1 Introduction
Over past decade, the interest of scientific community to the Arctic and the permafrost terrains in general increased tremendously, producing the amount of articles equals to that published on these topics between 1950 and 2002 (Web of Science search). This is certainly due to high importance of the Arctic Ocean and permafrost-dominated subarctic continental zones in water and carbon cycle on the Earth and the high vulnerability of circumpolar zones to the climate warming. However, the majority of conducted works have been devoted to the biogeochemistry of organic carbon whereas the main factors controlling biogeochemical cycles of other major and trace elements in the watersheds of the Arctic ocean remained poorly understood. The main specificities of the boreal Eurasian high latitude watersheds extended over cold and humid zone on the organic-rich soils making them drastically different from “classically” studied temperate and tropical regions are:
- • extremely high seasonal variability of element concentration in rivers producing the majority of annual organic carbon (OC) flux and related elements during 2 to 3 weeks of the springflood;
- • essentially unweathered nature of the rock substrate often located within 10 to 40 cm from the surface;
- • the effect of cryoturbation and freezing front migration yielding highly homogeneous soil profile;
- • specific poorly humified, fulvic-like dissolved organic matter of low molecular weight (LMW) in summer and high molecular weight (HMW) in spring, and at the same time the presence of old (Pleisotocene age) dissolved OC;
- • high concentration of dissolved Fe producing organo-ferric colloids as the main vectors of TE transport in surficial fluids;
- • high abundance, up to 70 to 80% of the watershed area, of glacial or thermokarst lakes capable controlling the solute transport from the soil to the river;
- • abundant coniferous forest of essentially deciduous Larix spp. throughout northern Siberia.
The importance of boreal and subarctic continental zones in regulation of both organic and inorganic carbon cycle follows from:
- • the high storage of Corg in soils;
- • the dominance of humid climate supporting abundant vegetation and yielding high riverine fluxes of both carbon and nutrients from the land to the Arctic Ocean;
- • the presence of shallow soils and fresh rocks exposed to the surface and capable uptaking atmospheric CO2 during weathering;
- • increase of physical degradation of rocks due to frost action and freezing cycles.
However, these “positive” factors, potentially enhancing the magnitude of C fluxes in subarctic zone compared to other regions may be overweighed by low-temperatures, short active period, low chemical weathering and denudation rates. The prediction of the evolution of these major driving forces under on-going climate warming requires understanding of physico-chemical and biological mechanisms that can be achieved solely by field observations, on-site measurements and modeling experiments.
Unlike many regions of the world, the Arctic and Subarctic zone exhibit extreme variations in the discharge and chemical elements concentration (Bagard et al., 2011; Gaillardet et al., 2003; Gislason et al., 1996; Gordeev et al., 1996; Guay et al., 2010; Guo et al., 2012; Huser et al., 2011; Prokushkin et al., 2011; Rember and Trefry, 2004; Stedmon et al., 2011; Stefansson and Gislason, 2001; Zakharova et al., 2005). The quantitative description of these systems, therefore, requires an understanding of how weathering rates vary seasonally. However, this high seasonality implies significant variations in the source of the elements in river flow over the year, which is further accentuated by high variability of the depth of the active layer and relevant contribution of mineral soil weathering processes compared to the leaching of the organic horizon of soils. As such, the important goal of this study was to assess the relative role of mineral dissolution versus plant litter dissolution in the rate of chemical denudation and element fluxes from the land to the ocean. Although several recent studies used isotopic techniques in an attempt to resolve the sources of elements in Eurasian rivers (Engström et al., 2010; Prokushkin et al., 2007; Reynolds et al., 2006), the contribution of mineral versus plant litter remains poorly constrained particularly for boreal watersheds. Precise knowledge of these sources is essential for predicting the response of Arctic ecosystems to the global warming as the plant litter production and degradation respond more rapidly to environmental change than abiotic mineral dissolution.
As a result of difficulties in conducting high-resolution geochemical studies in space and time in these remote and hostile regions, the majority of available geochemical information has been collected during the easiest time of sampling, the summer low flow period (Huh and Edmond, 1999; Huh et al., 1998). Recently, the fluxes of suspended and conventionally dissolved (< 0.45 μm) major components of the Arctic rivers became available thanks to thorough Partners program (Holmes et al., 2012 and references therein). However, a number of important issues of biogeochemical cycles in the Arctic remain poorly resolved. First, the relative contribution of colloidal fraction for other than DOC components (i.e., Guo and Macdonald, 2006) remains virtually unknown except for the watersheds of the Baltic Sea (cf., Dahlqvist et al., 2004; Ingri et al., 2000). Second, the origin of elements in rivers and the role of vegetation in element cycling remain poorly characterized, and, third, the role of highly abundant lakes as both terminal receptors of elements from the watershed (in glacial landscape) and the mediators of element transfer from the soil to the river (in thermokarst setting) is not yet well-quantified. To better characterize the above-mentioned issues, this work synthesizes results of multidisciplinary research conducted by our group over past decade in various climate and lithological zones of boreal and subarctic Eurasia and provides a first-order assessment of future changes in element biogeochemical cycles under climate warming scenario.
2 Study sites, sampling and analyses
The study sites are shown on the map of permafrost distribution in Russian Federation (Fig. 1). We investigated monolithological (granites, gneisses, carbonates, basalts) and mixed lithological (sedimentary rocks, carbonates, sandstones, claystones) river basins of both permafrost-free and continuous permafrost zones (European part of NW Russia, western and central Siberia, respectively). For comparison, discontinuous permafrost sites of the Aldan shield in the Transbaikal region (granite-gneisses) and Kamchatka peninsula (basalts, tuffs and sedimentary rocks) were also considered. Boreal and subarctic permafrost-free zone includes the Karelia region located in North-West Russia on the eastern Fennoscandian Shield and Severnaya Dvina basin of the Arkhangelsk region on sedimentary rocks (carbonates, clays). The western Siberia peat deposits developed on Neocene sands and clays offer a look on discontinuous permafrost zone subjected to active thermokarst processes. Finally, in continuous permafrost zone, we selected basaltic province located within the central Siberian Plateau which includes a considerable part of the basins of the Nizhnyaya Tunguska and Podkamennaya Tunguska Rivers, tributaries of the Yenisey River. All sites can be considered as pristine with minimal impact of human activity except long-range atmospheric deposition.
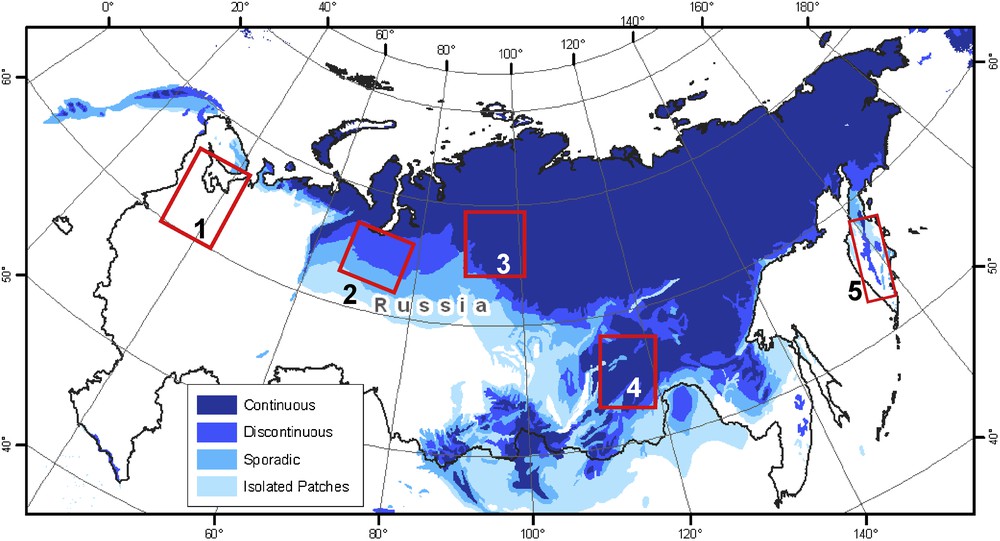
Permafrost distribution in Russian Federation and our study sites in NW Russia, western and central Siberia, Transbaikal region and Kamchatka. The map is based on Brown et al. (1998). Rectangles denote the regions selected for this work: 1, European part of Russia with granites, basalts and carbonates covered by 0.5 to 40 m glacial deposits (Pokrovsky and Schott, 2002; Pokrovsky et al., 2005a, 2010a, 2012; Vasyukova et al., 2010; Zakharova et al., 2007); 2, Western Siberia with peat deposits (0.5 to 3 m) over Neogene sands and clays (Audry et al., 2011; Pokrovsky et al., 2011); 3, basalts and tuffs of central Siberian Plateau (Pokrovsky et al., 2005b, 2006; Prokushkin et al., 2011); 4, Transbaikal Aldan shield of granite-gneisses (Zakharova et al., 2005); 5, Kamchatka peninsula with basalts and sedimentary rocks (Dessert et al., 2009).
Distribution du permafrost dans la Fédération de Russie et sites d’étude dans le Nord-Ouest de la Russie, la Sibérie occidentale et centrale, la région de Transbaïkal et le Kamtchatka. La carte est basée sur des données de Brown et al. (1998). Les rectangles correspondent aux régions sélectionnées pour ce travail. 1. Partie européenne de la Russie, avec granites, basaltes et carbonates recouverts de 0, 5 à 40 m de dépôts glaciaires (Pokrovsky and Schott, 2002; Pokrovsky et al., 2005a, 2010a, 2012; Vasyukova et al., 2010; Zakharova et al., 2007) ; 2. Sibérie occidentale, avec dépôts de tourbe (0, 5 à 3 m) sur des sables et argiles néogènes (Audry et al., 2011; Pokrovsky et al., 2011) ; 3. Basaltes et tufs du Plateau central sibérien (Pokrovsky et al., 2005b, 2006; Prokushkin et al., 2011) ; 4. Bouclier Aldan trans-Baïkal de granite et gneiss (Zakharova et al., 2005) ; 5. Péninsule du Kamtchatka, avec basaltes et roches sédimentaires (Dessert et al., 2009).
Weathering rates and fluxes of major and trace elements reported in this study are based on two data sources. The first source is samples collected and analyzed by the Russian Hydrological Survey in 1960 to 1970th (Resources of Surface Waters of the U.S.S.R, 1972, 1973). The second data source is water samples that we have collected during different seasons over several hydrologic years. These data sets allow:
- • the rigorous estimation of mean annual dissolved element fluxes for watersheds of various sizes and establishing the relative mobility of elements during weathering;
- • the resolution of how various environmental factors affect weathering rates;
- • assessing how these factors depend on a season (i.e. snowmelt, summer-fall and winter baseflow).
In addition to the river basins, thermokarst lakes formed due to permafrost thawing and soil subsidence were investigated in vast territory of the western Siberia along a latitudinal transect of 1000 km, from discontinuous/sporadic permafrost of the Nojabrsk region to the continuous permafrost of Novyi Urengoy region and Gyda Peninsula. To our knowledge, the chemical composition of thermokarst lakes in western Siberia has not been investigated previously.
River and lake water samples were taken from the shore or from the boat and processed on-site, within 2 to 6 hours after collection as described elsewhere (Pokrovsky et al., 2010b; Shirokova et al., 2010). Suspended load was quantified via decantation of large volumes (20 to 50 L) followed by filtration through 0.22 μm (Shevchenko et al., 2010) where as colloidal part (1 kDa–0.22 μm) was assessed via on-site filtration and ultrafiltration or in situ dialysis (1 kDa membrane, see description in Pokrovsky et al., 2012; Vasyukova et al., 2010).
All chemical analysis of dissolved load were conducted at the GET laboratory in Toulouse using routine procedure for cations, anions, DOC, Si and trace elements (see Pokrovsky et al., 2012; Vasyukova et al., 2010). Sample preparation was performed in the clean room and international certified samples of river and rain water and soil and sediments were routinely measured along with collected samples (Pokrovsky et al., 2005a, b, 2006).
3 Results
3.1 NW Russia, non-permafrost zone
3.1.1 Sources of elements in the river water
The chemical composition of the river water is controlled by the hydrological regime and results from the mixing of rain, snow melting and groundwater input. This implies insignificant effect of:
- • release of elements in the river water from the suspended particulate material (SPM) and the bed sediments;
- • precipitation of secondary carbonate or silicate mineral in the river channel;
- • biological uptake of petrogenic elements in the river channel by the peryphyton, phytoplankton or macrophytes.
It has been widely argued that, besides atmospheric precipitates, bedrocks and soil minerals, important sources of dissolved major and trace elements in rivers can be degrading plant litter (Glazovskaya, 1956; Kovda, 1956; Pokrovsky et al., 2005a, b, 2006; Polynov, 1944). Indeed, studies of granite (Millot et al., 2002, 2003) and basalt environments (Moulton and Berner, 1998; Moulton et al., 2000) demonstrated that the chemical denudation rate can be several times higher in vegetated areas than that on the bare rocks.
The key parameter for estimating the litter degradation impact on dissolved element transport is the ratio between the export flux (J), or the annual loss of elements from degrading litter in soil horizon towards the river and the net primary production (NPP). The NPP here means the annual biomass production which is equal to organic matter mineralization under condition of constant forest biomass and no accumulation of OM in soils. The J/NPP ratio is extremely low for biogenic elements such as N, C, and P (0.01–0.05) but reaches 0.88 for mineral components (Ca, Mg, Na, K, Fe, Al, Si…) in boreal taiga region as estimated by Bazilevich (1976) based on long-term mass balance studies of Remezov et al. (1959) and Rodin and Bazilevich (1965) in Russian boreal zone (Glazovskaya, 1977). Assuming steady-state conditions of forest biomass and soil organic layer thickness and converting J/NPP to element content in the dry biomass, major element annual fluxes linked to litter degradation can be evaluated (Flitter). The element content in the dry biomass was taken from Kovda (1956) and compiled with more recent data (Viers et al., 2012; Zakharova et al., 2007). Because the element concentration in the plant biomass varies significantly, we always used the lowest reported range in order to assess the minimal possible value of Flitter.
To estimate the relative proportion of various sources to river cationic composition on the annual scale, multi-annual fluxes of rivers dissolved components together with atmospheric and underground inputs were used. The last two parameters were assessed from systematic multi-annual monitoring of atmospheric precipitation chemical composition conducted by Russian Hydro meteorological Survey and by quantifying the minimal river discharge during baseflow (winter) period (Zakharova et al., 2005, 2007), respectively.
The overall flux can be represented as:
(1) |
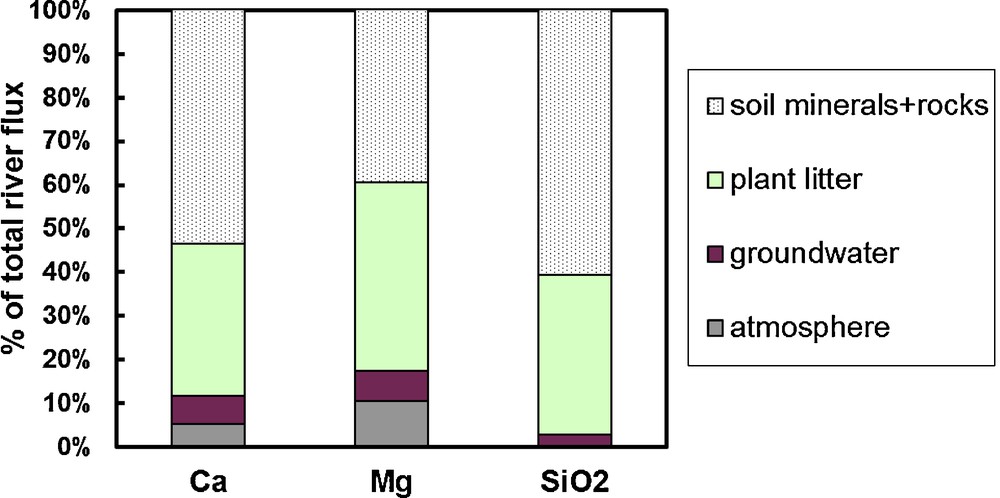
Contribution of different sources to annual fluxes of dissolved silica and cations in typical river of the Karelia region (White Sea basin, River Letnaya). The values of annual plant litter production used are 640 t/km2/y, corresponding to NPP = 320 t C/km2/y; J/NPP = 0.88 and the element content in dry biomass are 1, 0.3, and 1 g/kg for Ca, Mg, and SiO2.
Contribution de différentes sources aux flux annuels de silice dissoute et de cations dans la rivière typique de la région de Karelia (bassin de la Mer blanche, rivière Letnaya). Les valeurs de la production annuelle de litière utilisée sont de 640 t/km2/an ; correspondant à NPP = 320 t C/km2/an ; J/NPP = 0,88 et la teneur en éléments de la bio-masse sèche est de 1, 0, 3 et 1g/kg pour Ca, Mg et SiO2.
3.1.2 Boreal organic- and organo-ferric colloids
Boreal aquatic zone is a kingdom of colloids, the main vectors of the transport of most trace insoluble and even major elements such as Ca. Compared to tropical and temperate zones, dissolved fraction of boreal zone waters is richer in Fe(III) and contains higher proportion of low molecular weight (LMW< 1 kDa) labile organic matter. Thus, ultrafiltration of tropical rivers revealed that up to 60% of DOC is present as HMW entities (Dupré et al., 1999; Viers et al., 1997) compared to only 10-40% of 10 kDa–0.22 μm DOC fraction in boreal and subarctic settings during baseflow (Guo and MacDonald, 2006; Pokrovsky et al., 2006; Prokushkin et al., 2011). High concentration of colloidal Fe in boreal streams is especially pronounced during spring freshet (100–150 μg L−1 in Siberian rivers, Bagard et al., 2011; 500 to 900 μg L−1 in Severnaya Dvina River, Pokrovsky et al., 2010a, b) and may be as high as 1000 to 2000 μg L−1 in small boreal streams (Björkvald et al., 2008).
The enrichment of boreal colloids in Fe(III) may be linked to shallower soil depth (within glacial landscape of NW Russia and Scandinavia) or the presence of permafrost in Siberia and Arctic zone that does not allow the development of extensive soils. As a result, mineral soil horizons containing abundant Fe2+-bearing minerals are located closer to the surficial waters thus capable providing oxygenated, organic-rich upper soil horizons with Fe2+ ions necessary to form organo-ferric colloids at the hyporheic zone of the stream (cf., Pokrovsky and Schott, 2002). The dominance of LMW organic matter in boreal colloids is due to small residence time of DOM in shallow soils that are active only during 2 to 4 summer months. The polymerization of humic material producing high molecular weight (HMW) organic colloids is thus hampered by fast leaching of plant litter degradation products during freshet and summer baseflow yielding abundant LMW< 1 kDa organic ligands. At the same time, the majority of DOM in the Arctic rivers is composed of recalcitrant soil-derived material and not autochthonous material released from algae (Dittmar and Kattner, 2003). An important factor of the intensive degradation of HMW organic matter leading to decrease of molecular size of DOM could be photo-oxidation, especially pronounced during the Arctic summer. Note that during the spring flood, the proportion of colloidal (1 kDa–0.22 μm) compared to conventionally dissolved (< 1 kDa) organic carbon (OC) fraction increases by a factor of 2 to 3 (Pokrovsky et al., 2010a, b; Prokushkin et al., 2011).
The nature of colloidal material in the boreal zone comprises: i) HMW (10 kDa–0.22 μm) Fe-rich entities where the Fe oxy(hydr)oxides are stabilized by organic ligands; and ii) LMW (< 1–10 kDa), Fe-poor, organic-rich material. Respective role of both entities in speciation of trace elements (TE) has been first described based on size fractionation experiments conducted along a 2000-km latitude transect in NW Russia (Pokrovsky and Schott, 2002).
The presence of two groups of colloidal material in river waters has been further confirmed in a number of high-resolution chromatographic studies (Stolpe and Hassellöv, 2007, 2010; Stolpe et al., 2010). Recently, it has been demonstrated that the nature of mineral substrate has insignificant impact on TE distribution among colloids of different size fractions as illustrated in a plot of the relative proportion of colloidal Ca and U in 10 kDa–0.22 μm, 1 kDa–10 kDa and < 1 kDa pools measured on granite and basalt watersheds (Fig. 3 and Vasyukova et al., 2010). Moreover, the effect of DOC and Fe concentration and landscape setting on the relative percentage of colloidal versus truly dissolved fraction seem to be of secondary importance compared to the season of the year and the type of vegetation (Ilina, 2012). As a result, considering optimal period of sampling – summer baseflow – all boreal and subarctic streams may exhibit a universal character of TE distribution among different colloidal pools. We calculated the average value of colloidal (1 kDa–0.22 μm) proportion in non-permafrost, Fe-, organic-rich surface waters based on measurements of more than 20 sites collected in July–August (Table 1). Although these values are subjected to significant uncertainty, they can be used for the first-order assessment of colloidal status of chemical elements across the boreal and subarctic zone.
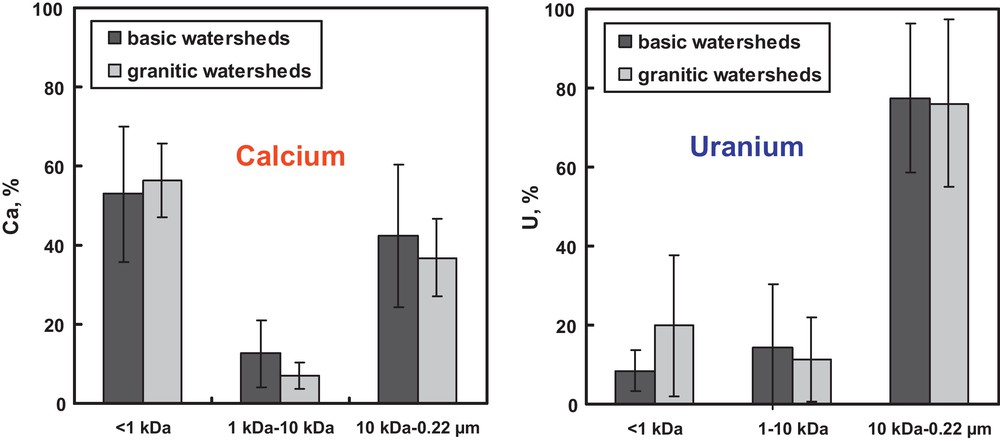
Ca (left) and U (right) distribution between LMW fraction (< 1 kDa) and two colloidal pools (1-10 kDa and 10 kDa – 0.22 μm) assessed for acidic- and basic rock-dominated catchments. The data represent the average (± 2 s.d.) of 9 and 5 samples for basic and granitic catchments, respectively.
Distribution de Ca (à gauche) et U (à droite) entre la fraction LMW (< 1 kDa) et deux groupes colloïdaux (1–10 kDa et 10 kDa – 0, 22 μm), évalués pour des bassins à dominance de roches acides et basiques. Les données représentent la moyenne (± 2 s.d.) de 9 et 5 échantillons de bassins basiques et granitiques, respectivement.
Valeurs moyennes du pourcentage de colloïdes (fraction 1 kDa - 0, 22 μm, comparée à la fraction < 0, 22 μm) dans 22 échantillons de fleuve boréal et d’eau de surface du Nord-Ouest de la Russie, mesurées en utilisant la dialyse et l’ultrafiltration pendant le flux de base estival (Ilina, 2012; Pokrovsky and Schott, 2002; Vasyukova et al., 2010). L’incertitude représente 2σ à p = 0,95.
Element | % colloidal | Element | % colloidal |
DOC | 60 ± 12 | Zr | 76 ± 12 |
B | 9 ± 1 | Mo | 28 ± 8 |
Li | 17 ± 10 | Cd | 38 ± 5 |
Na | 11 ± 10 | Sb | 34 ± 10 |
Mg | 25 ± 10 | Cs | 24 ± 11 |
Al | 65 ± 15 | Ba | 35 ± 9 |
Si | 5 ± 4 | La | 77 ± 10 |
K | 14 ± 5 | Ce | 81 ± 9 |
Ca | 30 ± 14 | Pr | 79 ± 8 |
Ti | 71 ± 13 | Nd | 78 ± 10 |
V | 45 ± 15 | Sm | 78 ± 9 |
Cr | 52 ± 16 | Eu | 71 ± 8 |
Mn | 39 ± 11 | Gd | 72 ± 12 |
Fe | 86 ± 5 | Tb | 78 ± 5 |
Co | 50 ± 9 | Dy | 73 ± 11 |
Ni | 40 ± 16 | Ho | 72 ± 9 |
Cu | 37 ± 15 | Er | 70 ± 9 |
Zn | 25 ± 19 | Tm | 70 ± 5 |
Ga | 61 ± 7 | Yb | 68 ± 9 |
Ge | 52 ± 13 | Lu | 66 ± 4 |
As | 39 ± 7 | Hf | 74 ± 12 |
Rb | 10 ± 4 | W | 41 ± 27 |
Sr | 26 ± 9 | Pb | 75 ± 13 |
Y | 73 ± 12 | Th | 80 ± 11 |
U | 65 ± 19 |
The generic scheme of colloids origin in surface waters (Fig. 4) includes massive leaching from uppermost surface organic horizons during springflood and mixing of underground anoxic waters with organic-rich oxygenated surface horizons during summer time. The latter process can also proceed in hyporheic zone of the river, a region beneath and alongside a stream bed, where there is a mixing of shallow groundwater and surface water (Pokrovsky and Schott, 2002). Note that in the permafrost zone, colloids generation involves soil solutions from mineral-rich deep soil horizons rather than shallow groundwaters (Bagard et al., 2011; Pokrovsky et al., 2006).
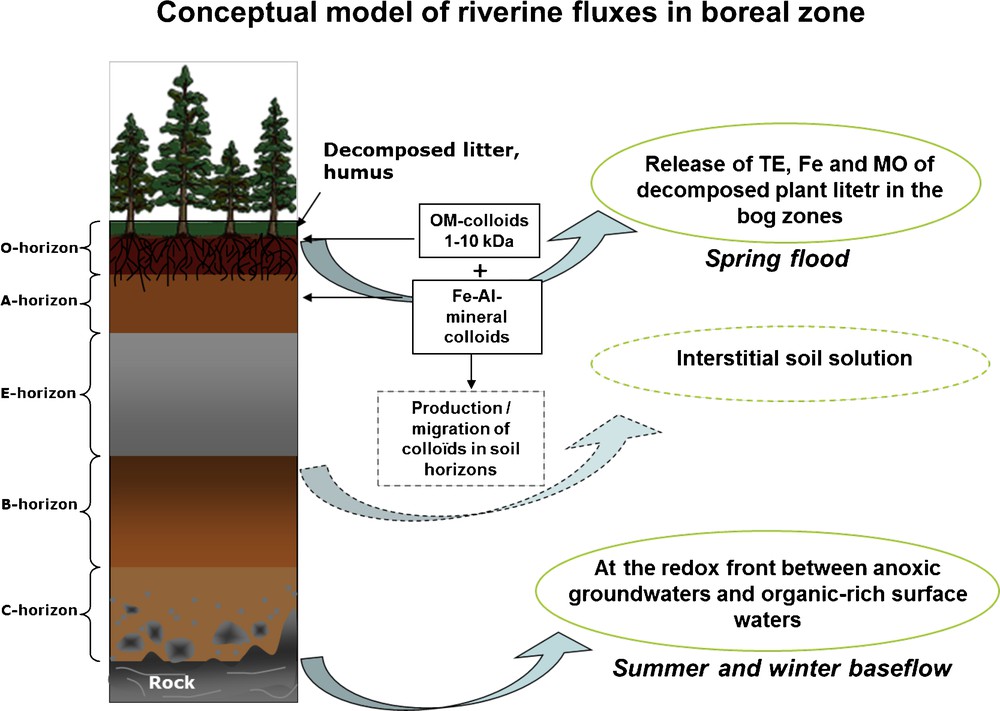
Generic scheme of colloids origin in boreal surface waters. The main source of colloidal material during the springflood is degrading plant litter at the organic-rich surface horizons. Formation of colloids during the summer baseflow occurs at the redox front between anoxic groundwaters and DOM-rich surface waters; this may happen in the hyporheic zone of the river or its riparian zone.
Schéma générique de l’origine des colloïdes. La principale source de matériel colloïdal pendant la crue de printemps est la dégradation de la litière dans les horizons organiques de surface. La formation de colloïdes pendant le flux de base estival se produit au front redox, entre les eaux souterraines anoxiques et les eaux de surface riches en DOM ; ceci peut se produire dans la zone hyporhéique ou riparienne de la rivière.
Similar to rivers, several pools of different colloidal material controlling speciation of TE are encountered in boreal lakes (Pokrovsky et al., 2012). In these settings, the origin of organo-mineral colloids includes:
- • heterotrophic degradation of sinking organic detritus with Fe (II) liberation from the biomass and oxidation in the presence of allochthonous and autochthonous DOM;
- • diffusive flux of Fe2+ from the anoxic sediments to the oxygenated epilimnic waters and Fe oxidation at the chemocline.
Compared to colloids from forested watersheds, glacial lake colloids are richer in Fe and poorer in Al. During the lake overturn, occurring in spring at high water level period, deep anoxic Fe(II)-rich waters are being mixed with oxygenated organic-rich surface waters additionally enriched by allochthnous dissolved organic matter delivered to the lake from its watershed, thus producing another pool of Fe-rich organic colloids. Similar to summer-time river-borne colloids described previously, the main gouverning process is likely to be the coprecipitation of trace elements with Fe(III) oxy(hydr)oxides stabilized by DOM. This spring and autumn pools of lake colloids are especially important for feeding the rivers by colloids within the previously glaciated terrain, such as Scandinavia and NW Russia, where numerous small rivers flowing to the sea are originated from the lakes.
Recently, the relative proportion of colloidal (1 kDa–0.22 μm), conventionally dissolved (< 1 kDa) and suspended (> 0.22 μm) element fluxes from the land to the Arctic ocean has become available for the largest European Arctic river, Severnaya Dvina (Pokrovsky et al., 2010b; Shevchenko et al., 2010). Ultrafiltration and dialysis, systematically conducted over all seasons of the year allowed integrated assessment of three main pools of element delivered to the ocean by this boreal river (Fig. 5). Note that the majority of insoluble elements (Fe, Al, REEs, Zr, Th) are transported both as colloidal and suspended fraction whereas U and organic carbon are delivered essentially in colloidal form. Divalent metals are equally distributed among < 1 kDa, colloidal and suspended fractions. The last result is important in view of micronutrients supply to the Arctic Ocean, given that the < 1 kDa fraction which is comparable with the poresize of cellular membranes, may be potentially bioavailable. Similar distribution of colloidal and dissolved annual fluxes has been observed for a large tributary of the Yenisey River (Bagard et al., 2011; Prokushkin et al., 2011). To our knowledge, such kind of information covering full period of the year is not available for other large temperate and tropical rivers. Therefore, rigorous comparison of the degree of colloidal transport to the Arctic Ocean and to the other oceans is at present impossible. However, it can be speculated that, normalized to its relatively small volume and surface area, the Arctic Ocean receives by far the highest colloidal element flux from the land, and this colloidal input occurs during 1 to 2 months of the spring flood.
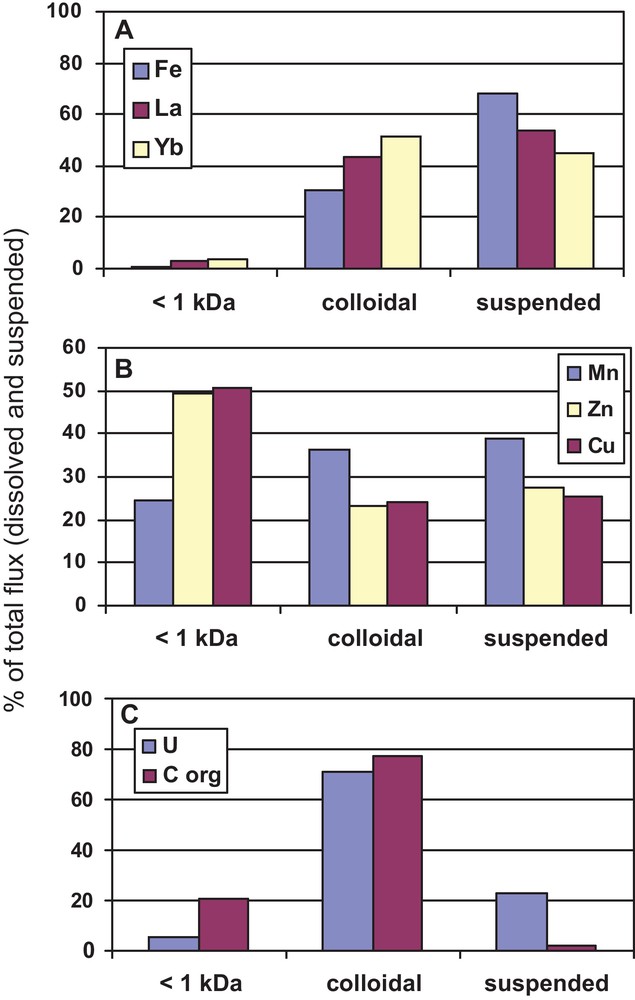
Relative proportions of truly dissolved (< 1 kDa), colloidal (1 kDa – 0.22 μm) and suspended (> 0.22 μm) fluxes in Severnaya Dvina river during spring flood in May. A, Typical insoluble elements such as Fe, Co, (Ni), Cr, Y, all REEs, Cd, Zr, Pb, (Hf) and Th, whose transport increases due to the presence of colloidal forms (15–50%), associated with a negligible amount of truly dissolved (0–5%) and a major contribution of suspended fluxes (40–70%); B, Divalent transition metals (Mn, Cu and Zn) with high affinity for biological ligands, being equally distributed among the three pools; C, Uranium and organic carbon exhibit the largest (70–75%) relative proportion of the colloidal pool, with low dissolved and suspended fluxes.
Proportions relatives des flux réellement dissous (< 1 kDa), colloïdal (1 kDa–0, 22 μm) et en suspension (< 0,22 μm)dans la rivière Severnaya Dvina pendant la crue de printemps en mai. A. Élements insolubles typiques tels Fe, No, (Ni), Cr, Y, tous les REE, Cd, Zr, Pb, (Hf) et Th dont le transport augmente en raison de la présence de formes colloïdales (15–50 %), associées à une proportion négligeable de flux réellement dissous (0 – 5 %) et à une contribution majeure de flux de matériel en suspension (40–70 %). B. Métaux bivalents de transition (Mn, Cu, Zn) avec une forte affinité pour les ligands biologiques, également distribués entre les trois groupes. C. L’uranium et le C organique sont les plus largement représentés dans le pôle colloïdal, avec des flux de matériel dissous et en suspension bas.
3.1.3 Estuary
The specificity of colloidal matter in the boreal rivers determines the transport of major and trace elements through the estuarine zones of the Artic Ocean. Whereas conventionally dissolved (< 0.22 or < 0.45 μm) organic carbon and Fe(III) concentrations decrease with the increase of salinity, following the classic coagulation scheme established for other organic-rich rivers, or exhibiting conservative behavior like it is the case of OC in large Arctic rivers (Dittmar and Kattner, 2003), the behavior of LMW<1 kDa fraction along the salinity gradient is dramatically different as it is illustrated for the Severnaya Dvina delta in Fig. 6. The concentration of LMW DOC remains virtually constant up to 15‰ of salinity whereas the concentration of LMW Fe increases by a factor of 5 in the end of the mixing zone compared to the freshwater end-member. This pattern can be linked to:
- • LMW form production in the water column, due to phytoplankton metabolism;
- • DOM restructuring via photodegradation;
- • degradation of DOM via heterotrophic bacterioplankton respiration;
- • OC and TE mobilization (desorption) from the sediments and suspended matter with the increase of salinity.
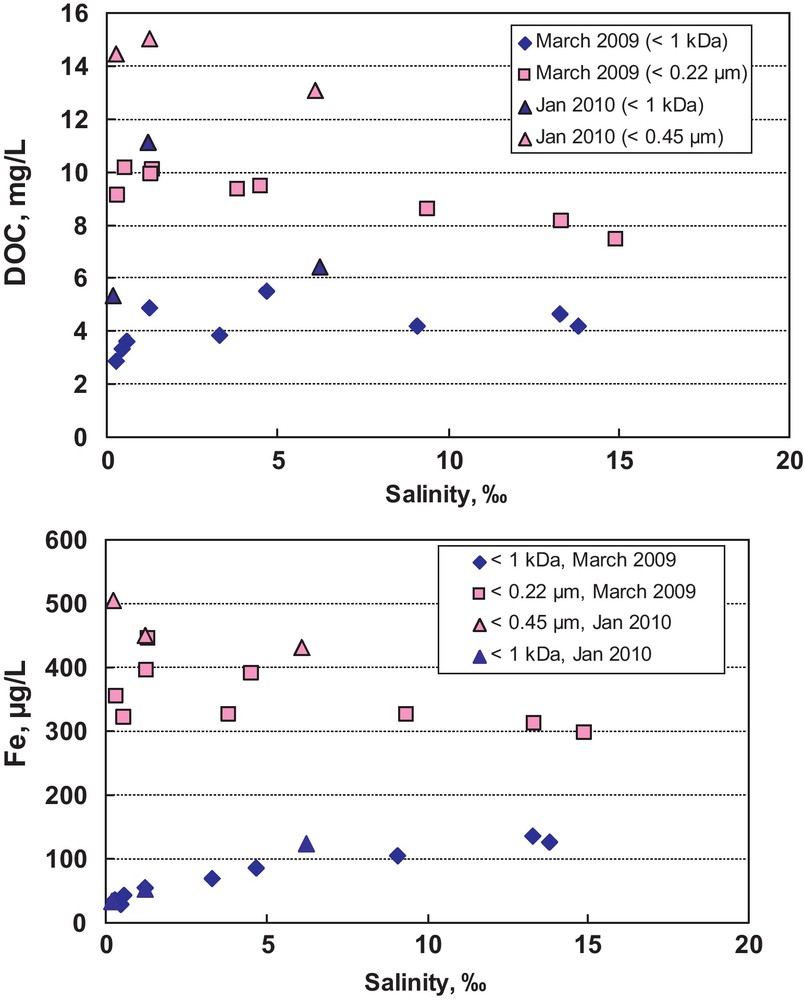
Plot of conventionally dissolved (< 0.22 or < 0.45 μm, red symbols) and LMW< 1 kDa (blue symbols) concentrations of organic carbon (top) and iron (bottom) as a function of salinity in January and March 2009–2010 (Severnaya Dvina estuary). While conventionally dissolved fraction exhibits slightly non-conservative behavior, the LMW fraction remains constant (DOC) or increases by a factor of 5 (Fe) with the salinity increase from 0 to 15‰.
Diagramme représentant les concentrations de carbone organique (en haut) et de fer (en bas) en éléments conventionnellement dissous (< 0, 22 ou < 0, 45μm, symboles rouges) et en LMV < 1 kDa (symboles bleus), en fonction de la salinité en janvier et mars 2009 à 2010 (estuaire de la Severnaya Dvina). Tandis que la fraction conventionnellement dissoute montre un comportement légèrement non conservatif, la fraction LMV reste constante (DOC) ou augmente d’un facteur de 5 (Fe), quand la salinité augmente de 0 à 15 ‰.
At present, one cannot quantitatively distinguish between these possible processes. In any case, given that this LMW is most labile, and, potentially, bioavailable, this result may have important consequences on OC and trace metal regulation of the Arctic Ocean productivity, making it different from the other oceans. Unfortunatley, the absence of comparable data on < 1 kDa fraction as a function of salinity in the mouth zones of other large rivers of the world does not allow straightforward comparison of element fluxes transformation between various climatic zones.
3.2 Central Siberia larch forest over continuous permafrost
3.2.1 Element sources and seasonal variations in large rivers
The central Siberian basaltic plateau underlain by continuous permafrost and covered by abundant larch forests offers a unique natural site for assessment of the contribution of different sources of elements to the rivers (Bagard et al., 2011; Pokrovsky et al., 2005a, b, 2006; Prokushkin et al., 2011). Similar to the non-permafrost zone, the majority of the OC flux occurs during the freshet whereas HCO3− flux, reflecting inorganic CO2 consumption during weathering, is similar for spring flood and summer baseflow but negligibly small during the winter period (Fig. 7). The majority of the metal (Fe, Al, other low mobile trace elements) flux occurs during the spring flood that lasts less than a month and contributes up to 60 to 80% of the total water discharge to the ocean. In contrast, the winter baseflow lasting from October to May and delivering only 10 to 15% of annual water discharge, still contributes to c.a. 35% of annual Ca flux. This is certainly linked to high concentration of Ca and other major components in the river water during winter, when Siberian rivers are fed by taliks connected to groundwater reservoirs of fluids. During this period, the TDS increases to ≥1 g L−1.The contribution of spring flood to the annual Ca(HCO3)2 flux is around 30%. During these 2-3 weeks of the freshet, the mineral soil is still frozen and the sources of solutes in river are mostly upper organic-rich soil horizon and degrading plant litter. The intensity of HCO3− leaching in the form of CO2 emission from the plant litter depends on the nature of organic substrate and the activity of soil microorganisms. As such, significant proportion of annual CO2 consumption during basalt weathering should be linked to the plant litter degradation in topsoil organic layers rather than to direct dissolution of parent rocks in subsoil interstitial solutions. As a result, the main response of the system to climate warming seems to be in the change of element uptake flux by roots which is merely linked to the plant biomass rather than to the change of soil hydrological or chemical regimes (see section 3.2.2 below).
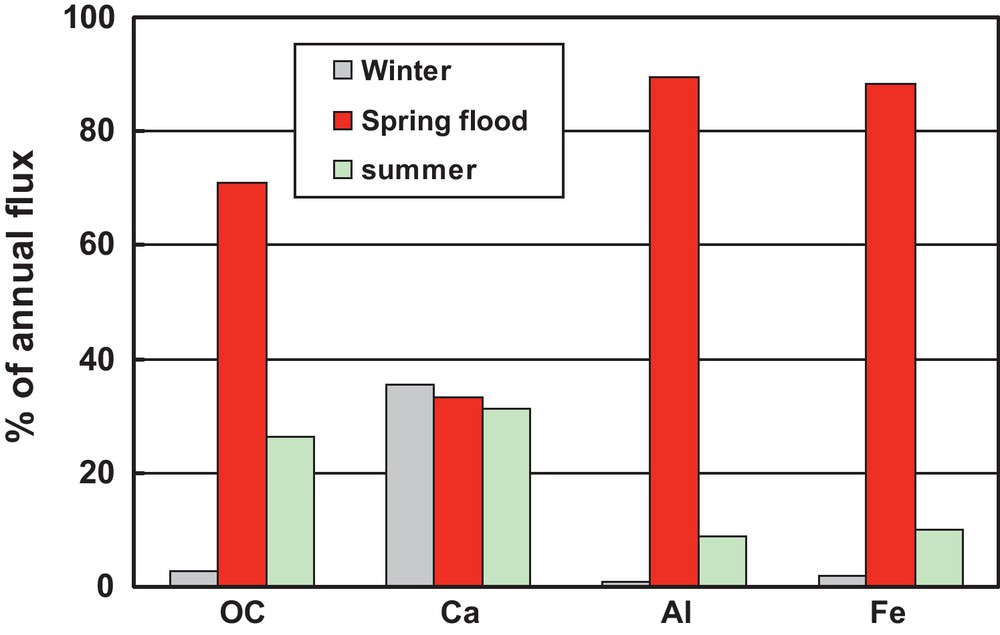
Stack diagram of the relative contribution of three main hydrological seasons to total annual flux of organic carbon (OC), Ca, Al and Fe in the Kochechumo River draining central Siberian basalts.
Diagramme d’ensemble montrant la contribution relative des trois saisons hydrologiques principales au flux total annuel de carbone organique (OC), Ca, Al et Fe dans la rivière Kochechumo drainant les basaltes de Sibérie centrale.
Moreover, the annual dissolved carbon flux from the watershed reflecting the total CO2 consumption by vegetation and chemical weathering, is largely dominated by organic carbon constituting in average 80% of the total C flux (Fig. 8). It means that only 20% of atmospheric carbon is directly linked to basalt mineral dissolution whereas the major part of carbon uptaken from the atmosphere is transported by the river to the ocean in the form of plant litter degradation products. The particularity of Siberian larch forests capable providing essential part of riverine element fluxes is that it is developed on permafrost terrain. Coniferous larch species shed needles in September when the element uptake by roots is limited as snow cover appears and soil starts to freeze. Since the aqueous reactivity of plant biomass is the highest at the very beginning of reaction (i.e., Fraysse et al., 2010), the litter degrades very fast the late autumn and in the next spring during the massive snow melt. At this period, both mineral and organic layers of soil, especially the rooting zone, are still fully frozen. The release of elements from larch needles happens directly at the site of deposition, under the tree canopy, and further continues in the riverwater, if, during the high water level period, the needles are transported by the meltwater. As a result, compared to temperate or non-permafrost forests, the recycling of elements from the degrading litter back to the plants is smaller, and, consequently, the fraction of element that can be released to the river is significantly higher.
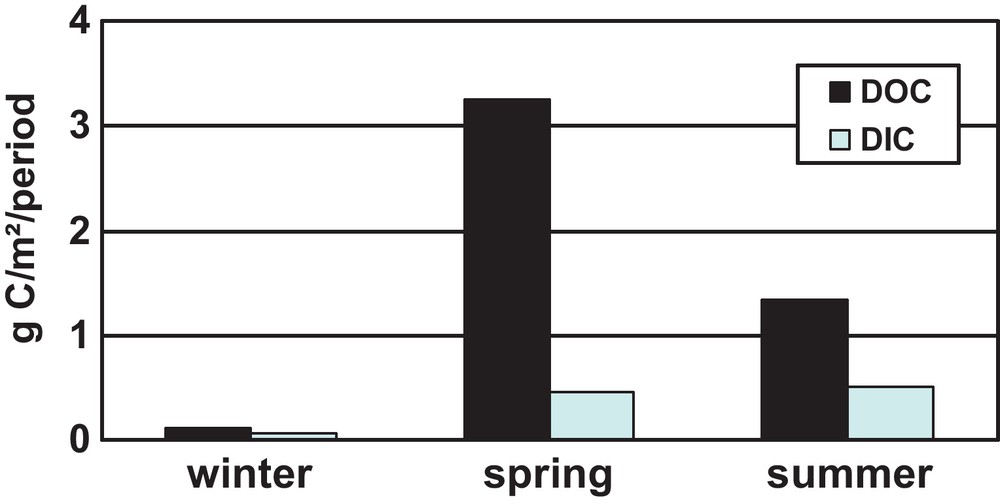
Stack diagram of the contribution of three main hydrological seasons to the annual flux of organic and inorganic carbon in the Kochechumo River, central Siberian basalts. The majority of the CO2 uptaken from the atmosphere at the watershed is transported in the form of dissolved organic carbon during several weeks of the freshet. As such, the direct role of chemical weathering of rocks in the total annual atmospheric CO2 consumption by Siberian basalts is negligible (≤ 20%).
Diagramme montrant la contribution des 3 principales saisons hydrologiques au flux annuel de carbone organique et inorganique dans la rivière Kochechumo, drainant les basaltes de Sibérie centrale. La plus grande partie du CO2 prélevé à partir de l’atmosphère au niveau du bassin est transportée sous forme de carbone organique dissous, pendant plusieurs semaines de l’avalaison. Ainsi, le rôle direct de l’altération chimique des roches dans la consommation totale annuelle du CO2 par les basaltes de Sibérie est négligeable (≤ 2 %).
It is important to note that the majority (c.a. > 85%) of annual trace metal flux in central Siberian rivers occurs during freshet following the OC flux. The transport of insoluble trace metals in Siberian rivers is linked to Fe and Al as major constituents of river organo-mineral colloids (Bagard et al., 2011; Pokrovsky et al., 2006). Given that this flux is mainly formed when the mineral soil is frozen, the importance of plant litter in providing the majority of dissolved trace metals is incontestable as also supported by mass balance calculations (Pokrovsky et al., 2006). As such, while the climate warming will certainly affect the winter-time element fluxes and speciation, it is unlikely to change the nature and magnitude of the TE fluxes to the ocean which occur in colloidal form during several weeks of the springflood.
3.2.2 South-facing versus north-facing slopes and relative role of vegetation
The importance of element pool stored in vegetation for chemical denudation of central Siberian basalts within the context of climate change can be also assessed from comparison of south-faced and north-faced slopes within a small watershed (Fig. 9). Among the basic techniques of experimental assessment of the climate warming effect – soil heating (cf. Kirschbaum, 2004), latitude gradient analysis (cf. Frey et al., 2007) and aspect variations – the approach “substituting space for time” using slopes of different orientations is by far the most straightforward and elegant one. These habitats, developed exactly on the same bedrocks exhibit identical chemical and mineralogical composition of soils, precipitation and canopy vegetation. However, they differ in terms of the amount of solar radiation received during the growing period, and, as a result, exhibit drastically different rooting and seasonal thaw depth, the larch biomass and the NPP (by a factor of 2 to 3). Rigorous assessment of plant chemical composition in the two habitats and the evolution of the needles chemical composition in the course of growing season demonstrated, based on robust statistics, that the element composition of larch foliage systematically changes in the course of growing season but the difference between south and north-facings slope is smaller than the difference between the seasons (Viers et al., 2012). There is no significant difference in soil chemical composition between different environments and the partitioning coefficients of more than 40 major and trace element between mineral soil and plant biomass are virtually the same for warm and cold habitats. However, the resorption efficiency for many elements (e.g. N, P, K, Cu, Zn etc.) is a factor of 1.5-3 higher in the cold habitats compared to the warm ones.
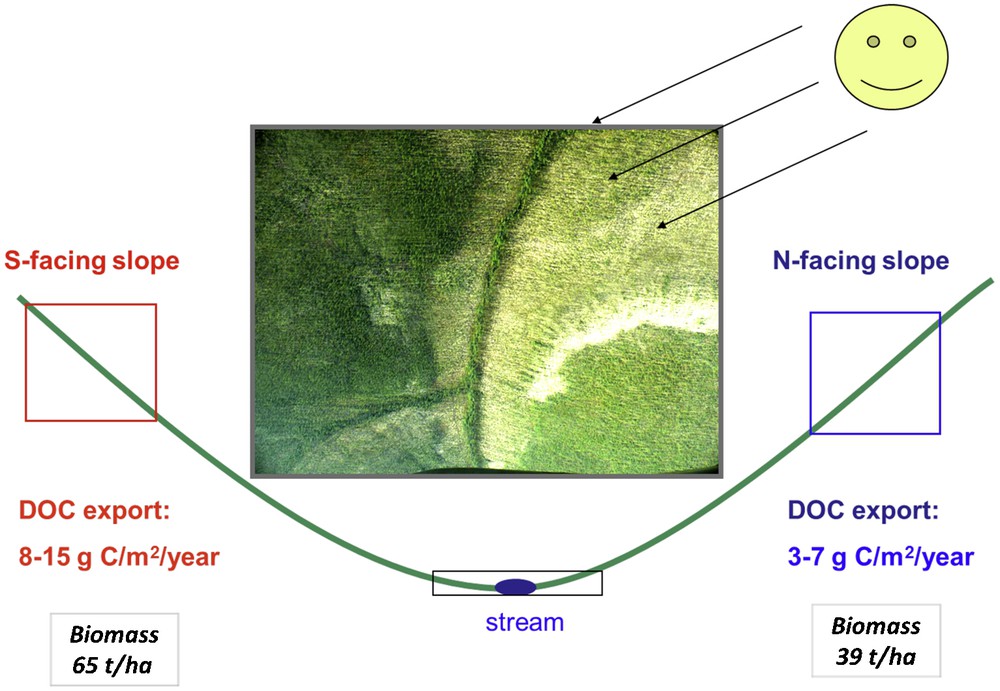
Scheme of south-facing and north-facing slopes within a small central Siberian watershed depicting significantly higher biomass (greener area) and export fluxes on the south-facing slope compared to north-facing slope.
Schéma des pentes exposées au sud et au nord dans un petit bassin de Sibérie centrale, montrant de manière significative une plus forte teneur en biomasse (zone verte) et des flux d’export plus importants sur la face exposée au sud que sur la face exposée au nord.
3.3 Western Siberia thaw lakes as mediators of CO2 flux from soil to the atmosphere
The processes of chemical weathering in western Siberia plain are dramatically different from those in the other parts of Siberia due to the dominance of thick (up to 3 m) peat deposits overlain already weathered Neocene sands (Audry et al., 2011; Pokrovsky et al., 2011; Shirokova et al., 2012b). In this region, thawing of frozen peat creates numerous lakes and ponds whose draining to the hydrological network provides the majority of the element flux during open water period. As a result, the transport of carbon from the W. Siberia plain to the Arctic Ocean is essentially controlled by leaching of organic matter and related elements from the peat surface depressions, most often occurring in the thermokarst (thaw) lakes and ponds. In these aquatic environments, soil carbon can be converted to CO2 and/or CH4 and released to the atmosphere, deposited at the lake bottom or delivered to the river as dissolved DOC or DIC, the latter exhibiting significantly smaller proportion in the annual carbon flux. In this regard, western Siberia plain may turn out to be dramatically different from other shallow freshwater ecosystems of the circumpolar Arctic (i.e., Kirpotin et al., 2009, 2011; Rautio et al., 2011). In fact, the total flux of C in the form of CO2 to the atmosphere from the surface of West Siberia thermokarst lakes may be a factor of 2 to 3 higher than the DOC flux of all Siberian rivers to the Arctic Ocean (Pokrovsky et al., 2011; Shirokova et al., 2009).
Recently, it has been shown that in western Siberia, the thaw ponds and depressions with a surface area of less than 1000 m2 exhibit concentration of CH4 and CO2 that is three to ten times higher, and a concentration of DOC that is two to three times higher, than those investigated previously in large thermokarst lakes (Shirokova et al., 2012b). Although all the investigated depressions, ponds, and lakes are supersaturated with respect to atmospheric CO2, the supersaturation degree is the highest for the small peat depressions formed through subsidence caused by degradation of ice-rich permafrost (Fig. 10). Significant increase in CO2, CH4 and DOC concentrations with decreasing surface area is pronounced for surface areas that are < 1000 m2, being maximal for small thermokarst depressions with 1 to 100 m2 of surface area (Fig. 10). These small and very shallow water bodies are extremely abundant, virtually invisible by remote sensing, and absent on available topographic maps or on the fine-scale lake databases (Downing et al., 2006). These depressions may turn out to be very important mediators of the transformation of old peat carbon into DOC, which is then respired by aerobic heterotrophic bacteria into CO2 (Shirokova et al., 2009).
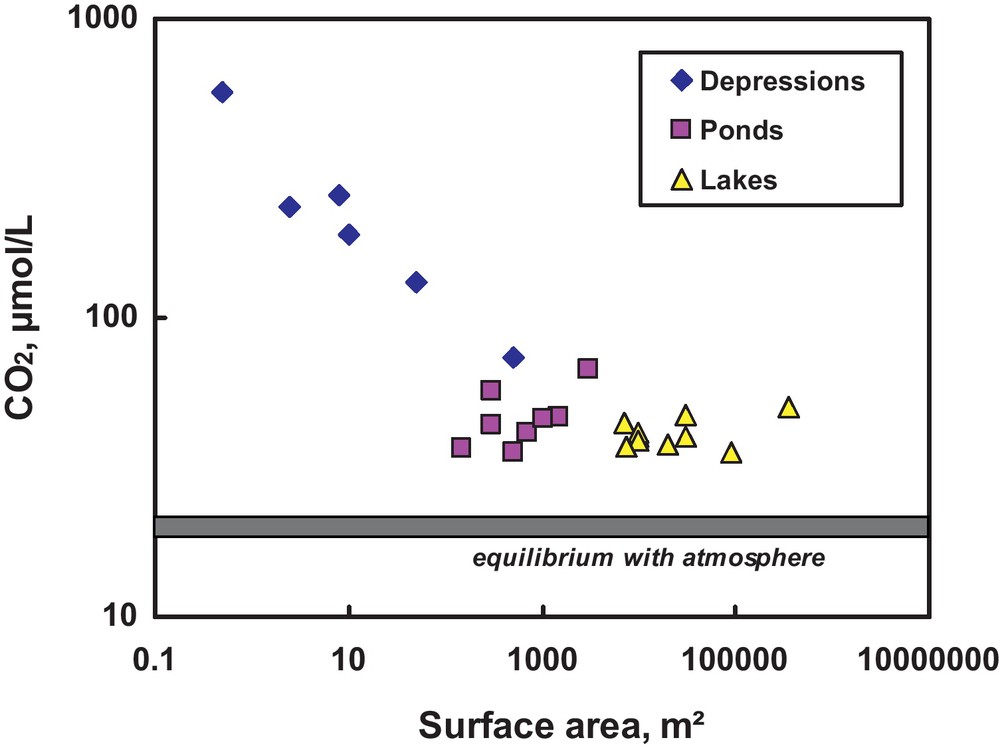
CO2 concentrations as a function of water body surface area in western Siberia, the thermokarst region. The symbol size reflects the value of the uncertainty. Note that all lakes of W Siberia are strongly supersaturated with respect to the atmosphere.
Concentrations de CO2 en fonction de la surface du corps aqueux en Sibérie occidentale, la région thermokarstique. La taille du symbole reflète la valeur de l’incertitude. À noter que tous les lacs de Sibérie occidentale sont très sursaturés par rapport à l’atmosphère.
Similar to aqueous CO2, there is a systematic decrease of total DOC (< 0.45 μm) from small depressions, to thaw ponds, to thermokarst lakes, and finally, to rivers. This tendency is in agreement with data from other discontinuous permafrost regions (e.g. Alaska) where the thermokarst depressions formed from permafrost thaw contained up to 37.5 mg L−1 of DOC, as compared to the typical values in permafrost-affected rivers (2 to 6 mg L−1, Balcarczyk et al., 2009). Aqueous concentrations of major and most TE also decrease with the increase of the water body size corresponding to progressive consumption of humic colloids originated from peat abrasion by heterotrophic aerobic bacterioplankton (Pokrovsky et al., 2011; Shirokova et al., 2009). The mechanisms of CO2, CH4, DOC, major and trace element enrichment in small thermokarst depressions compared to common boreal lakes may include:
- • more intensive bottom sediment respiration of fresh peat deposits;
- • shallow water depth and less pronounced methanotrophy in the water column;
- • high contribution of peat abrasion at the border to the DOC delivery to the lake;
- • die off and decay of moss and lichen biomass under water;
- • the fast release of DOC and related elements from submerged vegetation (mosses and lichens) at the beginning of the thaw.
4 Discussion: possible effect of climate warming on biogeochemical fluxes of major and trace elements in high latitudes
Because element riverine fluxes reflecting chemical weathering in Boreal and Subarctic zone are tightly linked to vegetation productivity and plant litter degradation, the changes in biota reservoir will be by far the dominant factor of flux modification under climate warming scenario. Analysis of major and TE evolution in the needles of larch, the dominant coniferous deciduous tree of Siberia, demonstrated rather weak difference of chemical composition between cold and warm habitats (Viers et al., 2012). As such, the main consequences of climate warming on plant-related biogeochemical cycles in Siberia seem to be the increase of element pool accumulated in plant biomass. This increase should be at least a factor of 2 judging from the difference in the biomass and NPP between south-facing and north-facing slopes. Given that the flux of the elements in rivers is proportional to the litterfall, the export fluxes of elements to rivers from the watershed, or the chemical weathering may as well double their intensity. This is consistent with direct measurements of the export of DOC from south-facing slopes (8–15 g C m−2y−1) which is a factor of two higher than that from the north-facing slopes (3–7 g C m−2y−1, Prokushkin et al., 2010). Because the dissolved organic matter is a main vector of many elements transport in boreal surface waters, the increase of both biomass and rooting depth with temperature increase will at least double the fluxes of trace elements present as organic colloids. Moreover, given that the resorption efficiency of many major and trace elements is lower in warm habitats compared to cold habitats (Viers et al., 2012), the possible weathering increase will be significantly amplified (up to a factor of 5, depending on the element) since the resorption will become less pronounced.
Note that the change in the intensity of chemical weathering under climate warming scenario as being linked to the change of pCO2 and dissolved organics in soil solution (i.e., Beaulieu et al., 2012) is unwarranted. First, the majority of dissolved flux occurs during the freshet, when the soil is mostly frozen. During this period, even if the first 0-10 cm of organic-rich layer is thawed, the full depth of mineral horizon is still inactive. Second, the dissolution rate of major rock-forming minerals responsible for Ca and Mg release and associated CO2 consumption is not affected neither by pCO2 (Golubev et al., 2005) nor by the presence of organic ligands and heterotrophic microbiological activity in soils (Golubev et al., 2006; Golubev and Pokrovsky, 2006; Pokrovsky et al., 2005b, 2009, 2010a; Oelkers et al., 2011; Shirokova et al., 2012a; Stockmann et al., 2012). As such, the change of soil hydrological regime and the chemical composition of interstitial soil solution within the mineral horizon, although being affected by temperature and precipitation change, will be of secondary importance compared to plant litter fall fluxes and litter degradation intensity change occurring in the organic-rich surface horizons.
The colloidal regime and major and TE distribution among various size fractions are unlikely to be affected by DOC level, or by the change in hydrological regime of surface waters. This follows from results of thorough study of aqueous colloids in small watersheds of basaltic and granitic lithology under various landscapes (rivers, bogs, soil depressions) and dissolved Fe and DOC concentrations (Ilina, 2012; Vasyukova et al., 2010). At the same time, the decrease of pH of boreal surface waters due to local acidification or climate warming (Neal et al., 2008; Reuss et al., 1987), and the increase of phytoplankton and periphyton activity in rivers and lakes (Pokrovsky et al., 2012) should produce an increase of the proportion of < 1 kDa element pool compared to colloidal (1 kDa–0.22 μm) pool (Vasyukova et al., 2012). This should certainly increase the relative element bioavailability in river and element mobility through the estuarine mixing zone to as much as a factor of 2. Similar conclusion is true for boreal lakes whose warming should amplify the intensity of OC and TE LMW< 1 kDa complexes production via heterotrophic mineralization and photodegradation of large-size allochthonous DOM. However, the degree to which the rising water temperature affects these processes is not yet quantified.
The recent finding that CO2, CH4, and DOC concentrations increase with decreasing thermokarst lake age and size may have dramatic consequences on the effect of on-going permafrost thaw on carbon cycle in aquatic ecosystems of western Siberia. The increase in the number of small water bodies, accompanied by the drainage of large thermokarst lakes to the hydrological network, will likely favour:
- • the increase of DOC and colloidal metal stocks in surface aquatic systems, but also their potential transport to the Arctic Ocean;
- • the enhancement of CO2 and CH4 fluxes from the water surface to the atmosphere.
According to a conservative estimation that considers that the total area occupied by water bodies in western Siberia will not change, this increase in stocks and fluxes could be as high as a factor of ten over the territory of several million km2.
5 Conclusions
A decadal study of major and trace element biogeochemistry in boreal and subarctic landscapes of northern Eurasia over a 12,000 km longitude and 3000 km latitude profiles covering the largest variety of pristine landscapes from southern taiga to arctic tundra allowed achieving first-order quantitative estimation of mechanisms controlling element fluxes and chemical erosion on watersheds. In terms of expected ecosystem change under on-going climate warming in high latitudes, the following preliminary conclusions can be suggested:
- • vegetation and landscape (aspect) position exhibit an overarching importance on element fluxes and speciation compared to the lithology of rocks;
- • plant litter decomposition (decay) and element turnover rates in terrestrial ecosystems are the primary factors controlling amounts and speciation of elements in riverine fluxes to the Arctic Ocean;
- • no significant change in element speciation in organo-mineral colloids is expected except if significant acidification of surface waters will occur;
- • the climate warming in high latitudes capable doubling the biomass and NPP may also increase the relevant element fluxes from the soil to the river by a factor of 2;
- • in the discontinuous permafrost and tundra wetland zone of high lake abundance, the short-term increase of the riverine fluxes at max. 3 to 5 times can be expected;
- • among 3 key factors of climate change–temperature, precipitation and biota – the plant productivity and community composition should be by far more important in enhancing element fluxes than the activation energy for chemical weathering (< 10%), soil pH and pCO2 change or the increase of the river discharge (max. 10–20%).
Acknowledgements
This work was supported by ANR “Arctic Metals”, LIA “LEAGE”, PICS No. 6063, GDRI “CAR WET SIB”, grants RFBR-CNRS Nos 12-05-91055, 08-05-00312_а, 07-05-92212-CNRS_a, 08-04-92495-CNRS_a, CRDF RUG1-2980-KR10, Federal Program RF “Kadry” (contract N 14.740.11.0935), and Programs of Presidium RAS and UrORAS.