1 Introduction and background
Industrial utilisation of plant fibres has always been a major item on this group's research agenda, since the very beginning of its life [1]. Since their conception, these R&D efforts have been based on the idea of integrated utilisation of plant biomass (Fig. 1), which is at the heart of the group's mandate. They have gradually evolved around a small number of largely interrelated ideas: (a) physicochemical fractionation of main macromolecular components (cellulose, hemicellulose and lignin), while preserving (or upgrading) fibre qualities; (b) development of sulphur- and chlorine-free delignification processes adapted to the characteristics of each plant, mainly based on the oxygen and organosolv concepts; and (c) formulation of analytical protocols and, where necessary, novel ‘intelligent’ ones with the aim to support industrial applications with relevant information at the molecular level.

The idea of integrated utilisation of plant biomass.
In this context, a number of wood fibres and, especially, many annual fibrous feedstocks have been investigated, including agricultural and agro-industrial residues – e.g., wheat, barley, and rice straws, corn stover and cobs, cotton, tobacco, grape and rape stalks – as well as potential dedicated industrial/fibrous plants, e.g., sorghum (fibre and ‘sweet’ varieties), miscanthus, arundo donax, okra. A few selected significant achievements of this research are presented in the following.
2 Results and discussion
Historically, the cornerstone of this group's achievements in this field has been the development of a prehydrolysis – chemical pulping process, especially for annual fibres, making possible the co-production of printing-paper grade straw pulp along with fermentable monosaccharides appropriate as substrate for biotechnological applications [2]. This initial scheme has been the source of several novel R&D lines focusing on various levels of the central concept, such as, e.g., the development of a prehydrolysis–organosolv scheme for the optimal fractionation of the three major fibre cell wall components [3].
Building upon the data obtained from this work, a non-empirical mathematical model that adequately describes the kinetics of the fractionation process was designed and developed for process optimisation and design purposes [4]. This model is based on the well-established idea of a sequence of reaction steps obeying (pseudo) first-order kinetics. We have applied the methodological approach based on the distinction of fractionation stages and reaction phases (heterogeneous system), and the use of modified Arrhenius-type kinetic equations (Fig. 2 shows an application to corn cobs). A very important concept of this model is the ratio of the resistant fraction to the easily hydrolysed one of each macromolecular cell-wall component, as uronic acid and other types of linkages appeared to be quite stable under the process conditions used. In such reaction systems, the heat and mass transfer effects were found to be statistically insignificant. The results show a high statistical correlation of the proposed model to the experimental data, with a correlation coefficient usually higher than 0.85; another important feature of this model is the potential use of the same kinetic formulas to quantitatively describe the fractionation of any type of plant fibre feedstock.

A non-empirical mathematical model that adequately describes the kinetics of the fractionation process.
In another line of its research, this group has worked on the development of a new analytical concept (AFFLUENCE) for fibre and fibrous products characterisation, combining fluorescence spectroscopy [5] with chemometrics. This concept consists in the multivariate analysis of fluorescence spectral and chemical data of lignocellulosic fibrous materials and their production and treatment processes [6,7]. In particular, fluorescence spectral data are correlated with physical and chemical properties through partial least squares models (PLS), whereas principal-component analysis (PCA) is employed as a multivariate method aiming at determining the main variation in a multidimensional data set by creating new linear combinations of the raw data. Three examples from the application of this analytical approach at various levels are presented here.
Fig. 3 shows the application of the AFFLUENCE approach for the study of annual fibres (in this case wheat straw; also sweet and fibre sorghum stalks were used) pulped with aqueous ethanol solution under acid- or alkali-catalysed conditions, and bleached with hydrogen peroxide aqueous solutions in an alkali environment during various processing times [8]. The results from the study of these examples have indicated that the fluorescence emission spectra of solid paper pulps and their black liquors could provide vital information about the origin of the pulp sample and the kind of chemical treatment applied. The latter was also concluded from the second example, i.e., the application of AFFLUENCE on residual Kraft lignin bleaching by various methods (Fig. 4), that is, one can distinguish the bleaching chemistry employed from the fluorescence spectra of the bleached samples [9,10]. So these two examples show the utilization of the AFFLUENCE approach to optimise a pulping, bleaching or fractionation process as the on-line use could give important information and data for the control of critical control points of the process.
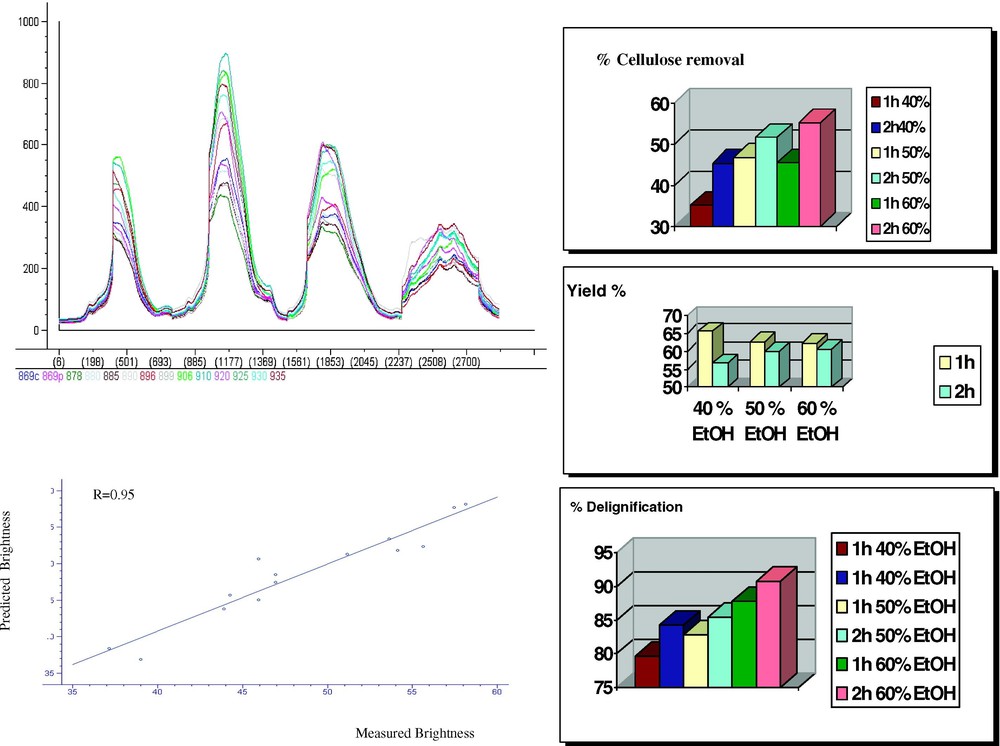
PLS model of the predicted versus measured values for the brightness (B/R475) of the bleached and alkali-catalysed organosolv wheat straw samples. R=0.95 for the second PC.


PCA score plot of fluorescence spectra of RKL samples subjected to treatments with varying charges of chlorine dioxide (D), dimethyldioxirane (AO), alkaline hydrogen peroxide (H), and oxygen (O).
The last example concerns the application of AFFLUENCE to the study of old paper. Our research findings include the existence of good correlation between the fluorescence data and the chemical and physical data (Kappa No., brightness, cellulose, hemicellulose and lignin contents) of the pulps through PLS models. We also studied the evaluation of phenomena related to paper ageing, using samples of naturally ageing paper from various Greek archives (see the paper age-spectral data correlation in Fig. 5). The results obtained [11] (unpublished data) have verified the power of the use of fluorescence spectrometry in conjunction to chemometrics to provide valuable information on ageing and storing of these paper materials, including the use of multivariate models to derive useful chemical information, e.g., on paper alkalinity and ash.

PLS model of the predicted versus measured values for the age of the samples.
3 A strategic view
Fig. 6 summarises the strategic view of this group's plant fibre research. In order to optimise technical action at the appropriate molecular and process levels, one needs three types of strategic tools, i.e., (i) novel technical solutions, e.g., appropriate fractionation, pulping or bleaching techniques; (ii) adequate process models permitting a satisfactory quantitative representation of the technical action of concern; and (iii) intelligent characterization methods for the imaging and integrated assessment of feedstocks, processes and products.
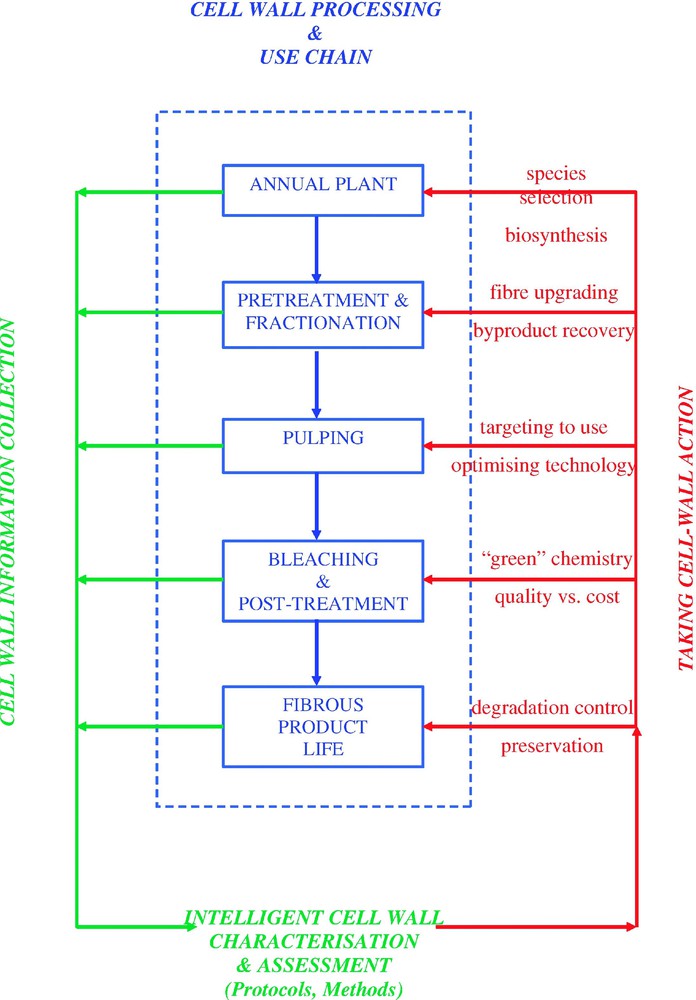
A strategic view at the annual plant fibre system, based on structural/functional information at the molecular level.
☆ This article is dedicated to Prof. Bernard Monties on the occasion of his retirement.