicz and Swierczewska, 2008; Swierczewska, 2005), modes and timing of exhumation in the Polish Outer Carpathians are still poorly constrained. In particular, the role of tectonic exhumation is generally overlooked, in favor of erosion (Swierczewska, 2005). On the other hand, it is well established that the late stages of the tectonic evolution of the Western Outer Carpathians are controlled by extension of variable magnitude and orientation, related to post-orogenic collapse (Zuchiewicz, 2001). Our results and interpretations, emphasizing the role of extensional tectonics – related with orogenic wedge dynamics and gravitational collapse – in controlling exhumation processes, shed new light on this key area of the Alpine–Carpathian orogen.
2 Tectonic setting
The Polish sector of the Carpathian mountain belt constitutes the northernmost part of the whole orogen (Fig. 1a). This is traditionally subdivided into two main parts, known as Inner and Outer Carpathians, being separated by the Pieniny Klippen Belt, a narrow zone of intensely deformed and sheared Mesozoic to Paleogene rocks (Birkenmajer, 2001). The Polish segment of the Outer Carpathians is commonly interpreted as an accretionary wedge – composed of Upper Jurassic to Lower Miocene sedimentary successions – overthrusting Miocene siliciclastics of the Carpathian Foredeep. Stratigraphic evidence indicates that thrusting terminated diachronously along the Carpathian front – being progressively younger towards the east (Nemcok et al., 2006a) – while fault-controlled ‘intramontane’ basins developed on top of the orogenic wedge in Neogene-Quaternary times (Birkenmajer, 1978; Tokarski, 1978). In the study area (Fig. 1b), thrusting is commonly interpreted to have ceased at ca. 11 Ma (Nemcok et al., 2006a; Garecka and Jugowiec, 1999). South of the frontal thrust sheets (Skole and Borislav-Pokuttia Units), the Magura Unit constitutes the structurally uppermost tectonic element of the Outer Carpathian orogenic wedge. It forms a roof sequence overriding a series of tectonic units – known as Dukla, Silesian and Subsilesian Units – that are also exposed in a series of tectonic windows. The tectonic contacts separating these units are commonly portrayed as thrust faults. However, recent geological mapping (Jankowski, 2004), as well as our new field survey, point out the occurrence of younger-on-older, mainly south dipping tectonic contacts that are best interpreted as extensional faults (Fig. 1b–c) rather than out-of-sequence thrust contacts (Nemcok et al., 2000; Nemcok et al., 2006b). In most instances, poor exposure of main tectonic contacts effectively hinders detailed kinematic analyses, and information on shear senses is generally lacking in the existing literature. Our fieldwork allowed us to unravel significant reworking of the preexisting folds and thrusts by extensional structures associated with orogen-normal horizontal stretching (Fig. 2). This is shown, for instance, by the intensely deformed Oligocene strata of the Silesian Unit exposed in the footwall to the Magura Unit within the Mszana Dolna tectonic window (sites b and c in Fig. 1b). These strata (Krosno Fm) display extensional reworking – in the form of S dipping extensional shears – of a preexisting, well-developed S-C fabric associated with top-to-the-north sense of shear, the latter being consistent with the original thrust-related emplacement of the Magura Unit. Extensional structures are well developed also in the Kleczany area (site f in Fig. 1b). Here, a hard-linked extensional fault system, including SSW dipping normal faults and mainly NNE trending, oblique-slip transfer faults, is exposed in thick-bedded sandstones of the Krosno Fm (Silesian Unit) located in the footwall to the Magura Unit. Although most post-thrusting normal faults dip to the south, north dipping extensional shear zones also occur (Figs. 1b, 2). The horizontal extension pointed out in this study (Fig. 2) is consistent with NNE directed, orogen-normal extension representing the latest deformation event in the Silesian Unit according to (Rubinkiewicz, 2007).

(a) Tectonic sketch map showing subdivision into Inner and Outer Carpathians, separated by the Pieniny Klippen Belt (PKB), and location of study area. (b) Geological sketch map of the Polish Carpathians (based on (Jankowski, 2004)), integrated with new field surveys), showing location of regional cross-section X-X’, sites of structural analysis (blue dots) and sample location (red dots). AFT cooling ages for the Outer Carpathians, including data from (Anczkiewicz and Swierczewska, 2008)Anczkiewicz and Swierczewska, 2008, are shown in Ma ± 1σ. Cretaceous cooling age (75.7 ± 12.8) is from sample PL7, showing no AFT annealing; for all other samples, showing total annealing of AFT, the data represent exhumation ages (cooling through the isotherm ∼110 °C). (c) Geological cross-section, constructed by the integration of surface geology with available well logs and geophysical information on basement structure (Oszczypko, 1998). Masquer
(a) Tectonic sketch map showing subdivision into Inner and Outer Carpathians, separated by the Pieniny Klippen Belt (PKB), and location of study area. (b) Geological sketch map of the Polish Carpathians (based on (Jankowski, 2004)), integrated with new field ... Lire la suite
(a) Schéma structural simplifié démontrant la subdivision en Carpathes internes et externes, séparées par la Zone des Klippes de Pieniny, et emplacement de la zone d’étude. (b) Carte géologique simplifiée de Carpathes polonaises (d’après (Jankowski, 2004)), nouvelles données de terrain intégrées), indiquant l’emplacement de la coupe régionale X-X’, les sites de l’analyse structurale (points bleus) et des échantillons analysés (points rouges). Les âges AFT de refroidissement pour les Carpathes externes, avec les données provenant d’(Anczkiewicz and Swierczewska, 2008)Anczkiewicz et Swierczewska, 2008, sont indiqués en Ma ± 1σ. L’âge de refroidissement du Crétacé (75,7 ± 12,8) provient de l’échantillon PL7, où un recuit AFT est absent ; pour tous les autres échantillons, qui démontrent un recuit AFT total, les données représentent les âges d’exhumation (refroidissement par l’isotherme ∼110 °C). (c) Coupe géologique établie par l’intégration des données de la géologie de surface avec les logs de forage disponibles et les données sur la structure du socle (Oszczypko, 1998). Masquer
(a) Schéma structural simplifié démontrant la subdivision en Carpathes internes et externes, séparées par la Zone des Klippes de Pieniny, et emplacement de la zone d’étude. (b) Carte géologique simplifiée de Carpathes polonaises (d’après (Jankowski, 2004)), nouvelles données de ... Lire la suite
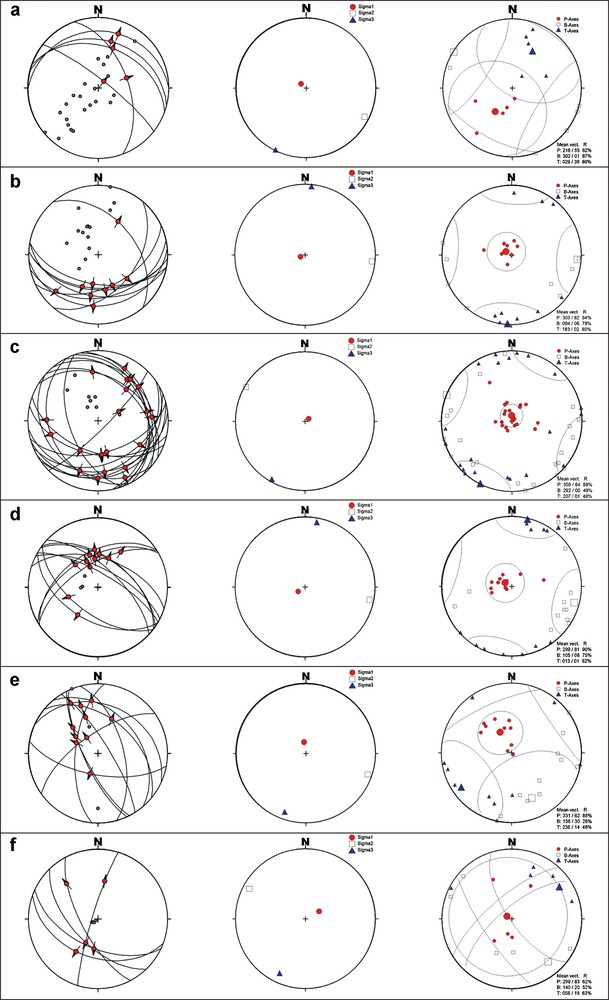
Orientation data and paleostress analysis for post-thrusting structures. For each measurement site (located in Fig. 1a), poles to bedding (S0) and great circles of fault planes (F), showing striae/shear fibre lineation on shear surfaces, are shown in the first column. The second column includes the results of paleostress analysis for the faults in the first column, carried out using the right dihedral method (Angelier and Mechler, 1977). The third column displays the orientation of the principal stress axes (or P-B-T axes (Turner, 1953)) obtained using a value of Θ (angle between the shear plane and the P-axis, i.e. σ1) defined by the maximum clustering of P and T axes (Wallbrecher, 1986). Plots and paleostress analysis were performed using TectonicsFP (software by F. Reiter and P. Acs for Microsoft Windows). (a) Major north-east dipping extensional detachment in the Bystre area, and mescocpic faults in Krosno Fm. strata north of it (in the area of sample PL1). S0 = 22, F = 5. (b)–(c) Major tectonic contact (extensionally reworked thrust zone) at the base of the Magura Unit, southern boundary of the Mszana Dolna tectonic window; for (b), Konina area, S0 = 15, F = 10; for (c), Koninki area, S0 = 8, F = 21. (d) Mesoscopic faults in Krosno Fm. strata, Lipinki area. S0 = 3, F = 11. (e) Mesoscopic faults in Menilite Fm. strata, Folusz area. S0 = 3, F = 10. (f) Hard-linked extensional fault system (tens to hundreds of meters-sized faults) in Krosno Fm. thick sandstones, Kleczany area. S0 = 4, F = 5. Masquer
Orientation data and paleostress analysis for post-thrusting structures. For each measurement site (located in Fig. 1a), poles to bedding (S0) and great circles of fault planes (F), showing striae/shear fibre lineation on shear surfaces, are shown in the first column. ... Lire la suite
Données d’orientation et résultats de l’analyse des paléocontraintes sur les structures post-chevauchement. La première colonne montre, pour chaque site de mesure (situé en Fig. 1a), les pôles du litage (S0) et les grands cercles des plans de faille (F) avec les linéations de strie/cisaillement des fibres présentes sur les surfaces de cisaillement. Les résultats de l’analyse des paléocontraintes pour les failles, réalisée par la méthode des dièdres droits ((Angelier and Mechler, 1977)Angelier et Mechler, 1977) sont présentés dans la deuxième colonne. La troisième colonne montre l’orientation des axes de contrainte principaux (ou les axes P-B-T (Turner, 1953)), établis à partir de la valeur Θ (angle entre le plan de cisaillement et l’axe P, c’est-à-dire σ1), obtenue par le groupement maximum des axes P et T (Wallbrecher, 1986). Les courbes et l’analyse des paléocontraintes ont été exécutées à l’aide du TectonicsFP (logiciel par F. Reiter et P. Acs pour Microsoft Windows). (a) Décollement majeur plongeant vers le nord-est dans la région de Bystre et failles mésoscopiques dans les couches de Krosno Fm. situées au nord (dans la région de l’échantillon PL1). S0 = 22, F = 5. (b)–(c) Contact tectonique majeur (zone de chevauchement remaniée par extension) à la base de l’unité de Magura, à la limite sud de la fenêtre tectonique de Mszana Dolna ; (b) pour la région de Konina, S0 = 15, F = 10 ; (c) pour la région de Koninki, S0 = 8, F = 21. (d) Failles mésoscopiques dans les couches de la Krosno Fm., région de Lipinki. S0 = 3, F = 11. (e) Failles mésoscopiques dans les couches de la Menilite Fm., région de Folusz S0 = 3, F = 10. (f) Système de failles normales fortement lié (les failles allant d’une dizaine jusqu’à plusieurs centaines de mètres) dans les grès épais de la Krosno Fm., région de la Kleczany. S0 = 4, F = 5. Masquer
Données d’orientation et résultats de l’analyse des paléocontraintes sur les structures post-chevauchement. La première colonne montre, pour chaque site de mesure (situé en Fig. 1a), les pôles du litage (S0) et les grands cercles des plans de faille (F) avec ... Lire la suite
3 Low-T thermochronometry
Based on clay mineralogy, fluid inclusion and AFT data, exhumation of sedimentary units from depths in the range of 6–10 km has been inferred for a large part of the Polish Outer Carpathians (Anczkiewicz and Swierczewska, 2008; Swierczewska, 2005; Swierczewska et al., 1999; Swierczewska et al., 2000; Hurai et al., 2004). Although the recorded burial conditions resulted from both sedimentary and tectonic loads, the latter appear to have been largely dominant in controlling the thermal evolution of the analyzed units. In fact, complete stratigraphic successions are preserved and have been extensively studied (Oszczypko, 2004), their thickness being clearly insufficient to explain recorded burial conditions (Swierczewska, 2005). Furthermore, significant burial is recorded by the youngest strata (Krosno Fm) of the stratigraphic successions exposed in the footwall to the Magura Unit. The load on top of such strata could have been represented uniquely by overlying tectonic units.
The post-depositional history of the sedimentary units is particularly well constrained by AFT data (Tables 1 and 2; Fig. 1b). This methodology is a useful tool to unravel the cooling histories experienced by rocks in their motion toward the surface (see (Donelick et al., 2005) for a review). Samples have been collected from medium- to coarse-grained siliciclastic sandstones. Most of the apatite grains are well rounded, ranging in size between 50 and 100 μm. In few cases, euhedral crystals also occur. Sample preparation and analysis followed the procedures outlined in (Zattin et al., 2002). Unfortunately, the low number of measured track lengths does not allow any inference about the rates of cooling for the new samples reported in this study. Moreover, it is not possible to exclude any re-heating event after maximum burial. However, the relationships between stratigraphic and AFT ages suggest that most samples were affected, during the Miocene, by maximum temperatures higher than total annealing temperature (ca. 125 °C (Reiners and Brandon, 2006)). Within the study area, AFT cooling ages range between 32.1 ± 4.8 and 7.0 ± 0.8 Ma. For the uppermost tectonic unit (Magura Unit), cooling ages are in the range of 31.9 ± 4.1 to 15.9 ± 1.9 Ma. In the footwall to the latter unit, cooling ages tend to become younger to the east, reaching values in the range of 10.0 ± 1.1 to 7.0 ± 0.8 Ma. Only one of the analyzed samples (PL7) did not reach burial conditions sufficient to produce total annealing of apatite. This sample records Late Cretaceous exhumation of the source rock.
Résumé des âges stratigraphiques et des âges de refroidissement AFT.
Sample number | Tectonic unit | Stratigraphic age of strata | AFT age |
PL1 | Silesian Unit | Late Oligocene | Late Miocene |
PL4 | Dukla Unit | Late Oligocene | Late Miocene |
PL5 | Magura Nappe | Eocene | Early Miocene |
PL6 | Silesian Unit | Late Oligocene | Late Miocene |
PL7 | Silesian Unit | Early Oligocene | Late Cretaceous |
PL8 | Dukla Unit | Early Oligocene | Late Miocene |
00/18 | Silesian Unit | Cretaceous | Early Oligocene |
Sk 1/00 | Silesian Unit | Oligocene | Early Miocene |
Ta 00/1 | Dukla Unit | Oligocene | Early Miocene |
Ni 00/1 | Dukla Unit | Oligocene | Early Miocene |
Ma 00/1 | Magura Nappe | Eocene | Early Oligocene |
Ba 00/1 | Magura Nappe | Eocene | Early Miocene |
Rz 00/1 | Magura Nappe | Eocene | Early Miocene |
Cze 00/1 | Magura Nappe | Eocene | Late Eocene |
SN 00/1 | Magura Nappe | Eocene/Oligocene | Late Oligocene |
DO 00/2 | Magura Nappe | Eocene | Early Miocene |
Kl 01/1 | Magura Nappe | Eocene | Early Miocene |
Détails des données AFT.
Sample number | Coordinates | No. of crystals | Spontaneous | Induced | P(χ)2 | Dosimeter | Age (Ma) ±1σ | Mean confined track length (μm)±std. err. | Standard deviation | No. of tracks measured | |||
ρ s | N s | ρi | N i | ρd | N d | ||||||||
PL1 | 34U0592661 5463708 | 20 | 0.84 | 78 | 2.04 | 1891 | 92.6 | 0.92 | 4368 | 7.0 ± 0.8 | 13.99 ± 0.26 | 0.99 | 15 |
PL4 | 34U0589234 5461200 | 14 | 0.52 | 17 | 1.22 | 398 | 86.9 | 0.92 | 4355 | 7.2 ± 1.8 | - | - | - |
PL5 | 34U0506233 5490060 | 20 | 3.21 | 132 | 2.51 | 1034 | 92.9 | 0.91 | 4343 | 21.3 ± 2.0 | 12.37 ± 0.58 | 1.92 | 11 |
PL6 | 34U0502288 5494066 | 20 | 1.26 | 90 | 2.08 | 1491 | 76.4 | 0.91 | 4306 | 10.0 ± 1.1 | 14.08 ± 0.38 | 1.27 | 11 |
PL7 | 34U0512961 5499260 | 16 | 10.60 | 405 | 1.92 | 733 | 0.0 | 0.91 | 4331 | 75.7 ± 12.8 | 12.73 ± 0.36 | 1.47 | 17 |
PL8 | 34U0531069 5486244 | 15 | 0.74 | 40 | 1.73 | 933 | 92.5 | 0.91 | 4318 | 7.2 ± 1.2 | 12.35 ± 1.4 | 1.99 | 2 |
An offset of AFT cooling ages in excess of 10 M.y. is recorded across the tectonic contact between Magura Unit hanging-wall rocks (displaying a cooling age of 21.3 ± 2.0 Ma) and the units exposed immediately in the footwall in the Ropa tectonic window (Fig. 1b).
4 Discussion
Timing of exhumation partially overlaps with thrust activity within the study area. However, our new AFT data from the eastern sector unraveled a recent exhumation stage, taking place in the last 10 Ma. Exhumation in this sector largely post-dated thrusting, as the sampled units display AFT cooling ages in the range of 10–7 Ma (Table 2). These values may imply relatively fast exhumation in Late Miocene to Present times, depending on applied paleogeothermal gradients. Paleogeothermal gradients inferred from methane–water fluid inclusions in quartz-calcite veins from the Magura Unit and from tectonically underlying footwall units (exposed in the Mszana Dolna and Szczawa tectonic windows) are 20 °C km−1 and 17 °C km−1, respectively (Hurai et al., 2004). Assuming a mean paleogeothermal gradient of 18 °C km−1 (Swierczewska, 2005), a surface temperature of 10 °C and an AFT closure temperature of 110 °C, a time-averaged exhumation rate of ca. 0.8 mm y−1 is obtained for samples PL1, PL4 and PL8 (all showing AFT cooling ages around 7 Ma). Even assuming a paleogeothermal gradient of 25 °C km−1 (i.e., present-day mean geothermal gradient in the Magura Unit; (Swierczewska, 2005) and references therein), a time-averaged exhumation rate of ca. 0.6 mm y−1 is obtained for the same samples. These values are significantly higher with respect to reconstructed erosion rates. Although these are available only for the Quaternary, a meaningful comparison with time-averaged exhumation rates may be carried out, taking into account the overall lithological homogeneity of the tectonic units forming the thrust belt (i.e., the rocks exposed during the Quaternary are likely to have similar characteristics, in terms of resistance to erosion, with respect to those exposed in Late Miocene and Pliocene times). Quaternary stages of ‘increased erosional activity’, as defined by Zuchiewicz (Zuchiewicz, 1991) based on the analysis of river terraces, are characterized by rates of 0.15–0.21 mm y−1 (800–472 ka) and 0.18–0.40 mm y−1 (130–90 ka). Only for a negligible time span – at the scale of the exhumation processes considered here – of 15 ka (Latest Pleistocene-Holocene), erosion rates in the range of 0.2–2.0 mm y−1 have been inferred by the latter author. Furthermore, the evidence that the youngest beds of the highest stratigraphic unit (Krosno Fm) are generally preserved not only within the tectonic windows – where they occur in the footwall to the Magura Unit – but also ahead (i.e., north) of the Magura Unit front, suggests that the overall amount of erosion has not been large.
The fact that erosion alone cannot explain exhumation rates recorded by sampled rock units cropping out in the eastern part of the study area, together with the evidence of normal faulting post-dating and dissecting fold and thrust structures, all suggest a significant role of extension-related tectonic exhumation. A fundamental role of tectonic exhumation is confirmed by the offset of AFT cooling ages across the tectonic contact separating the Magura Unit from the footwall units in the Ropa tectonic window (area of Ropa 1 well in Fig. 1b). Such a large offset, in excess of 10 M.y., suggests that the tectonic contact at the base of the Magura Unit has been active in this area – probably as a low-angle extensional fault partly reactivating a preexisting thrust – during the last 10 Ma (AFT cooling age of footwall rocks; sample PL6), long after exhumation of the Magura Unit rocks presently exposed in the adjacent hanging-wall block immediately to the south (sample PL5). As the extensional detachment reworked the pre-existing Magura Unit thrust, it is not possible to quantify exactly the magnitude of the extensional displacement, which anyway must have been of the order of a few kilometers (Fig. 1b).
According to (Zuchiewicz, 1998), the spatial arrangement of zones showing Quaternary uplift/subsidence suggests that the main mechanism controlling recent tectonic deformation in the Western Outer Carpathians is the relaxation of remnant horizontal stresses built up during Neogene thrusting. This is consistent with recent, post-thrusting thinning of the Outer Carpathian accretionary wedge as a result of gravity disequilibria. This process was accompanied by both orogen-parallel and orogen-normal extension (Zuchiewicz, 2001). The latter, being dominant, represents the youngest deformation event and also involves low- to moderate-angle normal faults (Rubinkiewicz, 2007). Age and style of extension, being mainly accommodated by roughly east-west striking structures within the study area (Figs. 1 and 2), are compatible with the regional geodynamic framework involving extensional collapse of Alpine belt segments and development of the Pannonian Basin (Horváth et al., 2006). Readjustments within the orogenic wedge also led to the reactivation of preexisting thrusts as low-angle, south dipping extensional faults in the study area. Furthermore, gravitational readjustments appear to have generated rootless, top-to-the-north low-angle faults by denudation of the tilted roof sequence (Magura Unit). The latter process, besides producing disrupted, rotated and structurally ‘chaotic’ frontal bodies of Magura Unit rocks (Konon, 2001; Jankowski, 2007), also enhanced tectonic exhumation of footwall units.
Morphotectonic evidence indicates that neotectonically uplifted areas become more numerous moving from west to east within the Polish Outer Carpathians, thus suggesting an eastward-increasing recent tectonic activity (Zuchiewicz, 1998). This is consistent with young tectonic activity controlling recent cooling ages unraveled by AFT data from the eastern sector of the study area.
5 Conclusions
New AFT data confirm that a significant part of the sedimentary rocks exposed in the Outer Western Carpathians experienced substantial tectonic burial (generally in excess of 6 km). Although part of the exhumation was coeval with shortening, our new data also point out a previously undetected, ‘young’ (i.e., post-thrusting) exhumation stage in the eastern sector of the study area. This, in turn, appears to be associated with a new tectonic regime affecting the orogenic wedge once convergence had ceased. Based on our results, we suggest that recent (< 10 Ma) tectonic evolution and exhumation in the Polish Outer Carpathians were mainly controlled by extension and gravitational readjustments within the orogenic wedge.
Acknowledgments
The article greatly benefited from thorough and constructive reviews by two anonymous Reviewers and CRG Editor. This work was partially supported by the Polish Ministry of Science and Higher Education (Grant NN307244733).