1 Introduction
Growth diminution has commonly been observed in plants subjected to cadmium- and copper-ion-poisoning situations. These toxic trace pollutants can induce many alterations in plant cells [1–4], but it is difficult to draw a general mechanism about the physiology of stress, since metal toxicity results from complex interaction of metal ions with several metabolic pathways. However, one of the underlying causes of tissues injury following exposure of plants to Cd and Cu is the increased accumulation of reactive oxygen species mediated-oxidative stress [4–8]. The deleterious effects resulting from cellular oxidative state may be alleviated by small reduced metabolites, such as ascorbate (ASC), glutathione (GSH), α-tocopherol, carotenoids, flavonoids, and uric acid, and oxygen radical detoxifying enzymes, such as superoxide dismutase (SOD), catalase (CAT), and enzymes of ASC-GSH cycle; ascorbate peroxidase (APX), monodehydroascorbate reductase (MDHAR), dehydroascorbate reductase (DHAR), and glutathione reductase (GR). The effects of Cd and Cu on the activity of these enzymes and their involvement in the defence of plant tissues against metal-induced damage remain controversial [2,4–11]. It has been suggested that the tolerance to metal toxicity is more dependent on the availability of reduced cell metabolites, such as NAD(P)H, than on antioxidant enzymes capacity of plant tissues [6,12]. For example, the enzymatic functioning of the ASC-GSH pathway, which involves successive oxidation and re-reduction of ASC, GSH and NAD(P)H [13], depends on the reducing power that can be supplied directly at the expense of photosynthate or supplied via secondary NAD(P)H-recycling dehydrogenase activities, such as glucose-6-phosphate dehydrogenase, malate dehydrogenase (MDH), 6-phosphogluconate dehydrogenase, isocitrate dehydrogenase [14,15].
On another hand, deleterious effects of heavy metal stress in plants may be coupled to other physiological processes via the stimulation of some enzymatic activities that limit cell growth and, consequently, accelerate tissue ageing [4,16–19]. At cellular level, increase of IAA oxidase activity, acting on auxin catabolism by oxidative decarboxylation [20], may control the endogenous rate of this growth phytohormone [21] and, thereby, reduce cell growth. Cell-wall stiffening can also control cell growth, through reduction of plasticity. This may be done by the activity of ionically or covalently bound cell-wall peroxidases involved in the polymerisation of phenolic monomers of suberin [22], extensin metabolism [21], and lignification. This latter is catalysed by the oxidative polymerisation of monolignols (sinapyl, coumaryl and coniferyl alcohols) in the presence of H2O2, which is produced by the activity of NADH oxidase, which itself receives NADH from MDH activity [23,24].
In this paper, we studied the effects of Cd and Cu on the malondialdehyde (MDA) content (indicator of oxidative stress) and the activities of (1) guaiacol peroxidase (GPX, stress marker), (2) antioxidant enzymes (SOD, CAT, APX, MDHAR, DHAR and GR), MDH and (3) some enzymes involved in cell growth limitation (IAA oxidase and lignifying peroxidases; coniferyl alcohol peroxidase (CAPX) and NADH oxidase) in pea leaves. The contribution of cell fractions to enzymatic responses to heavy-metal stress was also emphasized.
2 Materials and methods
2.1 Plant material
Seeds of pea (Pisum sativum L. cv. ‘douce province’) were disinfected with 10% H2O2 for 20 min and then washed thoroughly with distilled water and germinated between wet paper towels at 25 °C in the dark for three days. Seedlings were transferred to plastic beakers (10 plants per beaker) filled with 6 l of the nutrient solution (pH 5.7) containing: Ca(NO3)2 (2.5 mM), KNO3 (2 mM), KH2PO4 (1 mM), MgSO4 (1 mM), Fe–K–EDTA (50 μM), H3BO4 (30 μM), MnSO4 (10 μM), ZnSO4 (1 μM), CuSO4 (1 μM) and (NH4)6Mo7O24 (0.03 μM). Plants were grown in a growth chamber (16 h light–8 h dark) under mercury lamps, providing a light intensity of 150 μmol m−2 s−1, day/night temperature of 25/20 °C and 65 relative humidity. After 12 days, treatments were performed, for four days, by adding 0 (control), 20 and 100 μM of Cd(NO3)2 or CuSO4 to the above-mentioned solution. At harvest, leaves were weighed and stored in liquid nitrogen until use.
2.2 Estimation of lipid peroxides
Lipid peroxidation was determined by measuring the concentration of MDA, major thiobarbituric acid-reactive material, as described previously [25].
2.3 Extraction of proteins
Extraction of ionically and covalently bound cell wall proteins was performed as described by De Jaegher et al. [26]. Soluble and microsomal proteins were extracted according to the method of Basu et al. [27].
2.4 Enzyme assays
The enzyme activities were determined according to following methods: GPX [28], total SOD [29], CAT [30], APX [31], MDHAR and DHAR [32], GR [13], IAA oxidase [33], CAPX [34], NADH oxidase [35] and MDH [36]. All enzyme activities were expressed per gram fresh weight. One unit of enzyme was defined as the amount necessary to decompose 1 μmol of substrate per minute at 25 °C in the case of CAT, APX, MDHAR, GR, CAPX, and NADH oxidase. For GPX, DHAR, and MDH activities, one unit was defined as amount of enzyme producing 1 μmol of, respectively, tetraguaiacol, ASC and NADH/min at 25 °C, while IAA oxidase activity was estimated as at 25 °C. To calculate SOD activity: , where V and v are the slope of the change in absorbance in the absence and in the presence of enzyme extract, respectively.
2.5 Statistics
The experiments were repeated at least twice. The data presented here are from representative experiments. The mean values ±SD are reported in Table 1 and Figs. 1–4. The significance of differences was determined at the 0.05 level of probability.
FW and MDA content of leaves of 16-day-old pea plants grown in control nutrient solution and supplemented with cadmium or copper for 4 days
Treatment | μM | FW ± SD () | MDA ± SD () | ||
(mg) | (%) | (nmol g−1 (FW)) | (%) | ||
Control | – | 1123 ± 49a | 100 | 10.1±1.4d | 100 |
Cadmium | 20 | 905 ± 41b | 81 | 15.5±0.9e | 153 |
100 | 642 ± 131c | 57 | 24.4±1.5f | 242 | |
Copper | 20 | 1093 ± 85a | 97 | 15.2±1.1e | 151 |
100 | 775 ± 149bc | 69 | 21.4±2.0f | 212 |
a Values followed by a common letter are not different at the level of significance.
b Values followed by a common letter are not different at the level of significance.
c Values followed by a common letter are not different at the level of significance.
d Values followed by a common letter are not different at the level of significance.
e Values followed by a common letter are not different at the level of significance.
f Values followed by a common letter are not different at the level of significance.
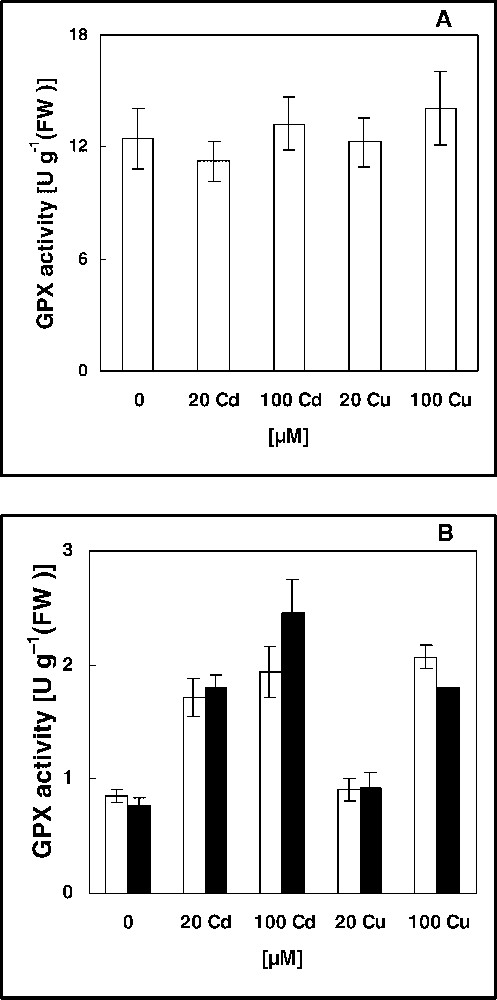
Activity of GPX in soluble (A), ionically (white), and covalently (black) bound cell-wall (B) fractions of leaves of 16-day-old pea plants grown in control nutrient solution and supplemented with cadmium or copper for four days. The values given are the averages of four experiments. Error bars (SD) indicated when large enough to be shown.
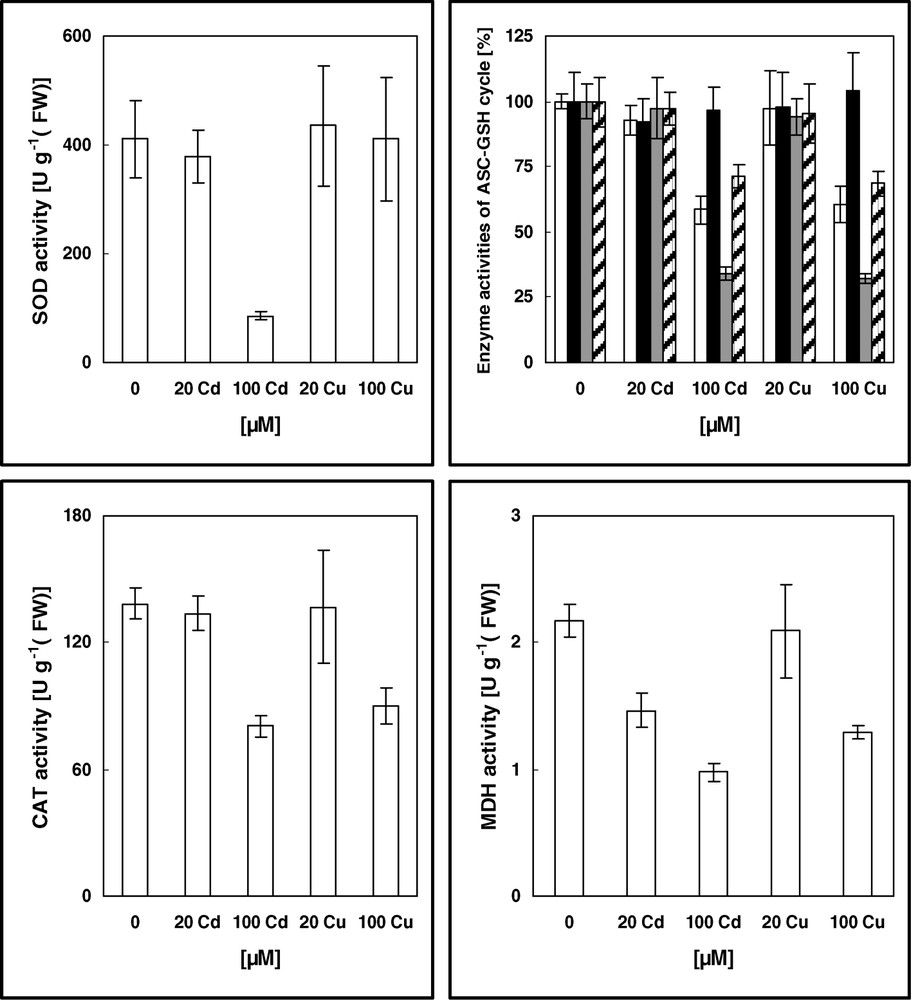
Activities of SOD, CAT, ASC-GSH cycle enzymes and MDH in leaves of 16-day-old pea plants grown in control nutrient solution and supplemented with cadmium or copper for four days. The values given are the averages of four experiments. Error bars (SD) indicated when large enough to be shown. The control activities of ASC-GSH cycle enzymes [U g−1 (FW)] are: 2.01 (APX; white), 0.64 (MDHAR; black), 0.47 (DHAR; grey), and 0.89 (GR; hatched).
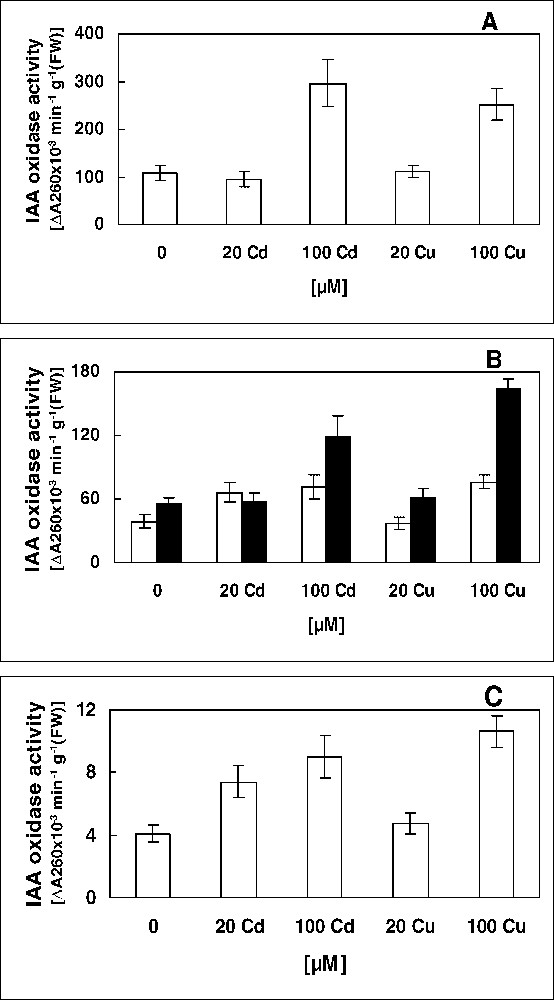
Activity of IAA oxidase in soluble (A), ionically (white) and covalently (black) bound cell wall (B) and microsomal membrane (C) fractions of leaves of 16-day-old pea plants grown in control nutrient solution and supplemented with cadmium or copper for four days. The values given are the averages of four experiments. Error bars (SD) indicated when large enough to be shown.
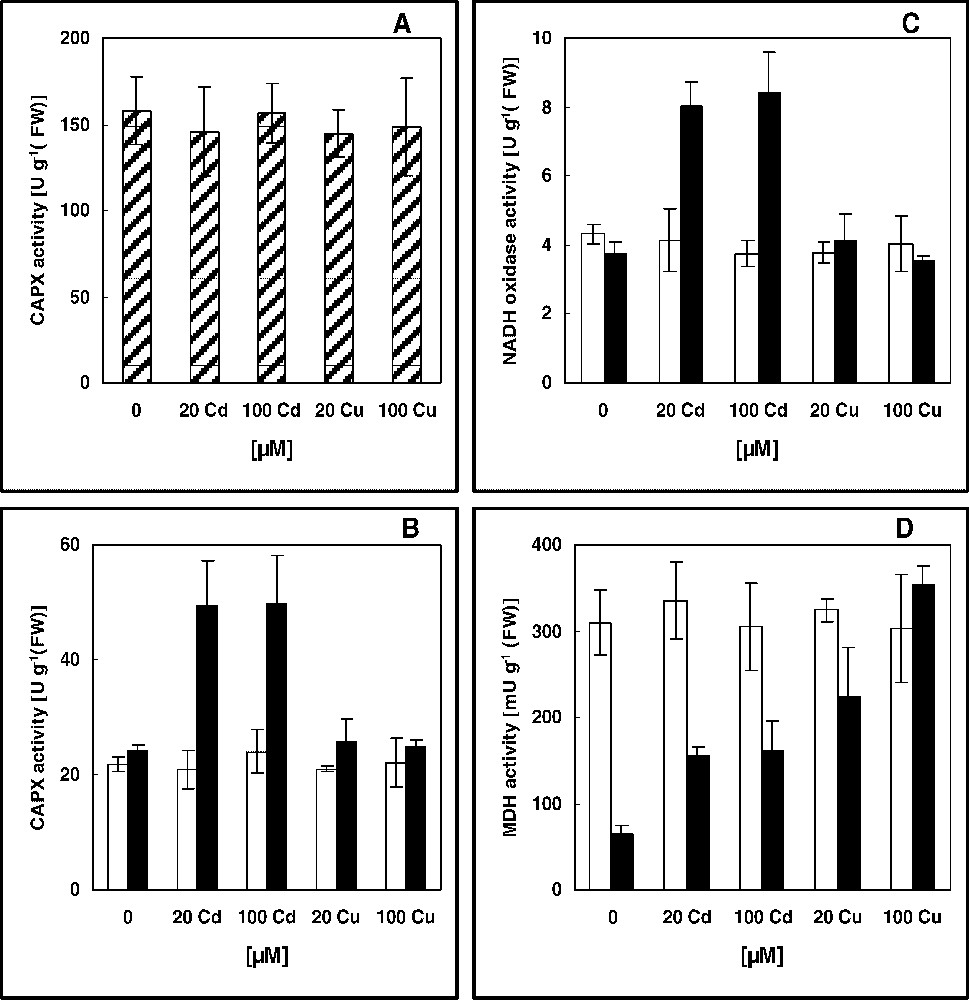
Activities of CAPX, NADH oxidase and MDH in leaves of 16-day-old pea plants grown in control nutrient solution and supplemented with cadmium or copper for 4 days: (A) soluble; (B)–(D) ionically (white) and covalently (black) bound cell wall fractions. The values given are the averages of four experiments. Error bars (SD) indicated when large enough to be shown.
3 Results and discussion
Table 1 shows the effects of metal treatments on fresh weight and MDA content of pea leaves. 100 μM of Cd and Cu caused strong accumulation of lipoperoxidation products; increase of 142 and 112% from control, and pronounced reduction of growth; decrease of 43 and 31% as compared to control, respectively. To estimate the oxidative stress, some workers have used carbonyl groups and 4-hydroxynonenal, respectively, as protein oxidation and lipid peroxidation markers [7,37], but the tissue MDA content had been widely used as an indicator of lipid peroxidation and, thereby, of oxidative damage in heavy- metal-exposed plants [5–7,10]. However, equivalent increases of MDA content were induced after exposure to 20 μM Cd and Cu (53 and 51% from control, respectively; Table 1), while the growth was suppressed by only Cd exposure (decrease of 19% from control; Table 1). In previous work, we have suggested that MDA is not an efficient indicator of overall cellular oxidative stress [9].
On the other hand, unspecific peroxidases, i.e. enzymes oxidizing phenolic substrates such as guaiacol [23] are considered to be heavy-metal-stress-related enzymes [38] and can be used as stress marker [39]. However, the changes of peroxidase activity and isoenzyme patterns have been often followed from the only readily extractable soluble proteins [38,40,41]. Data presented here (Fig. 1) indicate that exposure to Cd and Cu resulted in no significant modification in the GPX activity in the soluble fraction of pea leaves (Fig. 1A), which is in contrast to the inhibitory effect of metals on growth (Table 1). The GPX activity was markedly increased (stimulation range of 102 to 219% from controls) in ionically and covalently bound cell wall fractions in response to growth suppressing treatments (the two doses of Cd and 100 μM of Cu), but not after exposure to 20 μM of Cu (Fig. 1B). Thus, if unspecific peroxidases assay is confined to the soluble proteins extract, it would not be considered as an efficient stress indicator, but when extended to cell-wall fractions, at least, this parameter might be a suitable stress marker.
The effects of metal exposure on antioxidant capacities, total SOD, CAT, enzymes of ASC–GSH cycle and on NAD(P)H-recycling MDH are presented in Fig. 2. 20 μM of metal intoxication not significantly altered the control level of all enzyme activities, except for MDH (Cd inhibition of 32% from control). Exposure to 100 μM of metal suppressed the antioxidant capacities, except for SOD in Cu-treated plants and MDHAR. Equivalent diminutions from controls were approximately recorded for Cd and Cu, respectively; CAT (41%, 35%), APX (41%, 39%), DHAR (66%, 67%), GR (28%, 31%), and MDH (54%, 41%) (Fig. 2). Fluctuations in antioxidant enzymes in response to Cd and Cu have frequently been found [4]. However, the ability of plants to increase antioxidant protection to combat negative consequences of heavy-metal-induced oxidative injury appears to be limited. Many studies showed that intoxication by elevated concentrations of Cd and Cu resulted in a decreased antioxidant capacity, and several possible explanations had been suggested [4,7,8,10,19]. Particularly, the accumulation of H2O2 seems to be a crucial event coupled with growth reduction [8]. The latter authors found that exposure to H2O2 had effects on antioxidant systems and on growth similar to those observed after exposure to Cd. Thus, diminution in antioxidant activities (Fig. 2) might, at least in part, be responsible for the growth reduction of leaves after exposition of pea to the most-damaging concentration (100 μM Cd and Cu; Table 1). Similar results had been previously found in pea and other species [6–8,19]. However, when added at 20 μM, the difference between Cd and Cu, regarding the growth state of leaves (diminution by Cd, but not by Cu; Table 1), would not be only associated to oxidative metabolism, but probably due to other effects.
Effects of metal treatments on some enzyme activities implicated in growth limitation are presented, with respect to cell fractions; IAA oxidase (Fig. 3) and lignifying peroxidases (Fig. 4). When applied at 100 μM, Cd and Cu approximately caused the same increase in IAA oxidase activity in all cell fractions of pea leaves (Fig. 3). However, no increase in this activity was seen in plants treated with 20 μM Cu, whereas 20 μM of Cd stimulated it; 69 and 80% from controls, respectively, in ionically bound cell wall and microsomal membrane fractions (Fig. 3). The activity of peroxidases involved in lignification was assayed here by hydrogen peroxide-dependent oxidation of coniferyl alcohol; a lignin monomer [34]. No significant modification was imposed by Cd and Cu in the soluble fraction, although the control level of CAPX activity in this extract was about 7-fold higher than in the cell wall (Fig. 4A and B). In this latter, H2O2-generating NADH oxidase and NAD-MDH activities were also measured. Ionically bound CAPX capacity in leaves of metals-stressed plants was around the control value, while exposure to Cd increased the enzyme activity in the covalently bound cell-wall fraction (Fig. 4B). A stimulatory effect of Cd was also obtained for NADH oxidase and MDH activities (Fig. 4 C and D). Thus, it seems that (1) the successive enzyme activities involved in lignification pathway; NADH-producing MDH, H2O2-generating NADH oxidase and CAPX [23,24] were stimulated by Cd treatments in covalently bound cell-wall fraction of pea leaves, and (2) cadmium stress acted in a dose-independent manner, since the two concentrations induced the same increases in activities of CAPX; 105 and 106%, NADH oxidase; 116 and 127%, and of MDH; 140 and 150%, respectively, for 20 and 100 μM of Cd (Fig. 4). However, in spite of the fact that MDH activity was strongly elevated by copper treatments in covalently bound cell wall fraction (Fig. 4D), it appears that Cu did not increase lignification, since the final stage of this process, i.e. the directly lignifying CAPX activity, was unchanged by Cu stress (Fig. 4).
Increase in activity of IAA oxidase and reduction in cell growth associated to auxin catabolism have been observed in other metal-stressed plants [16]. An accumulation of insoluble phenols, as lignin, in secondary cell wall have been reported in plants exposed to heavy metal and could be associated to an increase in activity of lignifying peroxidases [17–19]. Lignification decreases the cell-wall plasticity and, therefore, reduces the cell growth [4,19]. It seems that the reduction in leaves growth in plants stressed with 20 μM of Cd (Table 1) might be associated to an increase in the activity of IAA oxidase (Fig. 3) and of enzymes implicated in lignification (Fig. 4). No increase in activities of these growth-limiting enzymes was recorded after a treatment with 20 μM of Cu (Figs. 3 and 4). For the most deleterious treatments (100 μM; Table 1), the growth reduction could also be related to an increase in auxin degradation (Fig. 3) and in lignification (Fig. 4), in concert to a diminution in antioxidant systems (Fig. 2). It appears that the auxin degradation would be a resultant contribution of the enhanced IAA oxidase activity from all cell fractions, while the covalently bound cell-wall peroxidases seem to be the only contributor to the stimulation of lignification pathway. All these observations taken together point to a possible induction of leaf ageing after exposure to Cd and Cu.
Concerning the mechanism of metal-increased lignification, it has been realized that a loss in antioxidant capacities results in an intrinsic accumulation of H2O2, which would then act as a signalling molecule triggering secondary reactions: mechanical strengthening of cell walls including lignification [4,19]. However, two evidences could be in contrast to this scheme in pea leaves: (i) when added at the same concentration, Cd and Cu approximately provoked oxidative stress, as evidenced by the MDA content (Table 1) and the antioxidant capacities (Fig. 2), whereas only Cd increased lignification (Fig. 4); (ii) Cd stimulated lignification with a dose-independent manner, since both applied doses similarly enhanced the activity of enzymes involved in this pathway (Fig. 4), but slight lipid peroxidation and no alteration in antioxidant systems were seen in 20 μM Cd-exposed plants, in comparison to the 100-μM-treated ones (Table 1 and Fig. 2). This would suppose that the sequence of reactions leading to Cd-stimulation of lignification might not be initiated, at least directly, by the disturbance of the redox control in cell wall. It has been reported that metal toxicity in plants can be ascribed to an accumulation of endogenous phenolic compounds [42]. Phenols, including intermediates of lignin biosynthesis, can be oxidized to phenoxyl radicals by peroxidases to form polymers such as lignin in apoplast [43]. However, an intriguing question remains to know whether or not Cd and Cu would similarly act regarding the control of phenolic reactions. Different chemical behaviours (Cd is a non-redox-reactive heavy metal, but Cu is a transition metal) could play a prominent role in this way, as reported for other processes [4,11].
One conclusion to be reached is that Cd and Cu approximately cause the same oxidative stress, while they differently act on the enzyme activities involved in the cell growth limitation of pea leaves, at least at the applied concentrations and during the treatment period.