1 Introduction
The question of coevolution of insects and their host plants has been important in the studies of plant-animal trophic interactions since the pioneering paper of Ehrlich and Raven [1]. These interrelationships seem to be never-ending ‘armament race’ involving various defences of plants against being eaten and the adaptive responses of phytophagous insects. Hence, survival of interacting species in time is a result of a dynamic equilibrium that can be influenced by external factors [2,3]. The influence is manifested, e.g., in the behavioural changes, feeding strategies, the efficiency of digestion and resorption, what may hinder the vitality and fertility of adult individuals [4–6].
Proper quality and quantity of necessary nutritional compounds are the factors that ensure the developmental success of specialized herbivores, thus their digestive functions change in parallel with their specific food source. This can be manifested in a synthesis of a limited set of digestive enzymes, the relative activities of which reflect food preferences of an insect [7–10]. Polyphagous insects, e.g., Spodoptera littoralis caterpillars secrete a rich set of proteases, α-amylase and invertase; aphids (Hemiptera) sucking phloem sap have a relatively high level of invertase activity [8,11], and the insects fed on blood or keratin have a relatively high proteolytic activity [7].
Until now the analyses of the activity of digestive enzymes in butterflies have focused on pest and other economically important species. These analyses point out the significance of β-glycosidases as the enzymes capable of hydrolyzing plant glycosides [12–14]. However, there is lack of data concerning digestive enzymes of endangered and protected species, such as Apollo butterfly (Parnassius apollo L.).
In Europe P. apollo has evolved into many subspecies and forms, differing in their morphology (body size, wing-span and wing pattern), behaviour and biology, including food preferences [15–19]. Each of the subspecies developed adaptations to a particular, often isolated and small habitat [18,20,21].
The process of extinction of local Parnassius subspecies has been observed in the whole Europe for about 200 years [16–19,22,23]. Research programmes carried out along with the recovery plan of P. apollo ssp. frankenbergeri Slabý in the Pieniny National Park (southern Poland) explained some aspects of Apollo biology and ecology, including relations between the caterpillar and its host plant – the orpine (Sedum telephium ssp. maximum) [24–31].
Sedum telephium (L.) ssp. maximum (L.) Krocker is a perennial succulent rhizocarp with characteristic roots of an elongated bulb type, preferring arid and sunny sites, like xerothermic rock grasslands [32]. Such places are preferred by P. apollo, due to high insolation and food availability for the larvae [20]. Since sufficient amount of proper quality food limits the growth rate of the larvae, their developmental success strongly depends on the host plant phenology. This was shown in the studies on energy budget of the last larval instars of Apollo – they fed on Sedum leaves when their energetic value was the highest [27,33]. Gathering sufficient amount of energy reserves during the larval stage is important for completion of the development by successful pupation and emergence of the adult. Proteins accumulated during that period are an important source of amino acids for reproduction in adult stage [8], since flowers preferably visited by the butterflies contain mainly di- and monosaccharides [34,35].
Phytochemical studies of the aboveground parts of S. telephium ssp. maximum revealed low contents of organic substances (about 8% wet weight of leaves), mainly organic acids, amino acids and saccharides [36]. Among secondary compounds the presence of phenolic acids and small amounts of non-phototoxic coumarins were stated. No alkaloids or their derivatives were detected [33,37]. However, neither clear changes of phenolic acids concentrations during the vegetation period, nor increase in their content in the response to larval feeding were stated so far. This leads to a conclusion that the secondary compounds present in S. telephium ssp. maximum are not toxic enough to repel effectively Apollo caterpillars from feeding on the plant leaves. On the other hand the presence of a constitutive defence system that depends on the changes of the plant metabolism is quite plausible, since in natural conditions larvae eat no more than about 30% of the leaves area of one plant and then move to another one [24,29]. Following that, one may speculate that a dynamic equilibrium between P. apollo larvae and their host plant results in an ‘acceptable’ ratio of digested and absorbed nutrients to secondary compounds. However, understanding of the digestion processes, contributing to that ‘equilibrium state’ is still insufficient, since there are no relevant data concerning this species. This raises a need to fill that lack of information.
In this part of our project, we focused on the digestive capacity of the larvae to utilize carbohydrates and other compounds containing glycosidic bonds as energy sources. Moreover, the relations between the enzymes activities and the rate of food intake and the body mass of Apollo butterfly larvae were analysed.
2 Materials and methods
The larvae of Parnassius apollo ssp. frankenbergeri Slabý originated from a semi-natural open-field breeding colony in the Pieniny National Park, set up for a recovery plan in the Pieniny Mts. The individuals for enzymatic analyses were obtained from additional eggs laid by Apollo females in the preceding year. In accordance with the permission from the Polish Ministry of Environment, the larvae of the fourth (L4) and fifth (L5) instars were chosen from the stock colony. Morphological and behavioural criteria allowed further separation of L5 individuals into two subgroups: ‘young’ – larvae up to the third day after moulting, and ‘hyperphagic’ – larvae that most of the time spent on feeding. Hence, the gut size and the amount of digested food were the major differences between the subgroups.
The larvae were anaesthetised with CO2 and kept at low temperature until dissection in 1.15% KCl solution at 4 °C. The midgut was cut out between a crop and a hindgut. The remains of trachea, fat body and Malpighian tubules were removed. The dissected midgut was cut open and rinsed in 0.1 M phosphate buffer (pH 7.4) containing 1 mM EDTA and 10% glycerol to obtain gut contents enclosed in peritrophic membrane. The cleared midgut wall was homogenised in the same buffer and then centrifuged for 10 min () at 4 °C. The midgut contents were centrifuged at for 10 min in order to remove the undigested leaf pieces. The resulting MT and MC supernatants were portioned out into Eppendorff tubes, and kept frozen at until the analyses of enzymes activities. Hence, from one individual, two samples were obtained: the midgut tissue together with glycocalyx (MT) and the liquid gut contents with peritrophic membrane (MC).
Appropriate pH optima for α-amylase, saccharase and α-glucosidase were assessed using 0.05 M citrate-phosphate (Mc Ilvaine's) (pH 2.6–7.0), 0.1 M phosphate (pH 5.8–7.8), 0.05 M Tris-HCl (pH 7.4–9.0) and 0.1 M bicarbonate (pH 9.2–10.7) buffers. Ionic strength of the buffers was adjusted to the values applied to other Lepidoptera (see Table 1 for references). Following the recommendation of the International Union of Biochemistry and Molecular Biology, all the enzymatic assays in P. apollo were done at 30 °C.
Assay conditions and methods used in the determination of glycosidases
Enzyme | Substrate | Buffer for substrate⁎ | Homogenate volume [μl] | Incubation [min] | Termination | Further procedures | Final vol. [ml] | Final product | λ [nm] | Comments | References |
α-Amylase | 250 μl 0.5% soluble starch | bicarbonate: 0.05 M, pH 9.4; 20 mM NaCl, 0.1 mM CaCl2 | 50 | 30 | 500 μl 3,5-dinitro-salicylic acid reagent | 10 min in water bath (100 °C) + 750 μl H2O | 1.55 | maltose | 540 | measurement after 10 min (until 1 h) | [38] modified |
Cellulase | 250 μl 1% carboxymethyl-cellulose Na-salt | citrate-phosphate: 0.05 M, pH 5.8 | 60a or 120b | cellobiose | |||||||
Maltase | 15 μl 0.056 M maltose | citrate-phosphate: 0.05 M, pH 5.2 | 15 | 60a or 120b | 300 μl 5% TCA | 100 μl (of 330 μl mixture) + 1.5 ml working reagent from diagnostic kit; incubation: 15 min at 37 °C | 1.6 | glucose | 500 | working reagent (pH 7.0): GOD, POD, 4-amino-antypiryne – “Biochemtest Glukoza et New” (POCh S.A.); measurement until 30 min | [39] modified |
Cellobiase | 15 μl 0.056 M cellobiose | ||||||||||
Lactase | 15 μl 0.056 M lactose | ||||||||||
Trehalase | 15 μl 0.056 M trehalose | ||||||||||
Saccharase | 15 μl 0.056 M saccharose | ||||||||||
α-Glucosidase | 50 μl 20 mM NP-α-Glu | citrate-phosphate: 0.05 M, pH 5.2 | 50c | 15a or 30b | 500 μl 1% SDS in 0.1 M bicarbonate buffer, pH 10.4 | – | 0.6 | p-nitro-phenol | 420 | measurement until 120 min | [40,41] modified |
β-Glucosidase | 50 μl 20 mM NP-β-Glu | 10a or 30b | |||||||||
α-Galactosidase | 50 μl 8 mM NP-α-Gal | 50 | 15a or 30b | ||||||||
β-Galactosidase | 50 μl 20 mM NP-β-Gal | 10a or 30b |
⁎ pH values of assay mixture are given;
a midgut tissue samples;
b liquid midgut contents samples;
c midgut tissue samples 25-fold diluted in homogenisation buffer.
The procedures of the enzymatic assays as well as substrates used for analyses are summarized in Table 1. Routine procedures were applied with initial substrate concentration ensuring linear reaction for the incubation time. This was confirmed for the most active α-glucosidase. The reaction was linear at least four times longer than the incubation time () (Table 1). For this enzyme, temperature dependence (16–37 °C) of the reaction rate was measured as well. Enzymatic activities were assayed colorimetrically and expressed per milligram of protein content, determined according to Bradford [42]. Due to a limited number of available individuals for planned analyses, enzymatic assay for each larva was duplicated and the obtained mean taken as single result for further calculations.
The significance of differences was assessed with ANOVA/MANOVA. T Tukey's test for unequal sample size was applied for post hoc analysis. Test t for dependent variables allowed estimation of significant differences within MT and MC samples in the same larval instar (). Pearson's linear correlation coefficients between activities of the enzymes were calculated at .
3 Results
The highest activity of α-amylase, measured in relation to various media, was stated at pH 9.0. For saccharase, it was at pH 5.6, and for α-glucosidase, in the 4.9–5.6 pH range in citrate–phosphate buffer for both enzymes (Fig. 1). For the latter, the increase of incubation temperature from 30 to 37 °C doubled the enzyme activity. In comparison with 16 °C, the rate of hydrolysis at 37 °C was 7.4-fold higher.
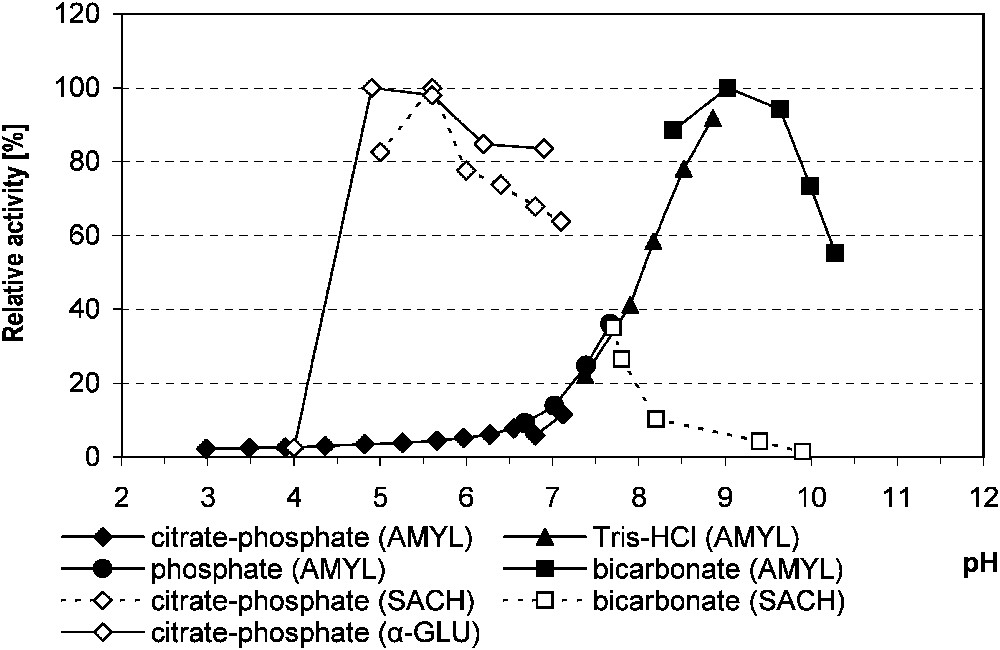
The relative activity of midgut α-amylase, saccharase and α-glucosidase in Parnassius apollo larvae in relation to the buffer pH. Abbreviations: AMYL (α-amylase), α-GLU (α-glucosidase), SACH (saccharase).
In general, the enzymes hydrolysing polysaccharidic linkages had higher activity in the samples of liquid gut contents (MC), contrary to the activities of disaccharidases and specific glycosidases that were higher in the midgut tissue with glycocalyx (MT). The ‘young’ and ‘hyperphagic’ L5 larvae did not differ significantly in their efficiency toward polysaccharides and disaccharides digestion. Hence, these results were pooled for comparison with the corresponding activities measured in L4 larvae (Table 2).
Glycosidases activity in the midgut of Parnassius apollo larvae (mean±SD)
Enzyme | L4 | L5 | ||
[μmol of product ]⁎ | MC () | MT () | MC () | MT () |
α-Amylase | 73.337 ± 23.082 | 9.677 ± 5.341 | 104.934b ± 48.798 | 19.478b ± 7.635 |
Cellulase | 0.187 ± 0.228 | 0.063 ± 0.047 | 0.750b ± 0.704 | 0.075b ± 0.058 |
Maltase | 0.072a ± 0.078 | 0.244a ± 0.104 | 0.024b ± 0.022 | 0.277b ± 0.070 |
Cellobiase | 0.004 ± 0.006 | 0.038A ± 0.028 | 0.009b ± 0.009 | 0.101Ab ± 0.058 |
Lactase | 0.008 ± 0.020 | 0 | 0.006 ± 0.010 | 0.020 ± 0.017 |
Trehalase | 0.001a ± 0.002 | 0.043a ± 0.024 | 0.008b ± 0.016 | 0.034b ± 0.011 |
Saccharase | 0.162 ± 0.189 | 0.353 ± 0.151 | 0.221 ± 0.505 | 0.491 ± 0.214 |
α-Glucosidase | 2.431 ± 1.734 | 9.050A ± 5.028 | 2.128b ± 1.666 | 16.650Ab ± 6.383 |
β-Glucosidase | 0.526 ± 0.161 | 0.740A ± 0.343 | 0.671a ± 0.447 | 2.607Aa ± 1.227 |
α-Galactosidase | 0.205 ± 0.085 | 0.195 ± 0.067 | 0.128b ± 0.107 | 0.334b ± 0.134 |
β-Galactosidase | 0.282 ± 0.099 | 0.296A ± 0.066 | 0.213b ± 0.170 | 0.830Ab ± 0.312 |
⁎ For product description see Table 1 (disaccharidases activity is expressed in μmol of hydrolysed substrate ); n, number of individuals used for statistical analysis.
a,b Significant statistical differences (, T Tukey's test for unequal sample size) between enzyme activity in MT and MC samples of the same stage larvae (a, L4; b, L5).
A Significant statistical differences (, T Tukey's test for unequal sample size) between enzyme activity in MT samples of two different stages larvae.
The activities of α-amylase and cellulase in L5 larvae were significantly higher in MC in comparison with MT ( and , respectively). Similar, although not significant, results were obtained in the L4 larvae. The activity of cellulase in a given compartment was much lower than that of α-amylase (Table 2, Fig. 2).
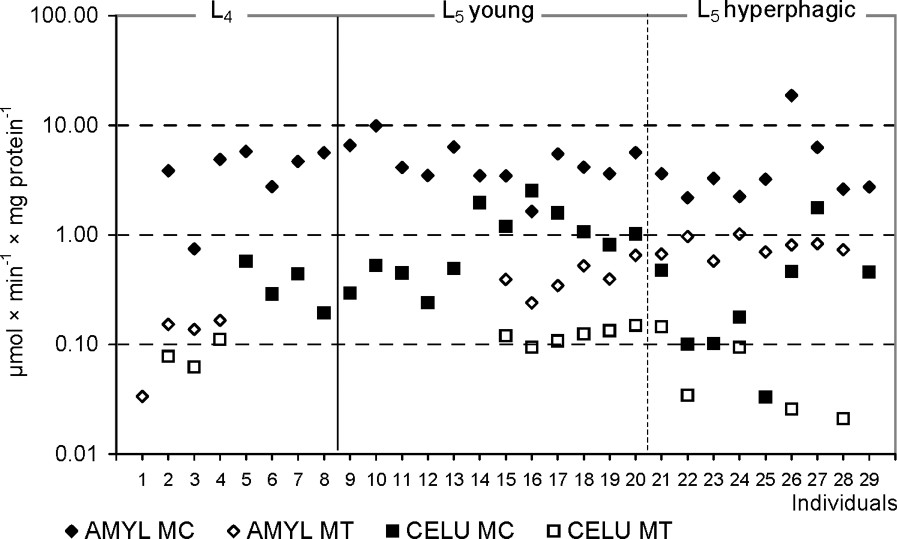
The amylolytic and cellulolytic activities in the midgut tissues (MT) and the liquid midgut contents (MC) in individuals of Parnassius apollo larvae. Abbreviations: AMYL (α-amylase), CELU (cellulase).
On the contrary, the activities of disaccharidases were higher in MT than MC samples. Assayed MT disaccharidases of the L5 differed in specific activities among themselves (, ). The highest total hydrolytic activity was stated for saccharase – it was twice as high as the activity of maltase (, ). The activity of lactase was practically not detected in either of the fraction or the instars. The activity of disaccharidases in the L4 did not differ significantly from those in the L5, except for cellobiase activity in MT samples (). The differences in the activity of enzymes in studied midgut compartments of the L4 caterpillars were found only for maltase and trehalase ( and , respectively), the activities of which were always higher in MT samples.
Assays of specific glycosidases activities provide another insight into the digestion of oligomeric compounds containing glycosidic linkages. Hence, MT samples were much more active in this respect than MC ones. In this compartment the total hydrolytic activity of glucosidases was higher than that of galactosidases, with the highest activity of α-glucosidase (Fig. 3). Among the assayed galactosidases, a higher activity was stated for β-galactosidase. Hydrolytic activities of both glucosidases and β-galactosidase in MT of the L5 larvae were significantly higher than in the previous instar. Comparison of ‘young’ and ‘hyperphagic’ larvae revealed significantly higher activities of assayed glucosidases and galactosidases in MT samples of the latter (Fig. 4). Hence, activities of these enzymes in L4 larvae were lower than in ‘young’ individuals of the last instar. No differences in the specific glycosidases activity were found between liquid midgut contents (MC) of both larval instars as well as between the midgut compartments in the L4 (Table 2).
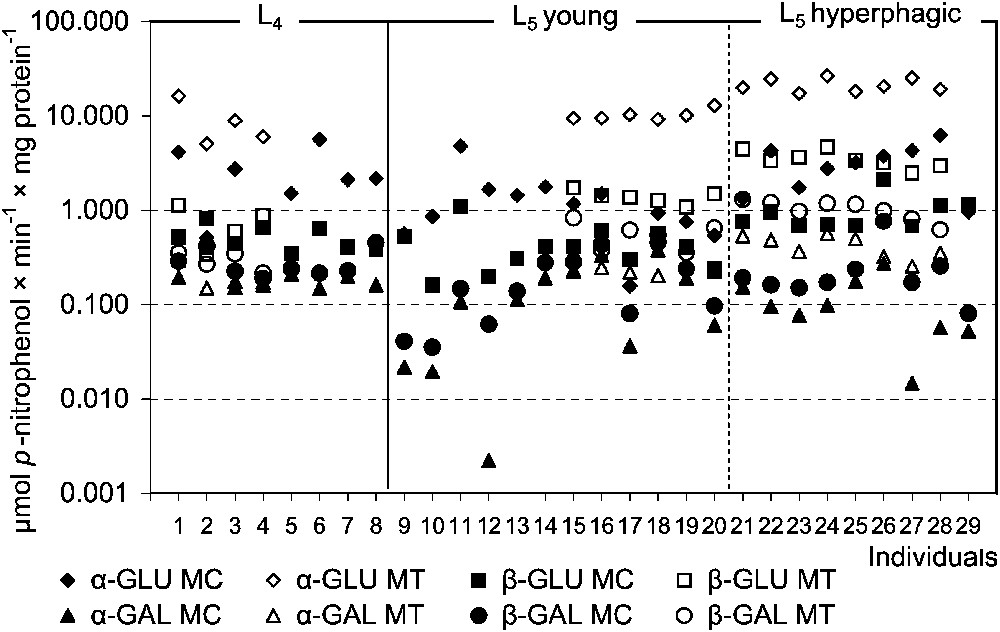
The specific glycosidases activities in the midgut tissues (MT) and the liquid midgut contents (MC) in individuals of Parnassius apollo larvae. Abbreviations: α-GAL (α-galactosidase), β-GAL (β-galactosidase), α-GLU (α-glucosidase), β-GLU (β-glucosidase).
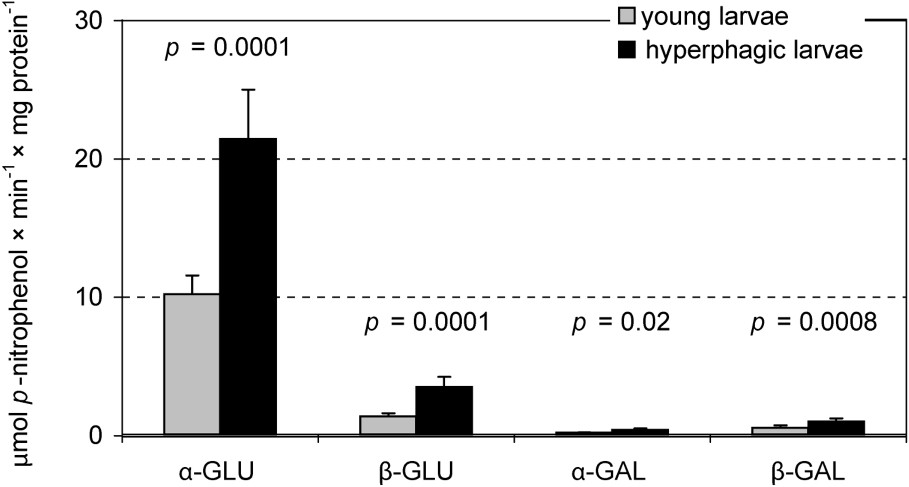
Specific glycosidases activities in the midgut tissue samples in the ‘young’ (n=6) and the ‘hyperphagic’ (n=8) L5 Parnassius apollo larvae (‘subgroups’). Values of p (T Tukey's test for unequal sample size) show differences in the enzyme activity between the ‘subgroups’. Abbreviations: α-GAL (α-galactosidase), β-GAL (β-galactosidase), α-GLU (α-glucosidase), β-GLU (β-glucosidase).
The activities of enzymes hydrolysing α-glucosidic linkages in starch (α-amylase, maltase/α-glucosidase) from the samples of the midgut tissue and the liquid gut contents of the L5 larvae were highly and positively correlated with one another (Table 3). It is noteworthy that α-amylase activity was much higher than that of maltase in both gut compartments. This points out relative abundance of poly- and oligosaccharides (consisting of more than six saccharide units) in the leaf tissue. Significant positive correlations between amylase and α-glucosidase activities and body mass of L5 larvae strongly indicate a nutritional role of these saccharides (Table 4).
Significant correlations between activities of assayed glycolytic enzymes in the midgut tissues (MT) and the liquid midgut contents (MC) of Parnassius apollo L5 larvae
Midgut compartment | n | Regression equation | r | p | T |
MT | 14 | 0.956 | 9.33×10−8 | 11.31 | |
MT | 14 | 0.934 | 9.9×10−7 | 9.09 | |
MT | 14 | 0.908 | 7.2×10−6 | 7.50 | |
MT | 14 | 0.887 | 2.4×10−5 | 6.64 | |
MT | 14 | 0.873 | 4.4×10−5 | 6.22 | |
MT | 14 | 0.819 | 3.0×10−4 | 4.96 | |
MT | 14 | 0.819 | 3.0×10−4 | 4.96 | |
MT | 14 | 0.737 | 0.003 | 3.78 | |
MT | 14 | 0.731 | 0.003 | 3.71 | |
MC | 21 | 0.705 | 3.0×10−4 | 4.34 | |
MT | 14 | 0.694 | 0.006 | 3.34 | |
MC | 21 | 0.609 | 0.003 | 3.34 | |
MT | 14 | −0.584 | 0.03 | −2.49 |
Significant correlations between the enzymes activities and body mass (bm) of Parnassius apollo L5 larvae
Midgut compartment | n | Regression equation | r | p | T |
MT | 14 | 0.756 | 0.003 | 3.83 | |
MT | 14 | 0.748 | 0.003 | 3.74 | |
MT | 14 | 0.739 | 0.004 | 3.64 | |
MT | 13 | 0.607 | 0.03 | 2.54 | |
MC | 20 | −0.647 | 0.002 | −3.60 |
Digestion of β-glucosidic linkages in plant β-glucosides presented a different picture. Negative correlation between activities of cellulase and cellobiase in the MT samples of the last instar was stated. There was no correlation between the enzymes in MC, as well as between the activities of cellulase and β-glucosidase in both compartments (Table 3). Cellulase activity in MC was negatively correlated with the body mass of that instar (Table 4). On the contrary, cellobiase activity very strongly correlated with β-glucosidase activities in MT samples of the L5 (Table 3, Fig. 5). These findings indicate a negligible nutritional role of the cellulose, although shorter β-glucosides may have some importance.
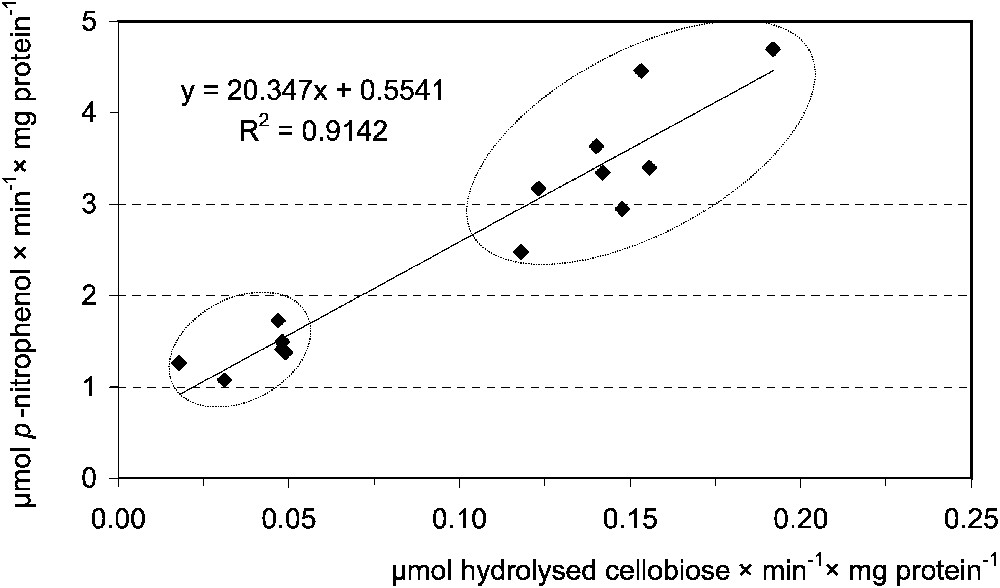
Correlation between the midgut tissue cellobiase [μmol hydrolysed cellobiose ] and β-glucosidase [μmol p-nitrophenol ] activities in Parnassius apollo L5 larvae. Lower ellypsis groups, ‘young’, upper, ‘hyperphagic’ larvae.
Positive and significant correlations between various enzymes hydrolysing glycosidic linkages were stated for p-nitroanilide derivatives only in midgut tissue. They point out the possibility of simultaneous digestion of different saccharide-containing compounds (Table 3).
4 Discussion
Alkaline pH optima for the assayed enzymes in P. apollo larvae were similar to the ones stated for the majority of the examined lepidopterans. The highest activity of α-amylase in Erinnyis ello (Sphingidae) and Ephestia cautella (Phyticidae) larvae was observed at pH 9.8 and 9.0, respectively [11,43]. Optimal pH for starch hydrolysis may have a broad range (e.g., from 5.5 to 9.0 in Earias vitella (Noctuidae)) [44], but this was not the case for Apollo larvae.
On the contrary, saccharase and α-glucosidase in the examined species had the highest activity in slightly acidic conditions. In other lepidopteran larvae, the optimal pH for disaccharidases and specific glycosidases ranges between 5.3 for trehalase in wax moth Galleria mellonella (Pyralidae) [45] and 8.0 for α-glucosidase in L3 larvae of E. vitella [44].
According to van't Hoff's law (Q10 law), the metabolic rate of an ectotherm doubles for every 10 °C increase in environmental temperature. Thus, increasing temperature results in internal changes that have an exponential effect on metabolism, hence various enzymes activities. Q10 values for α-glucosidase activity in the L5 P. apollo larvae were 2.4 for 16–24 °C and 2.5 for 30–37 °C range. Wide temperature range of this hydrolytic activity may reflect adaptation to high daily and seasonal temperature amplitudes (typical of mountain biotopes). Measurements done with P. apollo ssp. vinningensis proved that body temperature of dark brown caterpillar rearing in sunny places reached over 45 °C [46]. Such high temperatures are tolerated by Apollo larvae, although they seem to be above optimum for feeding and digestion, since the highest value () was stated for the 24–33 °C range. Tolerance of midgut glycolytic enzyme (trehalase) to high temperatures was stated also in tropical Orthaga exvinacea (Pyralidae) [47].
The activity of α-amylase in P. apollo was much higher than that of cellulase. Various authors use different basis for expression of the enzyme activities (per tissue fresh weight, per individual or per the whole organ). Hence, it is difficult to compare the obtained results with data provided by other authors [11,43]. The activity of α-amylase in P. apollo midgut detected mainly in the endoperitrophic space may result from a mechanism of enzyme production, storage and secretion by epithelium cells, similar to that stated in larvae of E. ello [43] and Spodoptera frugiperda (Noctuidae) [48]. These authors suggested that α-amylase is secreted into the gut lumen in small vesicles via microapocrine process. The enzyme is then released from the vesicle or, still bound with the vesicle membrane, is incorporated into the peritrophic membrane. Confirmation of this mechanism and identification of α-amylase distribution in P. apollo midgut needs further studies with immunocytochemical methods.
Cellulase is usually produced by the endosymbionts living in the insect alimentary tract [49]. In P. apollo larvae, this issue has not been studied so far. There is indirect evidence against their presence. Firstly, the cut leaf pieces pass quickly through the gut, giving little time for digestion by microbiotic organisms. Secondly, undigested fragments of orpine tissues, which can be easily observed during preparation of the gut, were not crushed and macerated. Thirdly, dissection of the gut revealed no structures, where the symbionts could survive. However, negative correlation between cellulase and cellobiase activity in MT samples and lack of correlation between cellulase and β-glucosidase activities (see Table 3) suggest that the cellulase activity detected in the samples was of exogenous origin. Since microbiota can reside in the gut also in the absence of any special structures (e.g., adhered to the peritrophic membrane or attached to the midgut surface), we cannot univocally say that they do not participate in the β-glucanase activity found in MC samples [50].
Disaccharidases and specific glycosidases activities detected in the midgut tissue of Apollo larvae indicate their presence in the glycocalyx or exoperitrophic space. They were also found in gut tissue of E. ello [43] and S. frugiperda [51]. Higher activities of these enzymes in Apollo MT samples support the view that final digestion in evolutionary advanced insects (e.g., Diptera, Lepidoptera) depends on the enzymes immobilised on the surface of midgut cells [52]. Our results suggest that in Apollo larvae saccharose is probably the most effectively hydrolysed disaccharide. This agrees with the finding that it is the main dicarbohydrate in host plant species S. telephium [36].
Trehalase activity in midgut tissue of P. apollo L4 and L5 larvae was 20- and 2-fold lower than in O. exvinacea and Lymantria dispar (Lymantridae), respectively [47,53]. Trehalose is an important energy source in insects and trehalase activity may serve as an indicator of energetic reserves resulting from the availability of carbohydrate compounds in their food [8]. Trehalose may also maintain nutrient absorption, osmotic regulation and other processes in midgut tissue [53]. Trehalose passes, along the concentration gradient, from the hemocel to the midgut lumen, hence low activity of trehalase in the Apollo midgut tissue may indicate low reserves of energy or a slight loss of this disaccharide resulting from its diffusion, and/or low intensity of the processes mentioned above. Possible inhibition of the enzyme activity by toxic β-glucosides and their aglycones from the Apollo food-plant remains to be justified [50]. Very low trehalase activity in the liquid midgut contents samples may also suggest the lack or negligible amount of trehalose in orpine leaves.
The ability to hydrolyse cellobiose may result either from a distinct enzyme, which ensures the complex cellulose digestion, together with β-glucosidase, or from the activity of A class β-glycosidase (former class 1 [52]), the active centre of which can bind a wide spectrum of substrates [50]. The earlier investigations revealed that S. frugiperda larvae have only one hydrolytic enzyme breaking down β-glycosidic bonds. The activity of this enzyme towards various substrates was the same in all cytosol fractions of the midgut tissue. Apart from this fact, the larvae have a well-developed detoxifying system for the neutralisation of toxic aglycones, released during digestion of plant glycosides. According to Ferreira and co-workers, this feature is characteristic for the more evolved insects [13,14]. To utilize plant glycosides as an energy source and avoid poisoning, less specialised insects selectively decrease the activity of β-glucosidase enzymes, responsible for the release of toxic aglycones [12]. Then the range of various β-glycosidic enzymes synthesized in the gut of particular species may reflect this coevolutionary trade-off [14]. Very high, in comparison to other Lepidoptera, β-glucosidase activity found in Apollo larvae suggest that its feeding strategy allows utilisation of at least some compounds containing β-glycosidic bonds. High activities of detoxifying enzymes in P. apollo midgut tissues [33] support this suggestion.
Yu [12] found that β-glucosidase activity increases in subsequent noctuid larval instars. Cellobiase/β-glucosidase, β-galactosidase and also α-glucosidase activities were higher in L5 than in L4 also in P. apollo larvae (Table 2). Such an increase in glycosidic enzymes activities may result from the greater food intake by older instars. Higher average glycosidic activity (Fig. 4) in MT samples from hyperphagic larvae fits well with this suggestion. Fast passage of chyme along an alimentary tract forces an insect to enhance the production of digestive enzymes to a level ensuring sufficient amounts of nutrients for absorption. Similar differences in cellobiase and β-glucosidase activities in hyperphagic and younger larvae, as well as strong positive correlation between these enzymes (Fig. 5, Table 3), suggest involvement of the same enzyme in digestion of glucose-containing plant compounds.
The lowest activity of α-galactosidase stated in P. apollo larvae may result from its low affinity for the substrate used – NP-α-Gal (p-nitrophenyl α-galactopyranoside). This was described in S. frugiperda (high for NP-α-Gal), hence the enzyme activity towards this substrate resulted only from high [51]. It does not seem possible that α-galactosidase activity in Apollo caterpillars is of endosymbiotic origin.
Yu [12] and Ferreira and co-workers [14] demonstrated that there was no correlation between the glycosidic activity and extent of insect polyphagy. However, the studied monophagous P. apollo subspecies [31] should develop a strategy of efficient utilisation of nutritional components, also secondary compounds, present in the host plant. One of the reasons is the very high water content of the leaves () [27].
It is concluded then that Parnassius apollo ssp. frankenbergeri caterpillars are equipped with a wide range of glycolytic enzymes. High α-amylolytic, but also other glycosidic activities, indicate that apart from starch also other compounds, including plant allochemicals, are utilized as energy sources. The set of digestive enzymes present in the gut of Apollo larvae probably reflects strict adaptation to its host plant phenology. This is supported by our earlier studies on larval development on other Sedum plants [31], as well as by other researchers and Apollo caterpillars breeders [6,54–56]. Incompatibility in caterpillars and host plant development (caused by, e.g., weather anomalies [24,26,28]) or experimental changes in food quality (e.g., caused by heavy metals pollution [25,30,56]) affected larval development into adult and their survival [31].
Acknowledgements
This work was supported by the Polish State Committee for Scientific Research (Project No. 6 P04F 085 21). We express our thanks to Managing Director and Scientific Board of The Pieniny National Park for administrative support and Mr Tadeusz Oleś for his expertise and help in Apollo breeding.