1 Introduction
Self-renewable haematopoietic stem cells (HSCs) make up a finite pool, from which they are mobilized during the whole lifetime upon physiological requirement. This pool is supposed to segregate during development. The circumstances of this segregation, an active field of study for nearly a century, are still fraught with enigmas. Originally, it was thought that this event occurred during a short period of the embryonic life; it was since found that commitment of HSCs is a more durable process that moves from a first site, the yolk sac, to a succession of others during embryonic and early foetal life (Fig. 1). Whether the whole span of sites and the developmental period involved has now been described is, in my mind, an open question. The mechanisms of HSC commitment during development include tissue interactions, intercellular signalling, and activation of transcription factors. Understanding the whole sequence would undoubtedly bring about information on the possibilities of waking this process up in later life, an interesting prospect for cell therapy.
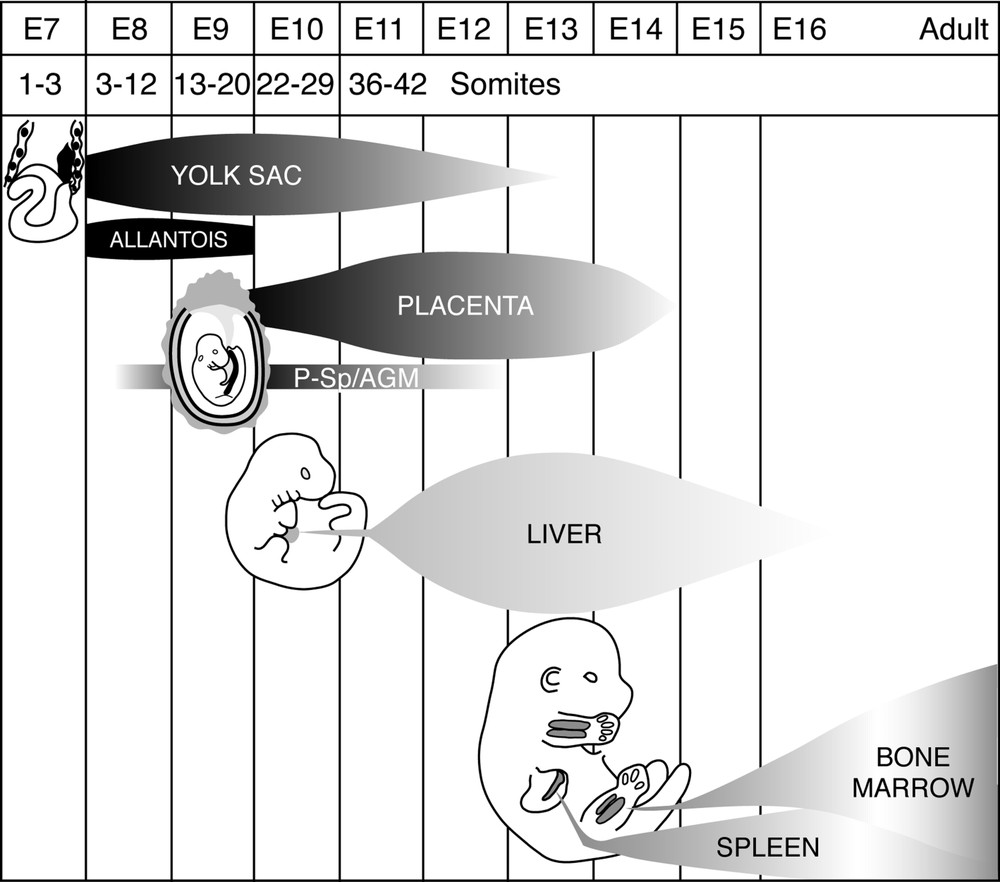
Synopsis of the development of the haematopoietic system in the mouse embryo and foetus. Extra-embryonic appendages and intra-embryonic organs are involved successively or in parallel in the emission of HSCs (yolk sac, allantois, aortic region – designated as P-SP/AGM for para-aortic splanchnopleura/aorta-gonad-mesonephros –, placenta), and others in the amplification and differentiation of this cell population (liver, spleen, bone marrow). A sequence of sites, exemplified here in the mouse, is the rule in all vertebrates.
2 Sites of HSC emergence
Sites where HSCs expand and differentiate are usually distinct from sites where they become committed. This feature was uncovered through experimental approaches in which organ rudiments were retrieved from an embryo and grafted into another, donor and host differing by a marker to diagnose the origin of cell types in the differentiated organ. This approach was originally devised in a chicken/chicken model and perfected in the quail/chicken model [1]. In the chicken model, the marker relied on the sexual chromosomes in male/female combinations. In the quail/chicken model [2], the marker was provided by the difference in heterochromatin appearance. Thymus, avian bursa of Fabricius, mammalian foetal liver, spleen, bone marrow, were shown to include a stromal compartment derived from the rudiment, and a haematopoietic compartment entirely derived from blood-borne progenitors. The only organ originally recognized as producing HSCs was the yolk sac, hence the hypothesis attributing to this appendage a unique role in the formation of the haematopoietic system [3].
However, a yolk sac-independent source of HSCs was uncovered by means of chimeric embryos, in which a quail body was engrafted onto a chicken yolk sac [4]. The region of the aorta was then shown, both in the avian and mammalian embryo, to produce HSCs for a short period [5], and was considered until recently as the ultimate site of HSC emergence.
The avian model recently permitted the discovery of a similar role for the allantois. In the avian embryo, the allantoic rudiment is a late-forming bud, emitted by the caudal intestine 24 h after gastrulation has ended. It becomes vascularised by in-situ-committed angioblasts [6] and displays haemoglobinised cell groups that look very much like blood islands. When the still unvascularised quail allantoic rudiment was grafted in a chicken host coelom, the bone marrow of the host became significantly colonized by quail haematopoietic cells (up to 8% of the total haematopoietic population). Furthermore, quail endothelial cells also appeared in the host medullary vascular network [6,7]. In this set of experiments, quail cells were sorted out or stained by means of the hemangioblastic quail-specific antibody QH1 [8].
These data prompted the search for a haematopoietic role of the mammalian placenta, since this appendage forms through the fusion of the allantois with the ectoplacental cone (Fig. 2). In a first approach, in vitro clonogenic progenitors were shown to be abundant in the placenta [9]. They were found consistently from day 10 of gestation, until day 17. Interestingly, in comparison with the foetal liver – a rudiment in which, as seen above, the haematopoietic population is entirely of extrinsic origin –, the quantitative and qualitative composition of the placental haematopoietic population displays specific traits:
- – it is first detected 24 h earlier than in the liver;
- – the proportion of early versus late progenitors is much higher at all stages than in the liver (Fig. 3).
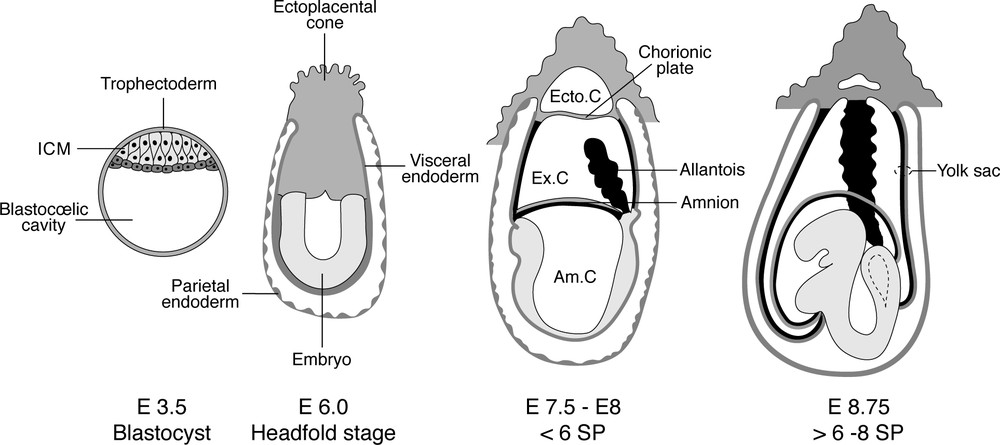
Development of the mouse embryo. The placenta forms through the fusion of the allantois to the chorionic plate occurring between the stages of six and eight pairs of somites, and becomes vascularised at the same time. Am.C: amniotic cavity. Ecto.C: ectoplacental cavity. Ex.C: exocoelom. ICM: inner cell mass. SP: somite pairs.
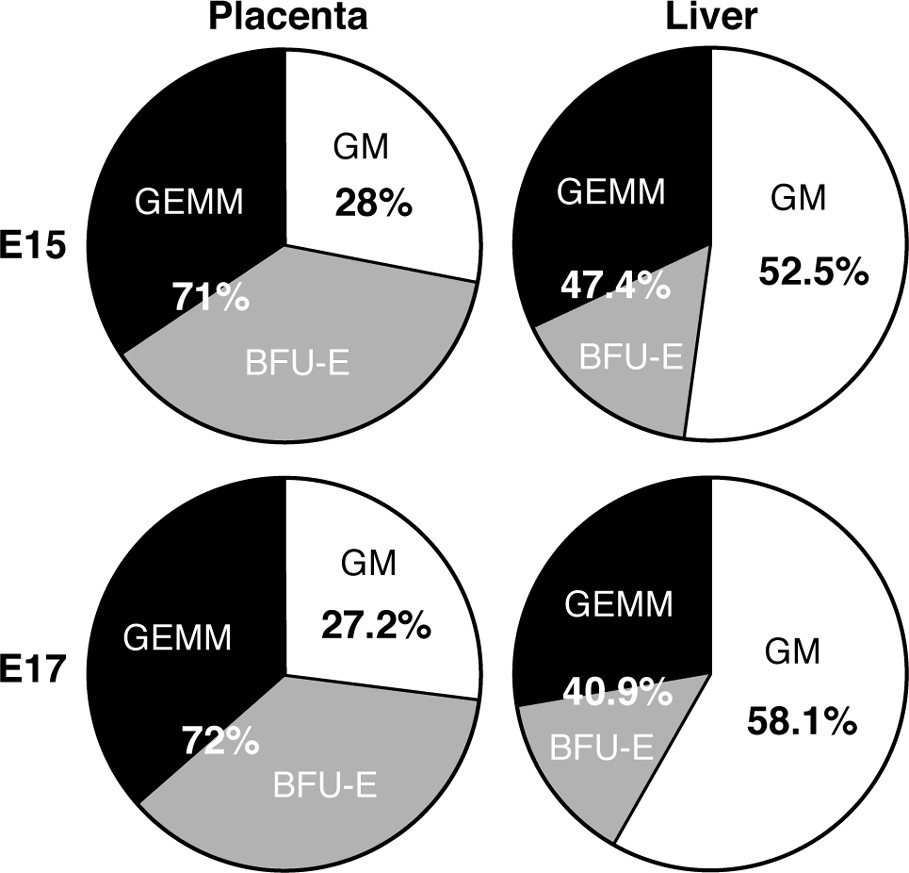
The placenta is rich in early clonogenic progenitors (GEMM: granulocyte/erythrocyte/macrophage/monocyte colonies; BFU-E: burst forming unit/erythroid) compared to the liver, where committed GM (granulocyte/macrophage) colonies are more abundant. E15, 17: days of gestation.
The second aspect concerns the origin of the HSCs or progenitors that are present in the placenta: do they become committed in situ or do they colonize the placenta? In order to determine this point, experiments must be carried out at very early stages, prior to vascularisation, which occurs in the allantois between the stages of six and eight pairs of somites, prior to the formation of the placenta. In view of the minute number of cells that can be obtained from these early rudiments, the clonogenic methodology was again applied [11]. As recorded above, progenitors were first detected in the placenta at the stage of 20 pairs of somites. It was thus unlikely to find them in the allantois prior to the 6-somite stage. In order to allow the cell interactions required for HSC commitment, allantoic buds retrieved between one and six pairs of somites were precultured in toto prior to dissociation. In these conditions, colonies developed from these early rudiments, while the possibility of colonization was excluded. Two studies carried out in parallel, with protocols differing only in slight detail, arrived at the same conclusion [11,12].
Thus, it can be concluded that the placenta is a site where HSCs become committed. Indeed, it is likely that this organ plays a major role in the development of the haematopoietic system, probably more important than the aortic region. The period of placental activity lasts 4 days, versus 36 h in the aortic region; furthermore, the number of progenitors or HSCs found is much larger in the placenta (Table 1).
Comparison of LTR HSC numbers between placenta (Pl) and aortic region (AGM). At E10.5, the caudal half of the embryo, which contains the AGM, was used. The figures are the numbers of ‘long-term reconstituting units per embryo equivalent’ = RU.ee (number of reconstituted receivers/total number of receivers/transplanted dose; the number of cells obtained at these stages from the pool of embryos used for reconstitution is too small to be counted). The number of RU.ee is similar in the two sites at E10.5 and E11.5. It is multiplied by 20 in the placenta at E12.5, while it remains small in the AGM. RU.ee disappear from the AGM at E13.5 and from the placenta at E15.5. The liver contains RU.ee only from E11.5 onwards, the delay corresponding to the requirement for colonization. During the following days, the overall haematopoietic population in the liver amplifies considerably, so that RU.ee become twice as numerous as in the placenta. However, as seen in Fig. 3, they are significantly outgrown by the progenitor population
P1 | Caudal half or AGM | |
E10.5 | 0.103 | 0.107 |
E11 | 0.48 | 0.31 |
E11.5 | 2.5 | 2.0 |
E12.5 | 50 | 3.3 |
3 Intra-aortic haematopoiesis
In the case of the yolk sac, tissue dissociation and re-association experiments have shown that, to carry out haematopoietic activity, mesoderm must have a contact with endoderm [13–15]. In the aorta, which is the next site engaged in haematopoiesis, this activity displays a stereotyped aspect in all vertebrates studied: clusters of haematopoietic cells are appended to the aortic ventral endothelium. This unique anatomical arrangement offers an opportunity to study the origin of the cells involved and the relationship between blood cells and endothelial cells, a question that divides the community of blood and vessel specialists. Efficient experimental approaches could be carried out in vivo in the avian embryo [16–18]. Since a corpus of data had established the existence of somitic progenitors of endothelial cells, quail somites were grafted orthotopically in place of chicken somites on day 2 (E2) of development, when the aorta is represented by two distinct rudiments. As the two rudiments fuse, cells derived from the grafted-quail somitic material are seen to integrate into the roof of the aorta. From there on, these quail cells trickle down along the sides of the aorta, finally replacing the original host endothelial cells, which disappear altogether. The ventral haematopoietic cell clusters are all chicken ones in these chimeras, i.e., host in origin; as they pursue their evolution, either by entering the aortic blood flow or by migrating into the mesentery, they disappear from the ventral endothelium, and are replaced by quail cells. In other words, cells derived from splanchnopleural mesoderm compose the early aortic endothelium and they are progressively replaced by somite-derived angioblasts, when they switch their fate to haematopoiesis.
These experiments demonstrate that the blood/vascular system derives from two distinct mesodermal lineages, a ventral one, which is hemangioblastic (endowed with blood and endothelial potentials), and a dorsal one, which is purely angioblastic. The dorsal lineage is also responsible for the vascularisation of the body wall, while the viscera are vascularised by the ventral mesoderm. In the transplantation experiments, the somitic-derived endothelial cells prove incapable of entering the viscera. The haematopoietic process occurring at the expense of the primary endothelial cells is detected at a very early stage by the activation of haematopoietic specific transcription factors such as Runx1 and c-myb and the inactivation of the hemangioblastic–angioblastic specific receptor VEGF-R2 [19].
It has been possible to manipulate these distinct dorsal and ventral potentials by treating the respective mesodermal layers, either by tissue contact with tissues, or by growth factors. Ventral mesoderm associated with ectoderm for 12 h prior to grafting or cultured on a semi-solid medium containing EGF or TGFα loses the potential to invade visceral mesoderm as well as to integrate into the floor of the aorta and undergo haematopoiesis. Inversely, dorsal mesoderm associated with endoderm or treated with VEGF or TGFβ acquires this potential [16]. The signals at work in normal development still have to be worked out. In particular, it will be interesting to know how long the endodermal signal is necessary. Paradoxically, the mammalian allantois, which forms at a very early stage as gastrulation proceeds, is a purely mesodermal rudiment. In contrast, the avian allantoic bud is emitted by the caudal intestine and has the same germ layer makeup, i.e., mesoderm lined with endoderm. It is likely that a short contact with nascent endoderm occurs as gastrulation is ongoing.
4 Conclusions
The present review emphasises recent findings obtained from both avian and mammalian models. In the continuity of previous investigations, several further aspects of the early development of the haematopoietic and endothelial systems were unravelled in the avian embryo by means of in vivo experiments and were then extended to the mammalian embryo. Classical and novel features are summarized below:
- – extrinsic HSCs colonize haematopoietic organ rudiments;
- – contact with endoderm allows mesoderm to become haematopoietic; this requirement, known in the case of the yolk sac, has been extended to lateral plate mesoderm, by the identification of a dorsal angioblastic lineage and a ventral hemangioblastic lineage, the first deriving from somites and the second from lateral plate splanchnopleural mesoderm;
- – HSCs and angioblasts are clearly linked in development, since a flip flop between angioblasts and HSCs can be rather easily brought about by several growth factors. The precise signalling network responsible in vivo is not worked out; it should be pointed in this regard that the production of haematopoietic cells by the floor of the aorta depends on precise cell peregrinations: cells from the ventral lateral mesoderm migrate centripetally to form the two aortic rudiment endothelia; as the two rudiments fuse, these cells are progressively driven ventrally by the insertion of somite-derived angioblasts; they enter the haematopoietic pathway exclusively when located in the floor of the aorta. Whether this precise pattern is chronological or due to the availability of local signals in the floor is an interesting question for future analysis;
- – evidence of circulating angioblasts in the avian embryo, yielded by allantoic grafts, converges with the experimental data in the adult mouse, and points to the capacity of progenitors with hemangioblastic potential to migrate in the blood flow. However, an approach involving cross circulation between early quail and chicken embryos indicates that circulating angioblasts are rare and thus not very likely to play a significant role during normal development [20].