1 Introduction
Plectranthus forskholii (Coleus forskholii) is an important plant used in traditional ayurvedic medicine. The tuberous roots of the plant produce an active principle called forskholin. In India, it is cultivated in many parts for its carrot-like tubers, which are used as condiments and spice. The active compound forskholin is a highly oxygenated diterpene isolated by methanolic extraction from the tuberous roots of Coleus forskholii [1]. Forskholin has multifaceted pharmacognostical effects that have been linked to its role as an activator of adenylate cyclase and have shown considerable potential for its development for the treatment of glaucoma, congestive heart failure, and bronchial asthma. The production of forskholin is completely dependent on the commercial collection of wild and cultivated plants. It is reported that natural resources have been decreasing and, hence, cultivated plants are the main source of forskholin. Increasing the yield of the plants through various agricultural practices by the application of growth hormones and growth regulators have been studied extensively in various crops.
Triazole compounds such as triadimefon (TDM), propiconazole (PCZ), hexaconazole (HEX), paclobutrazol (PBZ), etc. are widely used as fungicides and they also possess varying degrees of plant growth regulating properties [2]. Triazole acts as growth regulator by regulating plant growth and development [3]. Triazole acts as a plant-growth regulator that has a profound influence on hormonal balance, photosynthetic rate, enzyme activities, lipid peroxidation, and yield components in various plants [4–11].
Triazoles inhibit cytochrome P-450-mediated oxidative demethylation reactions, which are necessary for the synthesis of ergosterol and the conversion of kaurene to kaurenoic acid in the gibberellin biosynthetic pathway, which can affect the isoprenoid pathway and alter the levels of certain plant hormones by inhibiting gibberellin synthesis, reducing ethylene evolution, and increasing cytokinin levels [3–5]. Triazole compounds have been shown to improve the yield of many root crops such as radish, tapioca and Dioscorea [3,11–13]. Previous work has also revealed increased antioxidant potentials and an enhancement in alkaloid production under TDM application in Catharanthus roseus [7].
Triazoles affect the activities of several enzymes, especially those related to the detoxification of active oxygen species and antioxidant metabolism [2,9,10]. Especially in tuber crops, it was reported that triazoles influence root growth, although their effect may be either inhibitory or stimulatory, depending on the plant and the concentration of the triazole compound used [11]. The present work has been undertaken to assess the stimulatory or inhibitory action of different concentrations of hexaconazole on the growth and yield of Coleus forskholii.
2 Materials and methods
2.1 Plant materials, cultivation and hexaconazole treatments
The study was conducted in the Botanical Garden, Department of Botany, Annamalai University, Annamalainagar. A local cultivar of Coleus forskholii was utilized for the present study. The field was brought to a fine tilth by giving three ploughings. The uniform length of 10-cm herbaceous terminal cuttings was prepared from the nursery. The uniform sized cuttings were planted in the field at a spacing of 30 × 30 cm. Life irrigation was given on the third day after planting (DAP). Then the field was irrigated at weekly intervals and timely weeding was done. Hexaconazole of different concentrations such as 10, 15, 20, 25, and 30 mg L−1 was used by soil drenching on the 60th, 90th, 120th, and 150th DAP.
The experiment was laid out in a completely randomized block design, with seven replications. Observations were recorded on morphological and tuber characters on 165 DAP. The numbers of branches, stem length, leaf area, and fresh and dry matter content of shoot have been studied. The numbers of tubers, length, and girth of tubers and fresh and dry weight of tubers have also been studied.
2.2 Morphological parameters
Morphological parameters, like fresh weight (FW) and DW and also yield parameters were measured from the samples. The total leaf area was calculated with a LICOR photoelectric area meter (model L1-3100, Lincoln, USA).
2.3 Pigment composition
2.3.1 Chlorophyll and carotenoid contents
Chlorophyll and carotenoid were extracted from the leaves and estimated according to the method of Arnon [14]. Carotenoid content was calculated using the formula of Kirk and Allen [15] and expressed in mg g−1 fresh weight.
2.3.2 Anthocyanin
Anthocyanin was extracted and estimated by the method of Kim et al. [16]. Five hundred milligrams of fresh tissue taken from the third leaf and from the periphery of the tuber tissue (0.5 cm from the epidermis and 1 cm from head of the root tuber) were ground with a pestle in a mortar under liquid nitrogen, and extracted overnight with 20 ml of 50% acetic acid. The homogenate was centrifuged at 19,000 g for 15 min. The resultant supernatant was made up to 20 ml; 80 ml of McIIvaine's buffer (pH 3.0) were added, and the absorption was measured at 530 nm by means of a U-2001 Hitachi spectrophotometer.
2.3.3 Xanthophyll
Xanthophyll contents were estimated by the method of Neogy et al. [17]. Five hundred milligrams of fresh tissues taken from the third leaf and the periphery of the tuber were used for the assay. The tissue was ground with 10 ml of 80% acetone at 4 °C with a pestle in a mortar, and centrifuged at 1000 rpm for 15 min. The residue was re-extracted with 80% acetone until the colour completely disappeared in the residue. The aqueous acetone extract was shaken thrice with an equal volume of hexane in a separating funnel and the combined hexane fractions were washed with equal volumes of water. To separate xanthophylls from carotenes, the hexane fraction containing carotenoids was extracted repeatedly with 90% methanol. The absorbance of the methanol fraction containing xanthophylls was measured at 450 nm in a spectrophotometer.
2.4 Non-enzymatic antioxidant estimations
2.4.1 Ascorbic acid content
Ascorbic acid (AA) content was assayed as described by Omaye et al. [18]. The extract was prepared by grinding 1 g of fresh material with 5 ml of 10% TCA, centrifuged at 3500 rpm for 20 min, re-extracted twice; the supernatant was made up to 10 ml and used for the assay. To 0.5 ml of extract, 1 ml of DTC reagent (2,4-dinitrophenyl hydrazine-thiourea-CuSO4 reagent) was added, incubated at 37 °C for 3 h and 0.75 ml of ice-cold 65% H2SO4 was added, allowed to stand at 30 °C for 30 min. The resulting colour was read at 520 nm in a U-2001 Hitachi spectrophotometer. The AA content was determined using a standard curve prepared with AA.
2.4.2 Reduced glutathione
The reduced glutathione (GSH) content was assayed as described by Griffith and Meister [19]. Two hundred milligrams of fresh material were ground with 2 ml of 2% metaphosphoric acid and centrifuged at 17,000 rpm for 10 min. Addition of 0.6 ml of a 10% sodium citrate solution neutralized the supernatant. One millilitre of assay mixture was prepared by adding 100 μl of extract, 100 μl of distilled water, 100 μl of 5,5-dithio-bis-(2-nitrobenzoic acid) and 700 μl of NADPH. The mixture was stabilized at 25 °C for 3–4 min. Then 10 μl of glutathione reductase were added, the absorbance and the GSH contents were measured at 412 nm in the spectrophotometer.
2.4.3 Total phenols
Total phenol amounts were estimated by the method of Malick and Singh [20]. Five hundred milligrams of fresh plant tissue were ground with a pestle in a mortar with 10 ml of 80% ethanol. The homogenate was centrifuged at 10,000 rpm for 20 min. The supernatant was evaporated to dryness. The residue was dissolved with 5 ml of distilled water and used as extract. To 2 ml of the extract, 0.5 ml of Folin–Ciocalteau reagent was added. After 3 min, 2 ml of 20% Na2CO3 solution was mixed thoroughly. The mixture was kept in boiling water for exactly one minute and the absorbance was measured at 650 nm after cooling. The total phenol was determined using a standard curve prepared with different concentration of gallic acid.
3 Results and discussion
3.1 Effects of different concentrations of hexaconazole on growth parameters
The plant height was reduced in all the concentrations of hexaconazole used. However, the reduction in shoot length was more significant with a hexaconazole concentration of 25 mg L−1 compared to the other concentrations used (Table 1). Similar findings have been reported in various plants. Triazoles have a pronounced effect on the reduction in the plant height [21]. This reduction in shoot growth by triazoles occurs primarily as a consequence of reduced internodes elongation, and the effective dose varies according to species and cultivars [9,12,13].
Variations in the morphological parameters of Plectranthus forskholii with increasing concentrations of hexaconazole. Values are given as mean of seven samples in each group
Parameters | Hexaconazole concentrations | |||||
0 mg L−1 | 10 mg L−1 | 15 mg L−1 | 20 mg L−1 | 25 mg L−1 | 30 mg L−1 | |
Stem length (cm) | 56.96 | 54.0 | 51.5 | 48.11 | 45.74 | 43.15 |
Leaf area (cm2/plant) | 4455.96 | 4028.32 | 3837.41 | 3678.52 | 3348.91 | 3297.15 |
No. of branches/plant | 99.28 | 103.79 | 106.99 | 110.29 | 119.01 | 112.31 |
FW of shoot (g/plant) | 629.79 | 685.32 | 710.16 | 731.75 | 764.54 | 749.59 |
DW of shoot (g/plant) | 171.13 | 196.27 | 212.15 | 226.34 | 251.29 | 240.08 |
No. of tubers/plant | 18.15 | 23.11 | 29.12 | 36.19 | 44.11 | 40.14 |
Length of tubers (cm) | 15.04 | 13.06 | 12.17 | 11.05 | 10.03 | 9.48 |
Diameter of tuber (cm) | 1.48 | 1.87 | 1.96 | 2.44 | 3.16 | 2.89 |
FW of tuber (g/plant) | 252.14 | 293.14 | 348.69 | 412.60 | 512.03 | 491.20 |
DW of tuber (g/plant) | 38.6 | 47.15 | 67.69 | 85.54 | 101.22 | 98.06 |
The mean shoot length showed a downward trend, but the number of side branches increased in treated plants. The leaf area decreased in treated plants (Table 1). Triazoles have several morphological effects on leaves. They reduce leaf area, but increase epicuticular wax, width, and thickness [22]. Though the leaf area was decreased in hexaconazole-treated plants, the leaf dry weight increased. The observed increase in the dry weight of hexaconazole-treated plants leaves may be due to the formation of additional layers by palisade mesophyll. In wheat and Chrysanthemum, triazoles increased the thickness of leaves by inducing additional layers of palisade mesophyll cells [23]. Besides, in radish, triadimefon induces the formation of sun-type chloroplasts, which allows higher photosynthetic quanta conversion, and this effect is probably related to increased cytokinin levels [24]. This effect of triazole may increase dry matter accumulation, even though leaf area is reduced, as it was observed in this experiment.
The reduction in shoot growth after 120th DAP both in control and treated plants observed in this study could be attributed to the senescence of shoot during the final phase of growth. The reduced foliage weight observed after 120 days of growth was more pronounced in control than in hexaconazole-treated plants. Because hexaconazole treatment induced a transient rise in ABA levels, which makes the leaf darker green, possibly because of an increase in chlorophyll content per unit leaf area [21]. A similar result was observed in bean plant, i.e. the triazole induced a transient rise in ABA levels [25] and in Catharanthus plants under salt stress [26].
The number of roots showed a progressive increase in treated plants, and the highest number of roots was observed for a hexaconazole concentration of 25 mg L−1. The fresh and dry weight of tuber increased in treated plants. Nevertheless, the higher number of tubers in treated plants was recorded for a 25 mg L−1 concentration of hexaconazole (Table 1). The increase in the tuber yield-to-weight ratio after initiation in treated plants depended on the ability of aerial parts to synthesize the needed assimilates for the rapid development of tubers, which act as ‘sinks’. Increased root growth was previously reported in Catharanthus plants under plant-growth regulator treatments [27]. Triazoles are known to shift assimilate partitioning from leaves to roots, and they could also alter mineral uptake and plant nutrition [28].
There was an increase in the mean length and girth of tubers in hexaconazole-treated plants compared to untreated plants (Table 1). Paclobutrazol treatment of primary pea roots inhibited root extension, but promoted radial cell expansion [29]. Increased root diameter has been correlated with larger cortical parenchyma cells in soybean and maize [30]. This increased length and girth of tubers could be attributed to an increase in cytokinin levels. The enhanced levels of cytokinin found in the roots of soybean seedlings after treatment with various retardants could be due either to a stimulated synthesis of cytokinins, or to transport of cytokinins to the shoot, or to prevention of degradation of cytokinins.
3.2 Effects of different concentrations of hexaconazole on pigment content
Triazole-treated plants typically appear dark greener and this has been correlated with an increase of the chlorophyll content in Plectranthus (Fig. 1). The increased chlorophyll content was also attributed to more densely packed chloroplasts in a small leaf area as reported in maize [31]. Increased chlorophyll synthesis was observed with triazole treatment in Cucumis cotyledons [32] paclobutrazol treated plants [33]. It was also been reported that triadimefon has a cytokinin-like activity with antisenescence properties in cucumber seedlings [34].
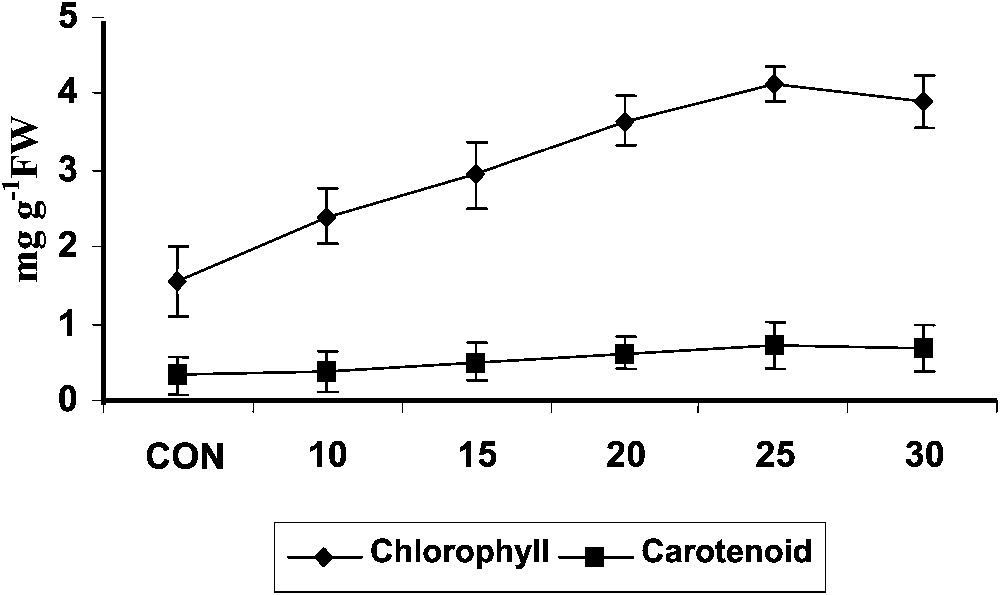
Variations in the total chlorophyll and carotenoid contents of Plectranthus forskholii with increasing concentrations of hexaconazole. Values are given as mean ± SD of seven samples in each group.
Hexaconazole treatment increased the carotenoid content (Fig. 1) in Plectranthus. Triadimefon treatment induced higher levels of carotenoid in cucumber cotyledons [34] and Catharanthus roseus [6]. Similar results were observed in uniconazole- and paclobutrazol-treated wheat seedlings [33]. Increased level of cytokinin, particularly transzeatin and its riboside, has been reported in sunflower cell suspension, rice, soybean and rape seedlings after uniconazole treatment, and thus increased amounts of zeatin might be responsible for the increased synthesis of carotenoid in the plants [35].
Hexaconazole treatment increased the anthocyanin content (Fig. 2) to a larger extent in Plectranthus. Triadimefon increased the chlorophyll and anthocyanin contents in radish cotyledons, and its effects can be compared to that produced by cytokinin [6]. Treatment with ABA increased anthocyanin accumulation in strawberry fruits [36]. Triazoles induced a transient raise in abscisic acid content in bean. This increased ABA content induced by triazole might be the cause for the increased anthocyanin content.
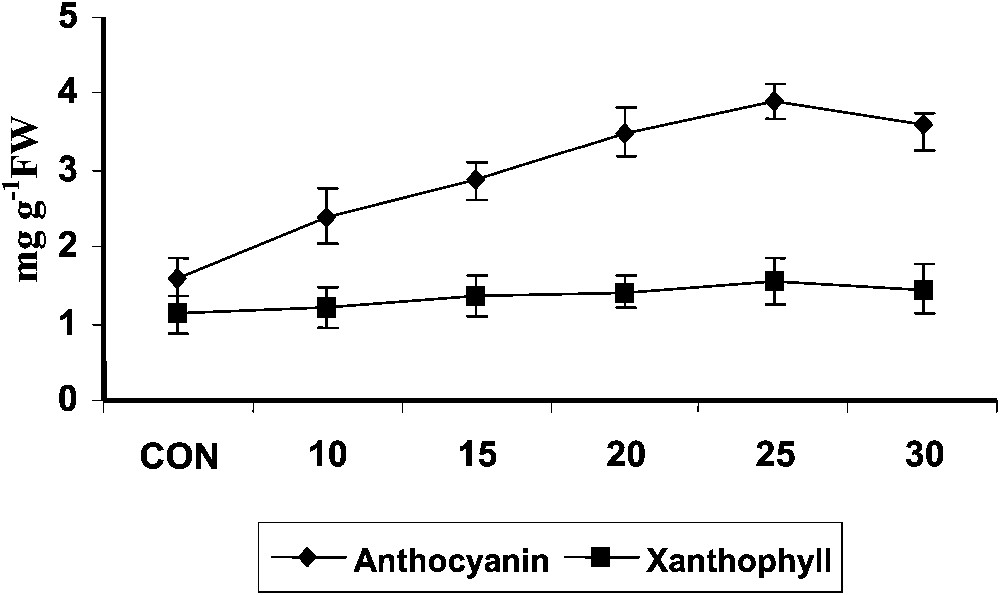
Variations in the anthocyanins and xanthophylls contents of Plectranthus forskholii with increasing concentrations of hexaconazole. Values are given as mean ± SD of seven samples in each group.
Hexaconazole treatment increased xanthophyll content at all stages of growth in Plectranthus (Fig. 2). Xanthophyll participates in light harvesting photosynthetic membranes and protects the photosynthetic apparatus from excessive light energy by quenching chlorophylls and singlet oxygen. Triadimefon treatment increased the chlorophyll, carotenoid, and xanthophyll content in the leaves of cucumber [34].
3.3 Effects of different concentrations of hexaconazole on the ascorbic acid content
The ascorbic acid content of the shoot tissue increased with age in the treated and control plants. Treatment with hexaconazole significantly increased the ascorbic acid contents of shoot as compared to control (Fig. 3). Hexaconazole treatments increased the ascorbic acid content in the tuber tissue of Plectranthus to a significant extent when compared to control. Hexaconazole-treated shoot tissue recorded higher ascorbic acid content than that measured in tuber tissue. Triadimefon [7], drought [37] and salt-stress [38,39] treatments increased the ascorbic acid content in Catharanthus plants. Ascorbic acid is an important component of the plant antioxidant system [40]. Ascorbate is one of the two major soluble antioxidants in chloroplast [41]. Ascorbate has a photoprotective function because of its antioxidant capacity. Paclobutrazol increased the level of antioxidants like ascorbic acid and α-tocopherol, like in Arachis hypogaea, and protected the membrane by preventing or reducing oxidative damage [42]. An increase in the ascorbic acid content was reported in the paclobutrazol-treated Citrus limon [43].
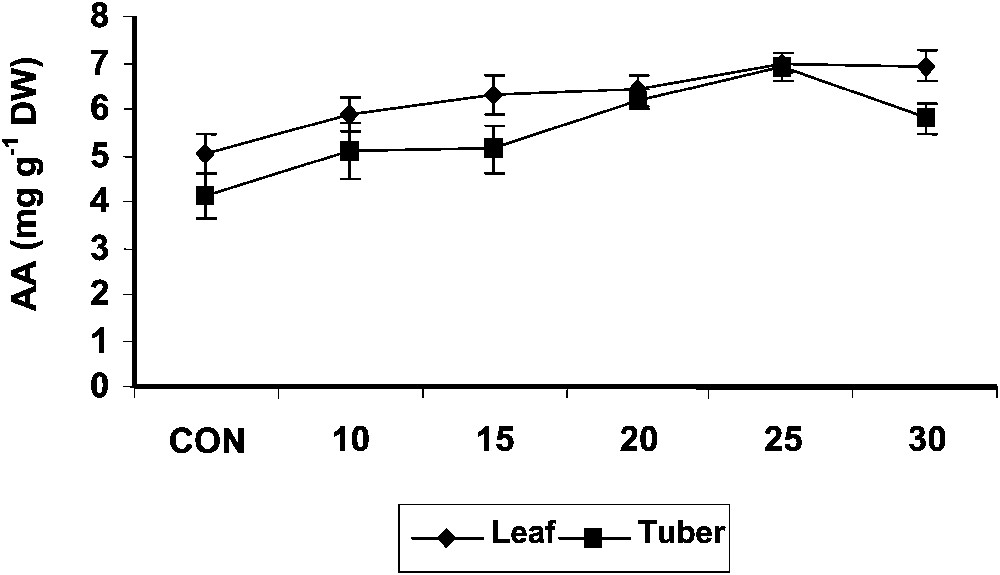
Variations in the ascorbic acid contents of Plectranthus forskholii with increasing concentrations of hexaconazole. Values are given as mean ± SD of seven samples in each group.
3.4 Effects of different concentrations of hexaconazole on reduced glutathione content
The reduced glutathione content was higher in hexaconazole-treated Plectranthus plants as compared to control. In tuber tissues also, the reduced glutathione content increased with hexaconazole treatment (Fig. 4) and there was not significant difference between the treatments. Similar results were reported in paclobutrazol [9] and gibberellic acid [44] treatments in Catharanthus roseus plants. Glutathione has been shown to regulate the expression of genes whose products are involved in redox regulation and enhancement of stress tolerance [45]. Enhanced reduced glutathione levels along with the interaction of ascorbic acid and tocopherol may increase the oxidative-stress tolerance.
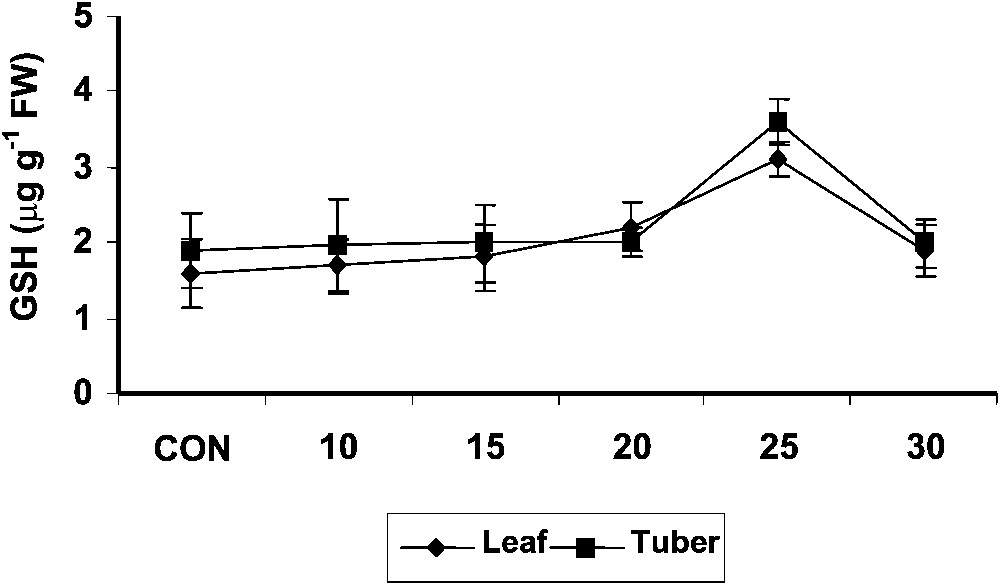
Variations in the reduced glutathione contents of Plectranthus forskholii with increasing concentrations of hexaconazole. Values are given as mean ± SD of seven samples in each group.
3.5 Effects of different concentrations of hexaconazole on total phenol content
The total phenol content (Fig. 5) in shoot increased with increase of age in both control and hexaconazole-treated Plectranthus plants. Hexaconazole treatment increased the total phenol content in tuber tissue when compared to control. The phenol content was higher in shoot than that in the tuber. It has been suggested that peroxidase could act as an efficient H2O2-scavenging system in plant vacuoles in the presence of phenolics and reduced ascorbate [46]. Sgherri et al. [47] hypothesized a cycle where H2O2 is scavenged by phenolic compounds. Phenolics are oxidized to phenoxyl radicals. These phenoxyl radicals reduce the ascorbic acid into monodehydroascorbate. Thus, phenols act as intermediary ROS acceptors in the vacuoles. In the present study, the total phenol content significantly increased with hexaconazole treatment both in shoot and tuber at all stages of growth. This increase of phenols by hexaconazole treatment may further enhance the antioxidant capacity of Plectranthus along with other antioxidants.
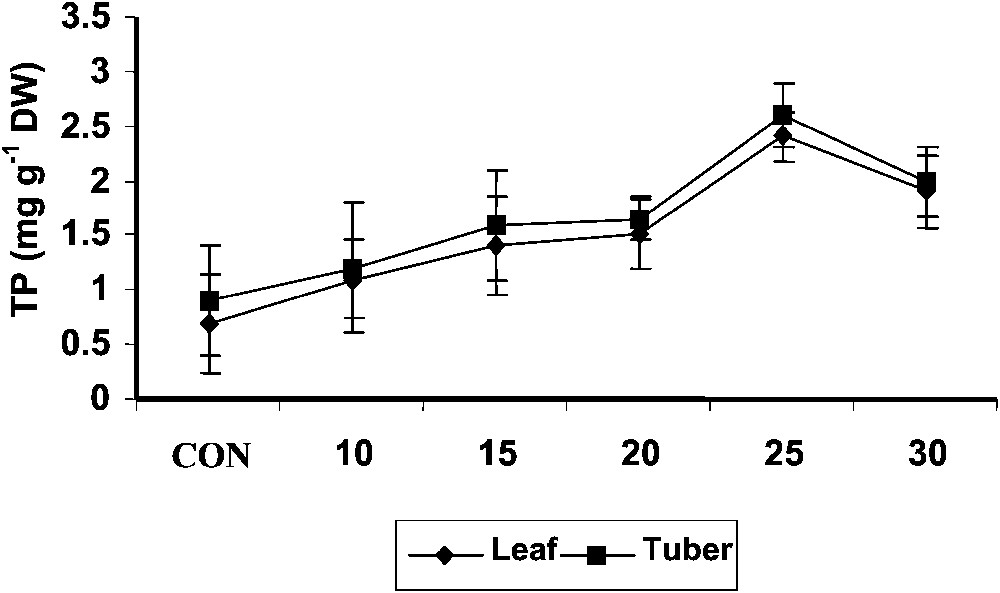
Variations in the total phenol contents of Plectranthus forskholii with increasing concentrations of hexaconazole. Values are given as mean ± SD of seven samples in each group.
4 Conclusion
A significant variation was observed in shoot length, leaf area, stem and leaf fresh and dry weight, number of tubers, length, girth and fresh and dry weight of tubers in treated Plectranthus plants compared to control plants. The variations of growth and yield components are more significant in treated Plectranthus plants for a hexaconazole concentration of 25 mg L−1 than in all those subjected to other concentrations of hexaconazole in this study.