1 Introduction
Autographa californica multicapsid nucleopolyhedrovirus (AcMNPV), a member of the Baculoviridae family, is an enveloped virus with a circular, closed double-stranded 132-kb DNA genome. The virus undergoes a biphasic life cycle in insect cells. The early phase of infection provides about one fifth of the intracellular copies of viral proteins to be targeted for maturation at the cell surface for production of budded baculoviruses [1]. During the ongoing budding process, the membrane containing integral proteins from the insect cell lipid-bilayer are selectively displayed on the viral envelope. Recombinant AcMNPV has been extensively used as temporal gene expressing vehicle. Here, a recombinant baculovirus, AcGFPgp64 [2,3], displaying green fluorescent proteins (GFP) as a fusion to envelope protein gp64, was investigated.
Virus-like particles (VLPs) of human parvovirus B19, a member of the Parvoviridae family, have also been used as biotechnological and diagnostic tools [4]. B19 is a non-enveloped icosahedral virus with a single-stranded DNA genome. The genomic size is 5.6 kb and the diameter of the virion is approximately 22 nm. B19 infects erythroid cells [5,6], leading to a variety of diseases including anaemia, aplastic or hypoplastic crisis, prolonged bone marrow failure [7], and foetal hydrops [8,9]. Canine parvovirus (CPV) is also a small non-enveloped ssDNA virus, having icosahedral symmetry, and a diameter of approximately 26 nm. The capsid is composed of three structural proteins VP1, VP2, and VP3. CPV causes intestinal enteritis, myocarditis, and lymphopoenia in neonatal, young and older animals [10]. Full DNA-containing capsids have a total of 60 subunits of the combined viral proteins VP1, VP2 and VP3, the majority of which is VP2, with smaller amounts of VP1 and VP3 also present.
In the present study, three structurally modified viral particles were analysed by fluorescence correlation spectroscopy (FCS): (1) AcGFPgp64, a baculovirus displaying GFP as a fusion with its viral membrane protein gp64, (2) human parvovirus-like particles B19 (B19 VLPs) displaying enhanced green fluorescent protein (EGFP) at the N-terminus of its structural protein VP2, and (3) canine parvovirus-like particles (CPV VLPs) displaying EGFP at the N-terminus of its structural protein VP2. The corresponding fusion constructs were expressed in insect cells and the purified fluorescent viruses and VLPs were analysed by FCS.
2 Results and conclusions
In a previous work, FCS analysis of AcGFPgp64 viruses showed the presence of GFPgp64 fusion proteins at the membrane of the baculovirus [3]. The number of gp64 protein molecules packed in the wild-type baculovirus envelope is not known. Also, it is not known to what extent the wild-type gp64 proteins are substituted with the GFPgp64 fusion. However, it is known that the wild-type gp64 protein is present on the viral membrane as stable disulphide-linked trimers [11]. For all baculoviruses, this key protein gp64 plays an essential role before infection; it anchors the virus to the host cell membrane. Assuming that the GFPgp64 fusion is also present on the viral membrane as trimers, the data presented here suggests that at least three fusion protein molecules are released from the membrane as detergent solubilized complexes [3]. The measured diffusion time of 0.2 ms is reasonable for a trimer–detergent complex with a protein mass of 180 kDa [12]. It is significant that dithiotreitol (DTT) in the presence of SDS reduced the diffusion time of the fluorescent species further from the value obtained with SDS alone (Fig. 1, Table 1). This suggests that fluorescent disulphide-bonded molecules were present in the detergent-solubilized material and that they were dissociated in the presence of this reducing agent (Fig. 1).
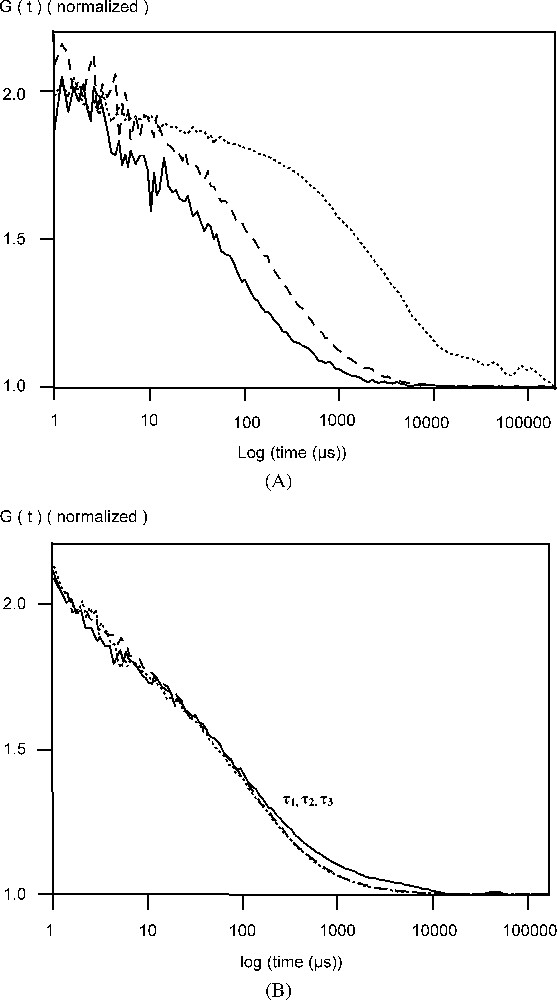
(A) Normalized autocorrelation curve of AcGFPgp64 baculovirus particles in phosphate buffered saline (PBS), pH 7.0 in the absence of SDS or DTT (…), in the presence of 5 mM SDS, (- -) and both 5 mM SDS and 25 mM DTT (—). (B) Normalized autocorrelation curve of soluble GFP in phosphate buffered saline (PBS), pH 7.0 in the absence of SDS or DTT (…), in the presence of 5 mM SDS, (- -) and both 5 mM SDS and 25 mM DTT (—).
Translational diffusion times ( and ), diffusion coefficients ( and ), hydrodynamic radii ( and ), and number of AcGFPgp64 virus particles before and after treatment with detergent SDS and reductive agent DTT
Particle | (ms) | (ms) | |||||
EGFP–CPV | 0.7 ± 0.3 | 0.3 ± 0.1 | 1.4 × 10−11 | 2.8 × 10−11 | 17 ± 5.0 | 8.5 ± 2.7 | 9.5 ± 0.7 |
EGFP–B19 | 0.6 ± 0.1 | 0.2 ± 0.0 | 1.8 × 10−11 | 5.0 × 10−11 | 14 ± 2.4 | 4.8 ± 0.1 | 9.0 ± 5.0 |
AcGFPgp64 (SDS) | 3.3 ± 0.8 | 0.2 ± 0.0 | 2.8 × 10−12 | 5.2 × 10−11 | 83 ± 19 | 4.6 ± 0.5 | 3.0 ± 0.0 |
AcGFPgp64 (DTT) | 0.2 ± 0.0 | 0.1 ± 0.0 | 5.2 × 10−11 | 1.1 × 10−10 | 4.6 ± 0.5 | 2.5 ± 0.4 | 4.5 ± 0.0 |
EGFP | 0.1 ± 0.0 | 0.1 ± 0.0 | 1.1 × 10−10 | 1.1 × 10−10 | 2.1 ± 0.2 | 2.1 ± 0.1 | 1.0 ± 0.0 |
Further characterization of the baculoviral particles showed that there might be more than one fluorescent fusion protein in the baculoviral membrane. This conclusion is supported by the analyses of the AcGFPgp64 baculovirus in the presence of both reagents: SDS, which will solubilize the lipid membrane and release the fusion protein to the solution, and the reducing agent DTT, which will cause the disulphide bonds between the fusion proteins to breakdown to complete the dissociation. The diffusion time for the baculovirus particles through an effective laser volume of 0.2 femtolitre was 3.3 ms (Table 1), corresponding to a diffusion coefficient, D, of . In the presence of 5 mM SDS, this slowly diffusing component rapidly turned into fast moving components with a diffusion times of 0.2 ms and a D of , with a release of a minimum of three fluorescent units from the viral envelope [3]. In order to distinguish different sized components in the FCS autocorrelation measurement, a difference in the diffusion time greater than 1.6-fold must be obtained. This criteria was fulfilled, since denaturation with 5 mM SDS, and then with 25 mM DTT caused a drop in the diffusion time from 0.2 to 0.1 ms, respectively (Fig. 1, Table 1). The change in the fluorescence fluctuation amplitude was also monitored, since the number of the particles in the effective measuring volume is inversely proportional to the amplitude. In the presence of 25 mM DTT, there was a 2-fold decrease in the amplitude of the autocorrelation function. Further, treatment with 25 mM DTT released a total of five (5) fluorescent units incorporated from the membrane (Table 1). In the presence of same concentrations of the detergent SDS and DTT, compared to PBS buffer alone, FCS showed that, for soluble EGFP, the diffusion time remained constant (Fig. 1B). Also, DTT did not change the photophysical properties of GFP in creating molecular triplet states, nor did it have any effect on the amplitude of the autocorrelation function by changing the molecular brightness of fluorescent species (data not shown). We also hypothesized that the GFPgp64 fusion proteins on the surface of the enveloped baculovirus form clusters after budding from the cell membrane. Since the number of non-fluorescent subunits remained unknown, clusters being either dimers or trimers still remain to be resolved.
Fluorescent VLPs of CPV and B19 were also studied by FCS. After incubation EGFP–CPV in 6 M urea for 15 min at 50 °C, the radius was reduced to at least 9 nm and there was a 6- to 10-fold increase in the particle number (Table 1, Fig. 2). For EGFP–B19 particles, the diffusion time was reduced from 0.6 to 0.2 ms, showing, that the EGFP fusions were incorporated in the capsid structure (Fig. 3). Following the breakdown of the fluorescent B19 VLPs, there was an increase in the number of particles by a factor of 9. For recombinant EGFP–B19 VLPs, the ratio of fluorescently labelled VP2 to non-labelled VP2 was 9 in 60, or 15% labelled subunits in a total 60 subunits. EGFP–CPV gave a ratio of 10 in 60, or 17% of labelled subunits in the VLP. This result is supported by the fact that CPV and B19 VLPs must contain 60 subunits in an icosohedron [13]. These results support the hypothesis that the fusion protein incorporated into the N-terminus of the structural protein VP2 in both B19 and CPV VLPs have the proteins projecting out from the five-fold axes of the icosahedral capsid structure, since an average of 9 to 10 EGFP–VP2 subunits are incorporated in both CPV and B19 VLPs, occupying most of the 12 five-fold sites.
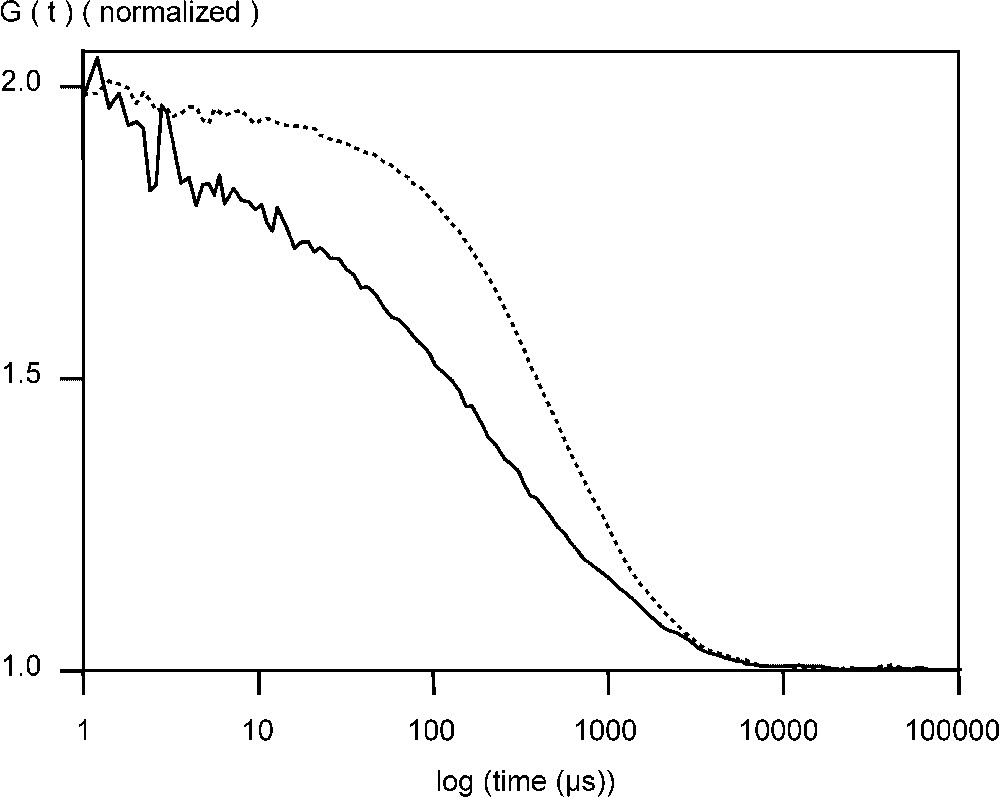
Normalized autocorrelation curve of EGFP–CPV VLPs measured at +20 °C phosphate buffered saline (PBS), pH 7.0, in the absence (- -) and presence of 6 mM urea (—) at +50 °C.

Normalized autocorrelation curve of EGFP–B19 VLPs measured at +20 °C in phosphate buffered saline (PBS), pH 7.0, in the absence (- -) and presence of 5 mM SDS (—).
3 Materials and methods
3.1 Cells and viruses
Spodoptera frugiperda (Sf9) insect cells were cultured at 28 °C as monolayer in plastic flasks (Greiner GmbH, Frickhausen, Germany) or in suspension in orbital shakers (130 rpm) using 250 ml Erlenmeyer flasks. Serum free HyQ SFX insect cell culture medium (HyClone Inc., Logan, UT) was used throughout the study. Cloning procedures for recombinant viral particles are described in more detail elsewhere [3,4,14]. The recombinant baculoviruses, AcGFPgp64, AcEGFP–VP2 CPV and AcEGFP–VP2 B19, were generated using the Bac-to-Bac™ system (Gibco BRL) based on transposon-mediated insertion of the foreign gene into the genome of the Autographa californica multicapsid nucleopolyhedrovirus (AcMNPV) [15]. Propagation of the recombinant viruses was performed according to standard procedures as described [16].
3.2 Chemical and temperature treatment of recombinant viral particles AcGFPgp64, EGFP–CPV and EGFP–B19
All reagents were obtained from Sigma-Aldrich Corp. (St. Louis, MO, USA). The purified baculovirus AcGFPgp64 was incubated in 5 mM SDS at room temperature for 10 min, following FCS measurements. The virus was further treated with 25 mM DTT (dithiotreitol) for 5 min and again analysed. EGFP–CPV VLPs were incubated for 15 min at 50 °C in the presence of 6 M urea and analysed. For EGFP–B19, the VLPs were treated with a 5 mM concentration of SDS, followed by FCS analysis.
3.3 Fluorescence correlation spectroscopy (FCS) analysis
FCS analysis was carried out at 20 °C using a ConfoCor2 instrument (Carl Zeiss, Jena, Germany) measuring samples in solution. An Ar-ion laser at 488 nm was used for excitation with a 530–600-nm BP (band-pass) filter. The instrument was operated in an autocorrelation mode and the manufacturer's software was used to calculate the autocorrelation function, diffusion time, and number of particles per observation volume. From these diffusion time values, obtained from the autocorrelation function, diffusion coefficients were estimated as follows. The diffusion time is defined as:
(1) |
(2) |