Foreword
The fundamental concepts, their evolution and consequences
In the middle of the 1980s, the so-called Hot Dry Rock (HDR) projects were under consideration, aiming at exploiting the heat stored in deep rocks by circulating heat-bearing fluids in closed loops through fracture networks artificially created in the rock by hydraulic fracturation. The typical geological targets of such projects were hot, and therefore necessarily deep crystalline massifs, which were supposed to be sufficiently tight in order to allow fluid transfers without too much loss of heat bearing fluids between the injection well and the production well, through one or several fractures, artificially maintained open under a sufficiently high pressure in order to allow the expected flow.
These projects had to face numerous technological questions, and they also promoted an important investment of scientific teams1, who realized that:
- • on the one hand, there were very few crystalline massifs, which could be considered as tight enough in relation with their low fracturation, for presenting reservoir volumes sufficiently large to allow their geothermal exploitation within the scope of the first generation of HDR concepts;
- • on the other hand, a high number of complex parameters was governing the circulation, in natural systems or in artificially maintained systems, of the fluids present in deep geological systems, which are rich in fractures, more or less open, but which present a very low matrix permeability.
For that reason, a working group, already initiated at this period, and supported by ADEME, CNRS and BRGM2, involving experts from very different scientific and technological origins, began to coordinate French research and expertise within the framework of a research program called géothermie profonde généralisée (Generalized Deep Geothermal).
Among other works, this group focused on the exploitable geothermal potential that could be offered by deep crystalline rocks, if naturally fractured enough and located in tectonic conditions, favourable enough to shelter deep natural convective loops. These circulation paths could be used for the exploitation of the heat bearing fluids without being forced to create artificially pressurized fractures.
In this context, the group selected the Soultz-sous-Forêts site, where a strong thermal anomaly, well known since oil field research from the 1920s in that area, had been previously intensively studied (Gérard et al., 1984; Munck et al., 1979) and was considered as showing favourable conditions.
The tectonic conditions generating these structures were considered as valid all along the major part of the Upper Rhine Graben area, and a connection with the German group of research teams, who had a similar approach to the problem (Gérard and Kappelmeyer, 1987), allowed us to found what became the Soultz-sous-Forêts European Geothermal Project whose history (Gérard et al., 2006b) is recalled by Genter et al. (2010).
Soon, the Soultz-sous-Forêts site became sufficiently validated (Kappelmeyer et al., 1991) to allow the growth of a program for development and testing of a heat exchanger at depths between 3000 and 3500 m between two wells, 450 m distant, and functioning as a doublet (Gérard et al., 2002) : (Fig. 1)
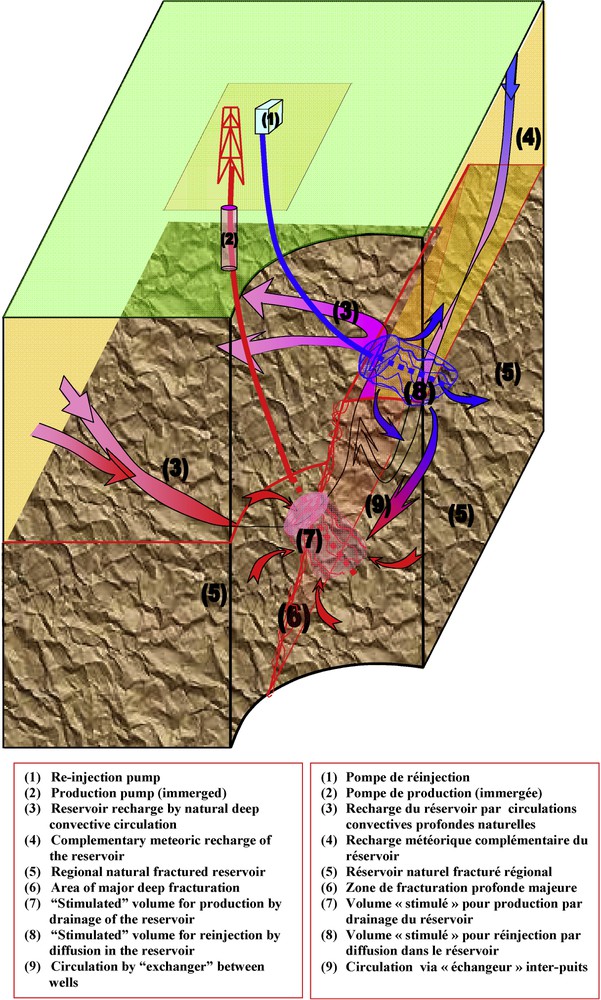
Theoretical scheme of a geothermal doublet functioning as in the context of a “Soultz–type” project.
Schéma de principe d’un doublet géothermique fonctionnant dans un contexte de « type Soultz ».
Following the results of these tests performed in 1997, the initial concept of the system had to be completed in order to take into account the fact that the well-to-well tracing tests showed clearly a very important contribution (> 50%, even more on the long term) of the thermal fluids coming from what had to be considered as a natural reservoir surrounding the wells, and providing, by downhole pumping, enough fluid to govern an equilibrated rate of flow3 between the injected and produced fluids during the tests. As mentioned by Genter et al. (2010), these observations have been confirmed during later experiments using a triplet of wells4 at greater depth (between 4500 and 5000 m).
In fact, the fractured massif surrounding the wells at Soultz, as described in details by Dezayes et al. (2010) and Sausse et al. (2010), is very rich in fractures, more or less open (the rocks looking therefore locally more or less permeable), and hydraulically interconnected, to create a real regional geothermal reservoir from the top of the granitic massif (# –1400 m) to the deepest drilled depths (# –5000 m). As shown by Sanjuan et al. (2010), the reservoir locally studied at Soultz contains a sodium - calcium - chloride brine (≥ 100 g/l) which is obviously provided by a natural convective flux coming from another region of the reservoir, located more in the centre of the Rhine graben, at a higher temperature. This study is completed by Cathelineau and Boiron (2010), who considered the mixing between this brine and some “recharges” of infiltrated meteoric waters. This chemical mixing generate mineral precipitations which may close many microfissures in the system, this phenomenon contributing (at least for a part) to the observed large local variations of the hydraulic behaviour of the reservoir.
In fact, as shown by Géraud et al. (2010), one has to consider that the natural hydrothermal circulation, and that which will occur during the test period and later during the exploitation, will not only concern the “heart” of the fractures, more or less filled by hydrothermal deposits but also, in many cases, the nearby environment of the fractures, which becomes porous due to the alteration of the granitic matrix in a complex general process (Fritz, 1981). In such a context, the choices for the well trajectories, their impact at the level of the reservoir, and the quality of the connections that will be possible to develop from the wall of the well to its vicinity through the most permeable fractures, and the most likely connected to the reservoir, become essential.
It clearly appears that this type of project is now far from the initial “HDR” concept, even in its option applied to naturally fractured environments, and one has to consider new strategies, specifically adapted to the exploitation of natural reservoirs, which are networks of interconnected fractures and fissures, showing a very low, if not negligible, global matrix permeability. The large number of “strong local heat flux anomalies” known in the world, in similar geological situations, shows that natural geothermal reservoirs of this kind are rather common, but their general economic potential has still to be validated by experience with appropriate methods.
Consequently, similar type of projects have appeared in this period in different places in the world, generally designated as Enhanced Geothermal Systems (EGS), but in this volume the authors use both HDR or EGS terms.
Direct consequences of the revised concepts applied to the construction of “EGS” production units
In order to develop an “EGS” production unit, the optimisation of the hydraulic performance of the injection–production well system needs to follow three major guidelines, directly dependent on the revised concept.
The first guideline will govern the strategy for the first well drilling.
This first drilling has to follow a trajectory and to reach impacts in the reservoir aiming to cross as efficiently as possible the network of permeable fractures, which has to be previously identified, as well as possible, after preliminary geological and geophysical studies. Examples of this approach are given by the first part, in papers by Place et al. (2010) and Geiermann and Schill (2010).
The second guideline will govern the organisation of the tasks necessary to be implemented as a priority in order to improve the efficiency of the initial hydraulically active connections (generally rare and of poor quality) between the walls of the wells and the surrounding rocks, along the open hole section.
Their major aim will be to increase the productivity/injectivity indexes of the system5 by reducing as much as possible the fluid friction losses. These fluid friction losses are dreadful at short distances from the wells, due to the high fluid velocities required to collect (or diffuse) the fluids within the reservoir with sufficiently high flow rates using a very small number of cross connections between few hydraulically active fractures and the walls of the wells. Two mechanisms can be operated for this purpose. Their more or less efficient combination will be a key-point for the short-term performance of the exploitation of any “EGS” project:
- • The first mechanism consists in generating fracture shearing from which one hopes to increase the mean residual fracture opening, by injecting a more or less saline solution at high flow rates (i.e., pressures high enough for generating the expected shearings), higher than the future rates for the geothermal exploitation. One speaks here of a “hydraulic stimulation”.
However, using hydraulic stimulation may induce, in the vicinity and inside the reservoir, microseismic events which may be felt (and not appreciated) by the local population; this could even threaten the project. These events could be also suspected to generate some risk for the integrity of the well casings, requiring their poststimulation testing with appropriate methods. Jung et al. (2010) have successfully experimented in Soultz one of those methods.
The optimisation of the safety and efficiency of this method and its possible limitation at the close vicinity of the wells is still under discussion for its specific application in EGS local conditions (Gentier et al., 2005; Gérard et al., 2006a, 2006b).
The impact(s) and efficiency of the hydraulic stimulation will depend on the combination between the local stress field, the geometry and “permeability distribution” of the fracture and fissure network surrounding the wells. These local conditions could sometimes be critical and will have to be carefully considered and taken into account for the design of any stimulation program aiming at creating the required over-pressure for any significant shearing activity, even limited inside the close vicinity of the well. Dorbath et al. (2010) propose a method for deducing the local stress field during a hydraulic stimulation test from the signals detected in the generated microseismic activity, in a way which has still to be explored more completely in order to improve the driving operations for their better efficiency and safety.
- • The second mechanism consists in a chemical treatment of the hydrothermal deposits which either obstruct the walls of the fractures which are hydraulically active and which are selected as in need of improvement, or which, after they have been crushed by the fracture shearing due to the hydraulic stimulation, are therefore displaced by the injected flow which partially clogs the neighbouring connections having diameters6 smaller than this debris.
The definition of the most adapted chemical treatments needs a complete knowledge of the composition of deposits in the fractures. Ledésert et al. (2010) give an example of the type of studies which may be done and the results which can be expected.
The third guideline will govern studies aiming at optimising the strategy for the selection of the trajectory and the impact on the reservoir of the second well7 from the results of the first drilling and the database available at that moment. These points are strategic for the future exploitation of the system and the efficiency of the choices applied will have to be estimated from the first tests of the global performance of the wells and reservoir system.
Indeed, the presence of natural fractures hydraulically active in the volume separating the drilling impacts in the reservoir may considerably favour the circulations from the re-injection well(s) to the production well(s) and, by the way, could increase significantly the global hydraulic performance of the system.
However, these fluid transfers could be too direct (too short distances, or large opened fractures making direct connections between wells, or a fracture morphology implying exaggerated channelling). Such a situation may considerably harm the long-term stability of production temperatures. Neuville et al. (2010) document this question of the impact of the fracture morphology on the thermal exchanges.
On the other hand, a larger distance between wells or an unsuitable distribution of wells impacts addressing the reservoir zone may reduce the risk of “thermal short connection”, but will increase the risk of low global hydraulic performance of the system by creating a handicap to the recharge of the reservoir around the production wells. The second part of the study by Place et al. (2010) illustrates the identification of the major fractures by VSP (Vertical Seismic Profiler), a very promising technique on the way to optimising the second or third wells trajectory and impact at the reservoir.
On the way to exploit “EGS” units
The expected duration of EGS systems, as shown in details by Heidinger (2010) and by a more general study from 1999 (Delacroix, 1999), should be around 20 years in order to pay off the investments which may be quite important for such projects8.
After a precise initial diagnostic of the possible performance and durability of the system, once under operation, it will be necessary to assess periodically the performance and behaviour, using thermohydraulic modelling, taking into account updated data from the exploited system. This point is particularly important in order to be able to prepare, in useful time, adapted decisions for the industrial management of the system. In this way, chemical tracings between wells should be an efficient tool for creating a database for all future modelling of the exploited geothermal system. Auradou et al. (2010) show how tracers can be used in such complex geological systems to follow their progression and the mechanism of their dispersion in the reservoir. These points have to be well understood to make progress in the industrial use of the tracers’ information.
Among major mechanisms that will govern the future evolution of a geothermal reservoir along the pathways of the reinjected fluid during tens of years of exploitation, it is clear that the water–rock interaction processes will play an important and complex role in the “life” of the reservoir, generating local dissolution processes, or on the contrary scaling phenomena. The location, the nature and the intensity of these processes will be variable in space and time. A major effort of research has been devoted in the Soultz-sous-Forêts project to these processes and some of the major results presented in this issue by Fritz et al. (2010), Portier and Vuataz (2010), Hellmann et al. (2010). All aspects of these water–rock interaction processes in the geothermal context are not yet totally understood and are still under development, in particular, for everything that concerns the kinetics of dissolution (Schott et al., 2009) and even more for the precipitation processes (Fritz and Noguera, 2009).
Conclusions
The results presented in this volume give a first overview of the scientific results which have been obtained in about 15 years in the framework of an industrial and scientific project, associating and stimulating the fundamental research all along its evolution from preliminary field work to the “final” production tests at the interface between science and industrial projects in Earth Sciences. This is not so common and has to be mentioned as having been very positive for both the scientific development and the industrial management of the project. The scientific results can now be applied to the conception and development of other EGS operations from the same type. The EGS concept succeeded progressively to the initial HDR concept, as it appeared clear that even most of the supposed “dry rocks” are usually more or less heavily naturally fractured and that the future geothermal exploitation of deep geological fractured systems requires a locally favourable natural fracture network and specific techniques. Now, this EGS concept addresses regions where rocks are fractured enough and under present tectonic conditions favourable enough for sheltering potential geothermal reservoirs. Some very promising ways have been investigated for their future exploitation, providing that the stimulation (and their exploitation parameters) will not create disturbing seismic hazards.
The development of other future operations will obviously promote complementary specific studies which may involve the expertise of the Soultz teams. The coming years will probably bring new opportunities in relation with the general energy problem accessibility in the world.
Avant-propos
Les concepts fondamentaux, leurs évolutions et leurs conséquences
Au milieu des années 1980, divers observateurs commençaient à s’interroger sur la pertinence des espoirs initialement soulevés par divers projets, en cours ou en préparation, dits hot dry rock (HDR) qui visaient à exploiter la chaleur stockée dans les roches profondes par la circulation, en boucle fermée, d’un fluide caloporteur au sein de fractures artificiellement crées par fracturation hydraulique. L’objectif type pour de tels projets était un massif de roches cristallines chaudes (et donc profond) supposé assez étanche pour permettre le transit sans pertes excessives d’un fluide caloporteur entre un puits d’injection et un puits de production, via une ou plusieurs fracture(s) artificielle(s) maintenue(s) sous pression suffisante pour assurer le débit voulu.
Au-delà des nombreuses questions qu’ils soulevaient, ces projets avaient cependant contribué dés cette époque au développement de la prise de conscience au sein d’équipes scientifiques diverses9 :
- • d’une part, de l’extrême rareté des massifs cristallins, assez peu « fissurés » naturellement pour pouvoir être considérés comme étanches sur des volumes suffisants pour permettre leur exploitation géothermique via les concepts HDR de première génération ;
- • d’autre part, de la complexité et de la multiplicité des paramètres gouvernant les circulations, naturelles ou artificiellement entretenues, des eaux présentes dans des milieux riches en fractures plus ou moins ouvertes mais dépourvus de toute perméabilité matricielle significative.
C’est pourquoi, dès cette époque, un groupe de travail, animé par l’Ademe, le CNRS et le BRGM10 avec la participation de divers experts d’autres origines, a entrepris de coordonner les réflexions et expériences françaises (en cours ou en projet) dans le cadre d’un programme dit « Géothermie Profonde Généralisée ». Entre autres travaux, ce groupe s’est intéressé au potentiel géothermique exploitable que pourraient recéler des milieux cristallins profonds assez fracturés naturellement et actuellement situés dans des conditions tectoniques suffisamment favorables pour laisser place à des circulations naturelles convectives profondes, suivant des chemins qui pourraient (après d’éventuels travaux d’aménagement par des moyens appropriés) servir de vecteurs à des échanges inter-puits d’un fluide caloporteur, sans devoir pour autant faire appel à des fractures artificielles pressurisées.
C’est dans ce contexte, que ce groupe a retenu l’intérêt du site de Soultz-sous-Forêts où une forte anomalie thermique (bien connue depuis les années 1920 par des travaux pétroliers) avait fait l’objet de nombreuses études depuis (Gérard et al., 1984 ; Munck et al., 1979) démontrant à cet endroit l’existence des conditions requises.
Les conditions tectoniques à l’origine de structures de ce type pouvant être considérées comme valides tout au long de la majeure partie du Fossé Rhénan supérieur, un rapprochement avec un groupe d’équipes allemandes, qui de leur côté avaient développé des réflexions du même ordre (Gérard and Kappelmeyer, 1987), a permis de poser les bases de ce qui est devenu le Programme de Recherches Géothermique Européen de Soultz-Sous-Forêts dont l’historique (Gérard et al., 2006b) est repris et complété par Genter et al. (2010).
Très rapidement le site de Soultz-sous-Forêts a pu être suffisamment validé (Kappelmeyer et al., 1991) pour permettre le développement du programme vers la création et les essais d’un échangeur à des profondeurs de 3000 à 3500 m, entre deux puits distants de 450 m fonctionnant en doublet (Gérard et al., 2002) (Fig. 1).
Au vu des résultats de ces essais conduits en 1997, on a dû constater que les concepts initiaux devaient être pour le moins complétés afin de prendre en compte les résultats des traçages inter-puits, qui démontraient la très forte contribution (> 50 % même à long terme) des eaux thermales en provenance de ce qui apparaissait comme un réservoir naturel présent autour des puits et assurant un équilibre exact à débit stable entre les volumes totaux produits et réinjectés.
Comme le rappellent Genter et al. (2010), ces observations ont été confirmées lors des expériences conduites ultérieurement, cette fois à partir d’un triplet11 entre 4500 et 5000 m de profondeur.
De fait, le milieu fracturé environnant les puits forés à Soultz, dont les articles de Dezayes et al. (2010) ainsi que de Sausse et al. (2010) fournissent une description détaillée, est assez riche en fractures plus ou moins ouvertes (donc localement plus ou moins perméables) et hydrauliquement interconnectées pour constituer, depuis le sommet du massif granitique (#−1400 m) jusqu’aux profondeurs explorées (#−5000 m), un véritable réservoir géothermique régional.
Comme le montre l’article de Sanjuan et al. (2010), le réservoir localement étudié à Soultz serait vraisemblablement alimenté par une saumure de salinité totale élevée (≥ 100 g/l), animée par un flux naturel convectif et en provenance d’une autre région du réservoir qui serait à température plus élevée et située vers le centre du Fossé Rhénan.
Les apports de ce travail sont complétés dans le présent volume par l’article de Cathelineau et Boiron (2010) qui prennent en compte les mélanges entre cette saumure et des « recharges » pluviales et leur impact sur les dépôts qui vont progressivement configurer les chenaux vecteurs des circulations, voire colmater nombre de microfissures.
De fait, comme le montre l’article de Géraud et al. (2010), on est conduit à observer que les circulations hydrothermales naturelles (et celles que l’on développera pendant les essais, puis que l’on entretiendra pendant l’exploitation) vont avoir pour cadres, non seulement les « cœurs de fractures » encombrés de dépôts hydrothermaux, mais aussi, dans bien des cas, l’environnement immédiat de leurs épontes rendu poreux par altération de la matrice granitique, l’ensemble ayant évolué selon des mécanismes généraux dont les résultats sont fort complexes (Fritz, 1981).
Dans un tel contexte, les choix relatifs aux trajectoires des puits et à leurs impacts au niveau du réservoir, ainsi que la qualité des connexions qu’il sera possible de développer à partir de la paroi des puits vers les fractures du voisinage les plus perméables et les mieux connectées à l’ensemble du réservoir, deviennent essentiels.
Comme on peut donc le constater, on s’est à présent beaucoup éloigné des concepts initiaux HDR, même dans le cas de leur « variante » appliquée à des milieux naturellement fracturés, pour parvenir à la définition de nouvelles stratégies spécifiquement adaptées à la mise en valeur d’ensembles de fractures/fissures interconnectées en milieux de perméabilité matricielle globalement négligeable mais constituant néanmoins de vastes réservoirs géothermiques naturels régionaux exploitables, sous réserve de les mettre en service avec des moyens appropriés. On s’est, par contre, beaucoup rapproché d’une généralisation de certains concepts déjà industriellement exploités dans quelques cas localement particulièrement favorables, en constatant l’intérêt d’adapter leur application aux conditions que l’on rencontrera sur de nombreux sites beaucoup moins exceptionnels.
Des projets du même type ont maintenant vu le jour en d’autres endroits et ils sont le plus souvent désignés sous le sigle Enhanced Geothermal Systems (EGS) mais dans les articles de ce volume, les auteurs emploieront encore indifféremment les deux dénominations HDR ou EGS.
Conséquences directes des concepts révisés appliqués à la construction d’unités de production EGS
Pour mettre en exploitation, par des unités de production de type EGS, des milieux de caractéristiques adéquates, la recherche de l’optimisation des performances hydrauliques de l’ensemble que constituent les puits d’injection et de production va devoir suivre trois lignes directrices majeures qui viennent en conséquence directe des concepts révisés, décrits précédemment.
La première ligne guidera la stratégie de forage du premier puits.
Elle nécessite de choisir pour le premier puits une trajectoire et des impacts au sein du réservoir visant à recouper puis à reconnaître au mieux le réseau des fractures perméables sur la base d’études préalables dont la première partie de l’article de Place et al. (2010) et celui de Geiermann and Schill (2010) fournissent des exemples dans ce volume.
La seconde ligne guidera les travaux nécessaires pour chercher prioritairement à développer, les performances des connexions initiales (le plus souvent peu nombreuses et de piètre qualité) entre les parois des puits et le réservoir qui les entoure sur l’intervalle des profondeurs choisi.
L’objectif majeur sera ici d’accroître au mieux l’index de productivité/injectivité de l’ouvrage12 en réduisant autant que possible les pertes de charge qui sont redoutables à faible distance des ouvrages, en raison des vitesses des fluides extrêmement élevées, requises pour qu’ils soient collectés (ou diffusés) au sein du réservoir avec des débits importants, à partir d’un très petit nombre d’intersections entre quelques fractures hydrauliquement actives et les parois des puits.
Deux mécanismes vont pouvoir être mis en œuvre à cette fin. De leur combinaison plus ou moins judicieuse dépendront, dans la plupart des cas, les performances exploitables à court terme d’un projet EGS.
- • Le premier mécanisme consiste à générer des coulissements des fractures dont on espère améliorer ainsi l’ouverture résiduelle moyenne, par injection d’eau plus ou moins salée à des débits (et donc sous des pressions) élevés vis-à-vis des futurs débits d’exploitation envisagés. On parle en ce cas de « stimulation hydraulique ». Néanmoins, la stimulation hydraulique peut induire, au voisinage et au sein du réservoir, des évènements microsismiques qui peuvent être très mal ressentis par la population locale et pourrait même menacer le projet. Ces événements pourraient également constituer un risque pour l’intégrité des tubages de puits, nécessitant d’effectuer un test post-stimulation de ces derniers, par des méthodes appropriées. Jung et al. (2010) ont expérimenté avec succès une de ces méthodes à Soultz.
Son emploi, générateur d’activités microsismiques, demande, d’une part, la plus grande prudence afin de limiter les risques d’éventuelles manifestations, susceptibles d’être perceptibles par les populations environnantes, et d’autre part, des modalités d’application bien spécifiques encore très discutées (Gentier et al., 2005 ; Gérard et al., 2006a, 2006b) visant à en optimiser l’efficacité au voisinage des puits.
Entre autres conditions, c’est de la combinaison entre le champ des contraintes local et le réseau des fractures/fissures environnant les puits que vont dépendre les impacts et l’efficacité d’une « stimulation hydraulique », conduite avec un débit choisi, dans l’espoir d’obtenir au voisinage du puits traité la surpression voulue. L’article de Dorbath et al. (2010), propose une méthode pour déduire le champ de contrainte local à partir des signaux issus de l’activité microsismique, s’inscrivant ainsi dans une voie qui reste encore à explorer plus complètement, vers la sécurisation des opérations de développement d’un champ géothermique.
- - Le second mécanisme consiste à « traiter » chimiquement les dépôts hydrothermaux, soit qui encombrent les parois des fractures hydrauliquement actives dont on souhaite améliorer les caractéristiques, soit qui, réduits à l’état de déblais du fait des coulissements induits par les « stimulations hydrauliques », vont, plus ou moins partiellement, obturer toutes les connexions voisines de sections inférieures à leurs diamètres13.
La définition des traitements chimiques les plus adéquats demande une connaissance approfondie de la nature de ces dépôts et l’article de Ledésert et al. (2010) est un exemple, sur le cas de Soultz, des travaux que l’on peut accomplir à cette fin et des résultats que l’on peut en attendre.
La troisième ligne guidera les choix à faire pour définir la trajectoire et l’impact au réservoir du second puits, à partir des résultats du premier forage14 et sur la base des observations disponibles à ce moment.
Ces choix sont stratégiques pour l’exploitation ultérieure des ouvrages et il importera de vérifier leur efficacité dès les premiers tests des performances globales du système puits–réservoir.
En effet, la présence de fractures naturelles hydrauliquement actives dans les régions séparant les impacts au réservoir des ouvrages peut considérablement favoriser les circulations à partir des puits de réinjection vers les puits de production et de ce fait accroître sensiblement les performances hydrauliques du système.
Cependant, des transferts trop directs (distance ou connexions inter-puits respectivement trop faible(s) ou de morphologie inadéquate) peuvent nuire considérablement à la pérennité des températures de production. Pour ce qui concerne l’impact de la morphologie des fractures sur les échanges thermiques, l’article de Neuville et al. (2010) en fournit un exemple.
Inversement, un éloignement abusif ou une distribution inadéquate des impacts réduira les risques de « court circuit thermique », mais fera peser un risque sur les performances hydrauliques globales des ouvrages en créant un handicap à la recharge du réservoir autour des puits de production.
La seconde partie de l’article de Place et al. (2010) donne dans ce volume un exemple de reconnaissance de fractures majeures par profilage sismique vertical, technique qui s’avère tout à fait prometteuse pour éclairer les choix à faire le moment venu.
Vers l’exploitation d’unités de production EGS
Comme le montre l’article de Heidinger (2010) dans ce volume, qui précise les vues d’une étude plus générale publiée en 1999 (Delacroix, 1999), une durée d’exploitation de l’ordre de 20 ans ou davantage peut être requise le plus souvent pour amortir des investissements qui peuvent être considérables.
Après un diagnostic approfondi, destiné à évaluer la pérennité de leurs performances jugées opérationnelles à l’issue des travaux précédant leur mise en service, les unités de production qui fonctionneront sur la base des concepts précédemment décrits n’en devront donc pas moins faire périodiquement l’objet de modélisations thermohydrauliques destinées à évaluer l’évolution prévisionnelle de leur comportement, pour permettre à l’exploitant de prendre en temps utile les mesures adéquates.
Pour ce faire, les traçages inter-puits sont un outil essentiel dont l’interprétation des résultats constitue l’une des bases indispensables à toute modélisation. Auradou et al. (2010) montrent que, lorsque des traceurs sont utilisés dans des milieux aussi complexes, leurs cheminements et donc les modalités suivies par leur dispersion dans le réservoir étudié exercent des influences complexes qu’il importera de prendre autant que possible en compte.
Parmi les mécanismes de base qui vont gouverner les évolutions internes du réservoir tout au long des chemins qu’emprunteront les fluides géothermaux pendant les décennies envisagées pour l’exploitation, il est certain que les échanges chimiques eau–roche vont jouer un rôle essentiel mais complexe dans la « vie » du réservoir, en générant des phénomènes locaux de dissolution, ou au contraire « d’entartrage ». Leur localisation, leur nature et leur intensité seront variables selon la distribution des températures et les variations de la composition des mélanges des fluides circulant en contact avec divers minéraux dont la plus grande partie sera d’origine hydrothermale.
Bien que des efforts considérables aient été accomplis dans le cadre des travaux des équipes scientifiques contribuant au projet pilote de Soultz-sous-Forêts, et dont les résultats majeurs sont présentés dans ce volume par Fritz et al. (2010), Portier et Vuataz (2010), Hellmann et al. (2010), tous les aspects de ces mécanismes et de leurs conséquences ne sont pas encore totalement maîtrisés en raison de leur complexité (Fritz et Noguera, 2009 ; Schott et al., 2009). Cependant, leur importance est telle, que les recherches en ce sens se poursuivent dans le cadre du projet pilote de Soultz avec un rang de priorité élevé.
Conclusions
L’ensemble des articles présentés dans ce volume constitue un « état des lieux » (à l’exception notable des résultats en cours d’acquisition pour ce qui concerne la modélisation thermo-hydrodynamique des essais réalisés et en cours à Soultz), qui permet une première vue d’ensemble des résultats d’approches scientifiques dont la plupart seront transposables vers la conception et la mise en œuvre des futures opérations de type EGS dont la préparation est en cours et qui contribueront à leur tour aux progrès à venir.
Il est vraisemblable, en effet, qu’au fur et à mesure des développements de ces opérations nouvelles, bien d’autres évolutions vont apparaître ultérieurement comme utiles, voire nécessaires et que les équipes ayant participé à ce volume sont donc bien loin d’avoir achevé leurs tâches.
C’est là une situation courante quand une industrie nouvelle tente de naître et les prochaines années vont selon toute vraisemblance être décisives.
Remerciements
Au nom de toutes les équipes scientifiques impliquées dans le projet de Soultz-sous-Forêts, et qui ont participé à ce volume, les auteurs souhaitent remercier pour leur support financier durant ces années de recherche le GEIE « Exploitation Minière de la Chaleur », consortium de partenaires industriels (EDF, EnBW, ES, Pfalzwerke, Evonik, Bestec), le ministère français de l’Enseignement supérieur et de la recherche, l’ADEME, le CNRS et le BRGM, en France, le Bundesministerium für Umwelt, Naturschutz und Reaktorsicherheit, les porteurs de projets, Jülich, BGR, EIfER, GTC, LIAG, et MeSy, en Allemagne, et IFE en Norvège. Les auteurs souhaitent également remercier l’Office fédéral suisse pour l’éducation et la science (Projet no 03.0460), l’Office fédéral suisse pour l’énergie (Projet no 150’649), ETH Zurich, Geowatt, CREGE, Häring Geoproject, Polydynamics Engineering, l’université de Neuchâtel, et la Communauté européenne pour ces soutiens financiers dans le cadre des 5e et 6e PCRD (contrats Hot Dry Rock Energy ENK5-2000-00301, et EGS Pilot Plant SES-CT-2003-502 706 respectivement).
Acknowledgments
In the name of all the scientific teams involved in the Soultz-sous-Forêts project, who participated to this volume, the authors would like to thank for their financial support during years of research the GEIE “Exploitation Minière de la Chaleur” made with a consortium of industrial members (EDF, EnBW, ES, Pfalzwerke, Evonik, Bestec), the ministère français de l’Enseignement supérieur et de la recherche, the ADEME, the CNRS, and the BRGM, in France, the German Bundesministerium für Umwelt, Naturschutz und Reaktorsicherheit, the Projektträger Jülich, BGR, EIfER, GTC, LIAG, and MeSy, in Germany, and IFE in Norway. The authors would also like to thank the Swiss Federal Office of Education and Science (Project No. 03.0460), the Swiss Federal Office of Energy (Project No. 150,649), ETH Zurich, Geowatt, CREGE, Häring Geoproject, Polydynamics Engineering, université de Neuchâtel, and the European Community with the financial supports of the 5th and 6th Framework Programmes, contracts Hot Dry Rock Energy ENK5-2000-00301, and EGS Pilot Plant SES-CT-2003-502 706 respectively.
1 They were also involved in other research programs or projects already exploited at that time with positive economic results, geothermal reservoirs naturally productive in fractured rocks more or less deep, and studies which were investigating crystalline massifs, considered to be tight enough to offer possibly safe storage sites for various wastes.
2 Agence de l’environnement et de la maîtrise de l’énergie, Centre national de la recherche scientifique, Bureau de recherches géologiques et minières.
3 This equilibrium was “per definition” due to the fact that only the produced flow was re-injected.
4 Two production wells located on each side of a re-injection well placed in central position.
5 The productivity/injectivity index of a well has been defined as the ratio between the flow rate (produced or injected) and the necessary pumping or injection pressure.
6 In some cases, one will have to apply a treatment to the cuttings which could have been unfortunately injected while drilling within the opened fracture network in the viciniy of the well's open hole section.
7 And the possible following ones.
8 This assumption being based on present energy prices.
9 D’autres programmes ou projets en cours à cette époque exploitaient déjà, avec des performances économiquement exploitables, des réservoirs géothermiques naturellement productifs en milieux fracturés plus ou moins profonds et de nombreuses études visaient à rechercher des massifs cristallins suffisamment étanches pour pouvoir abriter d’éventuels sites de stockages de déchets divers.
10 Agence de l’environnement et de la maîtrise de l’énergie, Centre national de la recherche scientifique, Bureau de recherches géologiques et minières.
11 Deux puits de production placés de part et d’autre d’un puits de réinjection en position centrale.
12 On définit l’index de productivité/injectivité d’un puits comme le rapport entre le débit produit (ou injecté) et la pression de soutirage (ou d’injection) nécessaire.
13 Dans certains cas, on devra aussi essayer de traiter des déblais malencontreusement injectés dans le réservoir en cours de foration.
14 Et des suivants le cas échéant.