1 Introduction
The immune system is providing us with a very powerful weapon against infection, which enables us to eliminate invading microorganisms and to kill infected cells. This is accomplished in part by lymphocytes that have randomly generated a great diversity of antigen-specific receptors. But there is an important price to be paid for this diversity, as it is essential to delete those lymphocytes aggressive towards self-constituents. How then does the immune system discriminate between what is self and what is nonself?
2 What is self?
Higher organisms can innately distinguish species self from the infectious nonself of micro-organisms by means of various pattern recognition molecules, such as complement, collectins, lipopolysaccharide-binding proteins, etc. [1]. This is a function of the nonadaptive immune system that has evolved primarily to recognize those molecular structures unique to bacteria. It provides a very powerful, although elementary discrimination between self and nonself. But are there any structural characteristics that might distinguish one individual of a species from any other individual of the same species? One might suggest the following. For the immune system, self might include all antigenic determinants or epitopes encoded in the individual's own DNA, all other epitopes being considered as nonself. It is, however, not possible to envisage any mechanism that would allow the immune system to scrutinize the entire germline DNA, its translation products and those that are subsequently modified. Could some unique structural properties be attributed to self-epitopes? In their book, The Production of Antibodies, Burnet and Fenner [2] stated: “Body cells carry ‘self marker’ components which allow recognition of their ‘self’ character. Antigens in general are substances of the same chemical nature as the marker components but of different molecular configuration”. But there is no evidence that primary or secondary structure alone is what determines the ability of the immune system to distinguish self from nonself. Attributes other than the mere structural characteristics of an epitope must also be sensed. Among these are the following:
- (a) stage of development of the individual;
- (b) state of maturity of the lymphocyte;
- (c) site of encounter: thymus, extra-thymic tissues;
- (d) type of cells presenting epitopes;
- (e) production of nonepitope products;
- (f) ‘Danger’.
3 Stage of development of the individual
This was suggested by Burnet and Fenner [2] and appeared logical as the immune system is usually faced with most self-components before birth and only later with nonself constituents. The classical experiments of Billingham et al. [3] certainly vindicated this idea. Thus injection of allogeneic cells in the adult induced an accelerated rejection to a subsequent skin graft from the same donor, whereas injecting these cells at birth or in fetal life induced specific tolerance to skin allografts. This phenomenon, known as immunological tolerance, could easily be accommodated in Burnet's clonal selection theory [4]: antigen encountered before birth deletes specific clones termed ‘forbidden clones’, whereas antigen encountered after birth stimulates the respective clones to proliferate and respond.
Implicit in this hypothesis, however, is the requirement for prenatal generation of the entire immune repertoire. This is not the case, since lymphocyte differentiation continues throughout postnatal life, and somatic mutation generates new B cell specificities after antigenic stimulation. Thus the key factor in determining whether tolerance or immunity results cannot be the development stage of the individual.
4 State of maturity of the lymphocyte
In 1959, Lederberg [5] suggested that the state of maturity of the lymphocyte at the time it encountered antigen was what determined responsiveness. Immature lymphocytes encountering antigen are deleted, whereas mature lymphocytes are activated to respond. Although strong evidence has been obtained to support this simple scheme, it does not agree with many experimental situations. Nossal, for example, failed to induce tolerance to influenza virus even after injection of the virus in utero in mice [6]. This failure was presumably due to rapid antigen clearance. On the other hand, tolerance to synthetic polypeptides or to the immunogenic form of a protein could easily be induced in adult mice [that have many mature lymphocytes] by preinoculation of the protein in deaggregated form [7]. Hence, contact of mature lymphocytes with antigen does not always lead to an immune response.
Because of the ease with which tolerance to foreign skin can be induced in neonatal mice, their lymphocytes were deemed immature. Thirty-two years ago, however, it was proved that neonatal T cells, from the spleens of one-day-old mice, could perfectly respond to foreign antigens [8]. Immature T cells are thus able to respond immunologically to the correct antigenic stimulus, but what about immature T cells in the thymus?
5 Site of encounter – intra-thymic tolerance
The first hint that the thymus may be a site of tolerance induction came from experiments with neonatally thymectomized mice. Such mice were first shown to be severely immunodeficient in 1961 [9], but when grafted with syngeneic thymus tissue, they were fully immunocompetent. They also became competent when they received allogeneic thymus grafts except insofar as they were specifically tolerant to tissues from the thymus donor [10]. The suggestion was made that the tolerance resulting from giving allogeneic cells at birth induced a ‘selective immunological thymectomy’, i.e. deleted those host thymus lymphocytes that were specifically reactive to the antigens on the donor cells [10], thereby implying that the thymus may be the seat where self-tolerance is acquired.
We now know that immature thymus T cells can either be deleted or selected for survival by the same self-epitope [peptide] [11]. Developing T cells are positively selected only if they can express antigen-specific T cell receptors [TCR] that allow them to bind with a certain degree of affinity to molecules encoded by the major histocompatibility complex [MHC] and present on thymic cortical epithelial cells. Such binding protects the cells from programmed cell death. Positive selection therefore ensures that the mature T cell will be able to recognize peptides loaded in the binding cleft of self-MHC molecules, and therefore will be self-MHC restricted. Positive selection will not, however, prevent the differentiation of those T cells expressing high affinity TCR for self-peptides and MHC molecules, i.e. potentially autoreactive T cells. Negative selection must therefore physically delete these cells as they encounter DCs and epithelial cells in the medulla [reviewed in [11]]. The affinity of the TCR for its target self-peptide and self-MHC molecule therefore dictates whether the selection will be positive or negative.
Where in the thymus does negative selection take place? At least three lines of experimental evidence have suggested that it may occur in the cortex. First, injection of moderate doses of peptide, superantigens or anti-TCR antibody has led to massive deletion of CD4+CD8+ double positive thymocytes that are predominantly located in the cortex [12]. But peptide-induced deletion of these cells was far less in neonatal mice, which suggests that deletion in the adult may have been related to the toxic effects of cytokines released after stimulation of mature T cells in the periphery. Second, in H–Y specific TCR transgenic mice, cortical thymocytes were deleted in response to the endogenous antigen H–Y [13]. But premature expression of abnormally high levels of the transgenic TCR may have been responsible for this deletion. Third, antigen-specific tetramer staining of cognate transgenic antigen showed negative selection of thymocytes throughout the thymus even prior to positive selection [14]. But the high amounts of the cognate neo-self antigens may have overridden the specific requirements for negative selection, e.g., the need for costimulation. Negative selection might therefore take place in the cortex but only under extreme conditions that may not always be physiological.
Could physiological negative selection occur in the medulla? It is packed with bone marrow-derived dendritic cells (DCs) that are involved in negative selection. It is relatively permeable to blood-borne soluble proteins, and thus might be involved in the negative selection of thymic T cells specific for some peripheral self antigens released as a result of normal cell metabolism. TdT-catalyzed DNA nick-end labelling of thymus tissue sections showed that apoptotic cells in mice undergoing negative selection to endogenous superantigens were restricted to the medulla [15]. About two-thirds of medullary T cells are semi-mature HSAhi single positive cells that are tolerance susceptible [16]. Significantly, transgenic mice expressing MHC class II exclusively on cortical epithelial cells experienced a breakdown of self-tolerance. By contrast, no such breakdown occurred in chimeric mice expressing these molecules on all epithelial cells but not on hemopoietic cells [17]. The medulla may thus be the normal site for negative selection and one obvious question to be asked is whether all self-antigens are expressed in the medulla.
Surprisingly many proteins, that were believed to be tissue specific and sequestered, are now known either to be synthesized or to have their transcripts synthesized by thymic medullary cells [reviewed in [18]]. The level of expression is low, up to 200 transcripts per cell, and in some cases radioimmunoassay has shown protein expression. Among the tissue antigens synthesized by medullary cells are the following [19]:
- (a) pancreatic autoantigens – pro-insulin, insulin, glucagon, somatostatin, GAD7, GAD5, IA-2 [ICA 512], trypsin, chymotrypsin, amylase, carboxypeptidase A1, elastase;
- (b) thyroid autoantigens – thyroglobulin, thyroid peroxidase;
- (c) nervous system autoantigens – MBP (PLP), astrocyte gene encoding S100β, neuroendocrine peptides vasopressin & oxytocin;
- (d) retinal autoantigens – arrestin, interphotoreceptor retinoid-binding protein;
- (e) liver autoantigens – C-reactive protein, serum amyloid protein, c-Met oncoprotein;
- (f) trophoblast – HLA-G;
- (g) acetyl-choline receptor.
What cells in the medulla synthesize these antigens? A transgenic mouse model has shown that expression of an MHC antigen exclusively on thymic medullary epithelial cells induced specific tolerance to that antigen [20]. More recent work has indeed identified cells expressing transcripts as subsets of medullary epithelial cells (although some have shown some of the characters of blood-borne DCs [21]). Intrathymic expression is confined to a few cells in the medulla [21]. This implies that there occurs differentiation of thymic epithelium along different epithelial cell lineages displaying a mosaic of so-called ‘extra thymic’ self-proteins. There must therefore be a strikingly efficient, but not yet understood, mechanism for inducing self tolerance as positively selected thymocytes would have to extensively scan medullary epithelial cells. This may account for the 5–10-day length of time that the T cells remain in the medulla.
To summarize, the thymic medulla appears to be the primary site of negative selection. Many tissue specific antigens, previously thought to be sequestered, are indeed synthesized by medullary epithelial cells, under the control of genes such as the autoimmune regulator gene, AIRE, discussed in Section 11.2. In addition, since the medulla, packed as it is with bone marrow-derived DCs, is relatively permeable to soluble proteins, it can be expected that small quantities of tissue specific antigens, released as a result of normal cell metabolism, could reach it via the blood stream. Tolerance to a great majority of tissue specific antigens could thus be achieved by intrathymic deletion of at least high affinity T cells. Low affinity cells that escaped to the periphery should be harmless and may ignore their target, or may even be subjected to peripheral tolerance mechanisms as described below. Viewed in this light, the thymus is the major site responsible for the induction of self-tolerance by the deletion of high affinity self-reactive T cells. As a corollary, one would expect that defects in thymic selection may be the major cause of some autoimmune diseases as discussed in Section 11.
6 Site of encounter – extra-thymic tolerance
What does happen to those T cells that may not have encountered self-antigens in the thymus? These may not induce an immune response if they are sequestered in privileged sites away from the circulating routes of naı̈ve T cells, or exposed on non-MHC-expressing cells that therefore cannot load peptides derived from those antigens. It may also be the case if the auto-antigens are present in amounts too low to be detected by T cells, or if the avidity of the combined TCR and accessory molecules is not sufficiently high for T cells to contact the autoantigen-presenting cells effectively. Under these conditions, naı̈ve T cells will ignore these cells [22], but the resulting lack of T cell activation is not equivalent to tolerance induction since presentation of the autoantigens by professional antigen presenting cells (APCs) would immunize. This may occur, for example, when molecules are released from dying cells. Fail-safe mechanisms inducing post-thymic tolerance must therefore exist.
Antigen may be presented to T cells by several intracellular pathways. The exogenous and endogenous pathways are well-documented, exogenous antigen being taken up by APCs and presented to class II restricted CD4 T cells, whereas antigens synthesized within the cell are presented to class I restricted CD8 T cells. But CD8 T cells can also be activated via cross presentation (Fig. 1). This was first demonstrated by Bevan with minor H antigens [23]. The phenomenon of cross priming was later extended by my colleagues and me to show cross-tolerance [24]. Our studies have illustrated a mechanism by which peripheral tissue antigens can be captured by APCs and cross-presented to CD8 T cells to induce deletional tolerance [25–27]. For this purpose, we used the following model. The host was a transgenic C57BL mouse, ‘RIP-mOVA’, expressing the major peptide of the ovalbumin molecule, SIINFEKL, as a membrane bound molecule in the β cells of the pancreas (‘mOVA’). It was thymectomized, grafted with a thymus from a nontransgenic C57BL donor, heavily irradiated and injected intravenously with bone marrow from a C57BL donor expressing a class I restricted transgenic TCR specific for SIINFEKL (‘RAG-deficient OT-I mouse’) (Fig. 2). The thymus graft and lymph nodes draining the pancreas of these chimeric mice were subsequently examined by flow cytometry. As evident from these profiles (Fig. 3), there was no deletion of transgenic CD8 T cells in the thymus graft, presumably because SIINFEKL was not expressed there. But in the lymph nodes draining the pancreas, there was a loss of these CD8 T cells. Other work showed that the peptide was transferred from the β cells to the draining lymph node, presumably via APCs, which cross-presented it to incoming CD8 T cells that became activated and eventually underwent activation-induced cell death. This process was dependent on bim expression by the responding T cells [28] and was referred to as cross-tolerance [24].
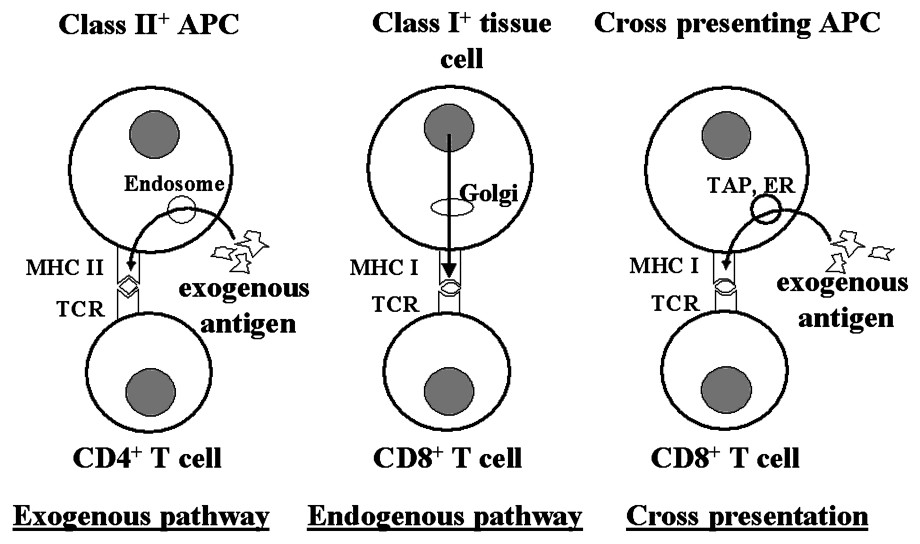
Pathways of antigen presentation to T cells. CD4+ T cells generally recognize antigenic determinants in association with MHC class II molecules on the surface of APCs that have taken up the antigen exogenously and processed it intracellularly (exogenous pathway). CD8+ T cells recognize antigen synthesized within tissue cells or APCs and presented in association with class I molecules (endogenous pathway). They can also recognize antigen taken up by the APCs exogenously and processed intracellularly. Phagosomes fuse with endoplasmic reticulum (ER) derived vesicles. The resulting phagosome-ER hybrid compartment contains newly synthesized MHC class I molecule and components required for peptide loading such as TAP (transporter associated with antigen processing). Phagocytosed antigen is then transported to the cytosol close to the phagosome, degraded by proteasomes and the resulting peptides transported back into the phagosome via the TAP complex for loading onto class I molecules.

Experimental set-up used to determine the fate of T cells reactive to an extra-thymic self-antigen; see text.

Thymuses from nontransgenic C57BL mice were grafted into thymectomized transgenic RIP-mOVA mice and nontransgenic littermate controls. The recipients were then heavily irradiated and protected with bone marrow from TCR transgenic OT-I mice. Flow cytometry of the thymus grafts 4 months after implantation showed that OT-I cells (anti-SIINFEKL CD8+CD4−Vα2− T cells) were able to mature in both types of recipients indicating that the thymus grafts were not expressing the target transgene autoantigen (mOVA). Conversely, flow cytometry analyses of the lymph nodes draining the pancreas of the RIP-mOVA recipients showed a significant reduction (3.2%) in the proportion of OT-I cells relative to that seen in the nontransgenic controls (20.3%).
We cannot prove whether the events observed in our transgenic mice do occur also in an unperturbed physiological situation. What we have found might, however, well mimic the following scenario. Self-reactive T cells that have escaped thymus negative selection enter lymph nodes draining a healthy tissue that releases a certain amount of self-antigen at a certain rate. If this amount and rate are below a particular threshold level, the naı̈ve T cells, which normally do not circulate into nonlymphoid tissues [29], will not be activated and thus will ignore their target. If the amount and rate are above that level, the T cells will be activated as soon as they enter the draining lymph nodes and will be subjected to activation-induced cell death. No autoimmune damage will thus take place.
The failure to induce T cell apoptosis is in fact an important factor that accounts for the loss of self tolerance and the development of autoimmunity in animals and individuals with a mutation in the genes coding for death receptors [30]. In patients with autoimmune lymphoproliferative syndrome [ALPS], Fas mutations, usually in the Fas death domain, cause defective Fas-mediated lymphocyte apoptosis. A similar syndrome, ALPS II, occurs in patients that have a mutation in caspase 10, which is a death protease that must be activated for apoptosis to occur [31]. Some patients with caspase 10 mutations significantly had a marked accumulation of DCs that, in a normal immune response, would be rapidly eliminated [31]. The failure to eliminate T cells and DCs in ALPS patients must therefore have contributed to the autoimmune pathogenesis.
7 Type of cells presenting epitopes
Only cells bearing MHC molecules can present antigen to T cells since these are self-MHC-restricted. Intra-thymic selection by APCs has already been discussed above and a major question that has to be resolved is whether the same APC in the periphery can induce both tolerance and immunity, or whether distinct cell types are involved in either response. Our initial studies showed that cross-tolerance involved antigen presentation by a bone marrow-derived cell [25] and that this cell expressed CD11c [32], suggesting it was of DC origin. DCs can be subdivided into at least six subsets [33], but it is a single subset, defined by expression of CD8α and CD205, that appears responsible for cross-tolerance [34]. These DCs are referred to as CD8α DCs. In other models, a second subset, which is CD8α-negative and expresses CD11b, induced CD4 T cell tolerance to self antigens [35], but only the CD8α DCs appear to induce CD8 T cell cross-tolerance. CD8α DCs have been reported to be the main subsets responsible for cross-presenting foreign cell-associated antigens [36], suggesting that this subset may be responsible for both cross-priming and cross-tolerance. Interestingly, by most routes of infection, CD8α DCs have also been shown to play a dominant role in the generation of immunity to several viruses, including herpes simplex virus, influenza virus and vaccinia virus [37,38] confirming their role in immunity and suggesting that cross-priming may be important for CTL responses to viral antigens. Together, these studies highlight the central role for CD8α DCs in the induction of both immunity and tolerance to cellular antigens, particularly with respect to CD8 T cells.
8 Co-stimulatory activities
In the early 1970s, Bretscher and Cohn [39] claimed that two signals were required for lymphocytes to respond. Signal 1 alone would switch off the cells and tolerize, whereas signals 1 and 2 would lead to an immune response. Lafferty and coworkers [40] extended this claim and postulated that the first signal was antigen specific and the second was a costimulator signal delivered by an APC. The subsequent discovery of co-stimulator molecules on APCs and of the powerful immunogenic properties of DCs has vindicated this idea. But does signal 1 alone, under physiological conditions and in vivo, exert any effect? Excluding the neonatal period, it is well established that naı̈ve T cells fail to enter nonlymphoid tissues [29], i.e., the very tissues whose cells can produce signal 1 alone, as they do not possess co-stimulatory activity. One wonders, therefore, how naı̈ve T cells could ever be anergized in vivo, since they enter such tissues only after being activated in the draining lymph nodes that are populated by APCs that provide both signals 1 and 2.
9 The danger concept
Matzinger [41] has claimed that the immune system discriminates between harmless and dangerous entities rather than between self and nonself. Several examples are difficult to explain according to this idea. One such situation pertains to graft rejection. As nonplacental allografts do not normally occur in nature, the immune response could not have evolved to regard these as dangerous and yet they provoke a powerful immune response [42]. The danger resulting from the trauma of surgically implanting the graft cannot account for the rejection, since syngeneic grafts do not provoke a response and are accepted. Yet the inflammation associated with the surgical procedures must stimulate the many DCs expressing costimulatory molecules and MHC molecules loaded with peptides. Of course, in both these examples the clonally individuated T cells, with high affinity receptors for self-MHC and self-peptides, have been deleted in the thymus [11]. Hence at least in the thymus, discrimination does occur between self and nonself rather than between harmless and harmful entities. But since, in these cases, there are no self-reactive T cells circulating, does the danger concept apply only to situations where there are specific T cells circulating in the periphery? In the classical and well-controlled experiments of Billingham, Brent and Medawar, longstanding [up to 144 days] allografts in healthy immunologically tolerant mice were destroyed following an intravenous injection of purified naı̈ve lymphocytes from normal unsensitized donors of the same strain as the tolerant host [43]. Where does danger exert its effect in this experiment? It would really be stretching credulity to argue that an intravenous injection mimics a danger signal, particularly as an intravenous injection of bone marrow syngeneic with the donor of the tolerant host produced no effect and, more importantly, injecting lymph node cells immune to the tolerated graft, but foreign to the host, did not lead to skin graft rejection [43].
10 T cell-dependent suppression
Evidence has steadily been accumulating in favor of some type of T cell-dependent suppression of potentially autoaggressive T cells [44–46]. Suppressor T cells, first suggested in 1974 by Gershon [47], have had a long and tempestuous history. Nevertheless, one way in which such cells can suppress immune responses is by the inhibitory effects of cytokines, such as TGF-β released by T cells after some types of antigenic stimulation [48]. Furthermore, the evidence first obtained by Mosmann and Coffman [49] for two types of helper T cells, Th1 and Th2 with distinct antagonistic lymphokine profiles, indicates that T cell dependent immunoregulation of immune responses is a reality that requires further exploration at both the cellular and the molecular levels. More recent work has shown an important role for CD4+CD25+ T cells in the active suppression of autoimmunity. The forkhead transcription factor Foxp3, specifically expressed in these cells, is essential for their development [50]. As CD25+ T cells usually express high levels of CD5, which represents T cells with high avidity for self-peptide-MHC, their regulatory function may reflect their inordinate ability to access antigen on APCs and growth factors such as IL-2 and IL-7 [51]. This could limit expansion of any autoreactive T cells that may be present or introduced.
11 Defects in thymic selection
If, as stated above, the thymus is the major site responsible for the induction of self-tolerance by the deletion of high affinity self-reactive T cells, one would expect that defects in thymic selection may be a crucial factor in the pathogenesis of some autoimmune diseases. This is indeed the case as evidenced in both experimentally induced and naturally occurring defects.
11.1 Experimentally induced defects
Transgenic mice expressing MHC class II exclusively on cortical epithelial cells experienced a breakdown of self-tolerance as evidenced by the circulation of a large number of self-reactive lymphocytes (up to 5% of the total pool). This presumably resulted from unopposed positive selection [17].
H2-M is a molecule that loads a diverse peptide repertoire onto MHC class II molecules. In the absence of H2-M, the class II molecules are occupied only by the class II associated invariant chain peptide, CLIP. Yet, this single MHC peptide complex was able to positively select a substantially diverse T cell repertoire, but the proportion of CD4 T cells with autoproliferative response to wild type APCs was very high, at 70–80%. Here again we see defective negative selection in mice with efficient positive selection [52]. Likewise in mice expressing a transgenic MHC linked to a single peptide, the failure of the MHC molecule to bind a vast array of normal peptides precluded self-tolerance induction to these peptides [53].
11.2 Naturally occurring defects
BB rats show no thymic abnormalities until 4 weeks of age, when regions of the cortex and medulla become depleted of epithelium, and the rats then develop autoimmune conditions such as insulitis and thyroiditis [54].
NOD mice, which spontaneously develop autoimmune diabetes, have a number of thymic abnormalities. The medulla is heavily disorganized with a scattering of medullary epithelial cells in the cortex and a reduction in the size of the medulla [55]. The NOD MHC class II molecule H2-Ag7 has an unstable ‘SDS phenotype’, which is believed to be associated with a significant decrease in the density of self MHC-peptide complexes on thymic APCs [56]. To attain selection thresholds, there must therefore be a correlative increase in the mean population TCR affinity for such complexes, and this in turn results in the escape from negative selection of T cells which would otherwise be deleted. It thus predisposes the repertoire to peripheral autoimmunity. But since the same MHC defect exists in the periphery, other factors such as inflammation must play a role in determining the autoimmune response, and indeed MHC alone is known not to be sufficient for autoimmune sequelae. It is also possible that the extra-thymic engagement of T cells bearing high affinity autoreactive TCRs might bias the repertoire towards a destructive Th1 phenotype, which is in fact the predominant phenotype of peripheral NOD T cells.
Patients with autoimmune polyendocrinopathy syndrome have a mutation causing a loss of function of the AIRE gene, a transcription factor expressed mainly in thymic medullary epithelial cells [57]. Significantly, mice lacking the AIRE gene show autoimmune symptoms and a substantial reduction of tissue specific antigens in thymic stromal cells [58]. To determine whether these mice were deficient in central thymic tolerance to self-antigens, the following experiment was performed [59]. Transgenic mice expressing hen egg lysozyme [HEL] in the β cells of the pancreas and in the thymus were crossed to HEL-specific TCR transgenic mice. The double transgenic mice had reduced numbers of HEL-specific CD4+ T cells in the thymus and spleen as compared to those in single TCR transgenic mice, the reduction being pronounced for those T cells expressing a high density of HEL-specific TCR. After crossing these mice to AIRE−/− mice, the numbers of TCRhi HEL-specific CD4+ T cells expressing autoreactive CD4+ T cells were markedly increased, thus establishing a role of AIRE in the central deletion of forbidden clones.
11.3 Genetic studies
Further evidence supporting the idea that defects in thymic selection may be a major factor in the pathogenesis of some autoimmune diseases comes from genetic studies. In rat and mouse models of autoimmune uveoretinitis, there is an inverse correlation between thymic expression of the retinal specific antigens arrestin and interphotoreceptor retinoid-binding protein and susceptibility to the disease that is induced by those antigens [60]. Likewise in insulin promoter transgenic mice [61,62], susceptibility to autoimmune diabetes inversely correlates with levels of thymic expression of the transgene but not with pancreatic levels. In humans, there is an inverse correlation between insulin mRNA levels in thymus and susceptibility to insulin-dependent diabetes [63,64]. IDDM2 is one of the polymorphic genetic loci that correlate with susceptibility to IDDM. There are two main polymorphic allele classes distinguishable by the length of the mini satellite element. The short type (class I) correlates with predisposition to develop IDDM, the long version [class III] segregates as a resistant allele. Individuals with class III alleles show a 2–3-fold higher thymic insulin mRNA levels than those with class I alleles, and this correlates with disease resistance. No such correlation can be made between these alleles and pancreatic mRNA levels.
12 Conclusions
- 1. The thymus is the prime organ responsible for self–nonself discrimination and the acquisition of self-tolerance.
- 2. Negative selection of high affinity self-reactive T cells occurs predominantly in the medulla and involves epithelial cells and blood-borne dendritic cells.
- 3. Many tissue antigens, previously thought to be sequestered, are now known to be synthesized by medullary epithelial cells, under the control of genes such as AIRE.
- 4. Tissue antigens, released as a result of normal cell metabolism, may reach the relatively permeable medulla via the blood and be taken up by dendritic cells involved in negative selection.
- 5. Defects in negative selection are factors in the pathogenesis of autoimmune diseases.
- 6. There is an inverse correlation between gene expression of some ‘tissue-specific’ antigens in the thymus and susceptibility to autoimmune disease.
- 7. Self-reactive T cells that have left the thymus, either because of low affinity for the target autoantigen or because the latter was not represented intrathymically, may ignore their target or be subjected to peripheral tolerance mechanisms such as activation-induced cell death and suppression.