1 Introduction
Triazole compounds are mostly used as systemic fungicides. Triazole compounds, like triadimefon (TDM), propiconazole (PRO), etc., have plant growth regulating properties and induce many morphological changes, like reduction in shoot elongation, stimulation of rooting, inhibition of gibberellin synthesis, increase of chlorophyll content, alteration of the carbohydrate status, and increase of cytokinin synthesis [1]. Triazole compounds have been shown to improve the yield of many root crops, such as carrot, sugar beet, Chinese potato, and tapioca [2,3]. Triazole compounds influences hormone balances, photosynthetic rate, enzyme activities and yield components in crop plants [3–5].
Triazole inhibits cytochrome P-450 mediated oxidative dimethylation reaction, including those that are necessary for the synthesis of ergosterol and the conversion of kaurene to kaurenoic acid in the gibberellin biosynthetic pathway. The plant growth regulating properties of triazoles are mediated by their ability to alter the balance of important plant hormones, including gibberellic acid, ABA, and cytokinins [1,6]. They also inhibit gibberellin and ergosterol biosynthesis in plants and fungi, respectively [7]. The triazole compounds confer protection against various environmental stresses in plants [8–13].
Lipid peroxidation (LPO) can be initiated enzymatically by lipoxygenases and this enzyme incorporates molecular oxygen into linoleic and linolenic acids, to form lipid hydroperoxides [14]. Compatible solutes accumulation in the cytoplasm is considered as a mechanism contributing to stress tolerance [15]. Compatible osmotica such as proline (PRO) and glycine betaine (GB) are thought to function as osmoprotectants for proteins [16]. PRO and GB accumulation provide an environment compatible with macromolecular structure and function, and help plants to adapt to salinity injury [17–19]. Two enzymes play an important role in controlling the level of PRO, viz., proline oxidase (PROX), which catalyzes the conversion of PRO to glutamate, and γ-glutamyl kinase (γ-GK), which plays an important role in PRO synthesis [17]. Though the previous works demonstrated the effects of triazoles on agricultural crops [3,4,8,11–13,15,20], this is not the case in medicinal plants.
Catharanthus roseus (L.) G. Don. (Madagascar periwinkle), one of the highly exploited and studied medicinal plants, belongs to the family Apocynaceae. C. roseus is a perennial tropical plant that produces more than 100 monoterpenoid indole alkaloids (MIAs) including two commercially important cytotoxic dimeric alkaloids used in cancer chemotherapy [21]. All parts of the plant are rich in alkaloids, with maximum concentrations found in the root bark, particularly during flowering. An infusion of the leaves is used to treat menorrhagia. The juice of the leaves is applied externally to relieve wasp stings. All parts of the plant are credited with hypoglycaemic properties and are used to treat diabetes [22]. Many works have already been carried out on this plant because of its medicinal importance [23] concerning growth-regulator effects [24,25], but effects of fungicides on this medicinal plant attracted little attention [26,27]. To the best of our knowledge, no information about the effect of TDM in LPO, EL, GB, and PRO metabolism in this medicinal plant is available. This investigation was aimed at finding out the extent of changes in LPO, EL, GB and PRO contents, PRO synthesizing (γ-GK), and PRO degrading (PROX) enzyme activities in C. roseus under TDM treatment.
2 Materials and methods
2.1 Plant materials and cultivation methods
The seeds of Catharanthus roseus (L.) G. Don. were collected from J.P. Laboratories, Rajapalayam, Tamil Nadu, India. In an attempt to remove germination inhibitors, the seeds were leached with distilled water for five days before the experiment. Seeds were then surface sterilized in an aqueous solution of 0.1% HgCl2 for 60 s to prevent fungal attack, and rinsed in several changes of sterile water.
The triazole compound TDM [1-(4-chlorophenoxy)-3,3-dimethyl-1-(1H-1,2,4-triazolyl)-2-butanone] as Bayleton-25 WP was obtained from Bayer (India) Ltd., Mumbai. During the study, the average temperature was 32/26 °C (maximum/minimum), and the relative humidity (RH) varied between 60 and 75%. The experiments were carried out in the Botanical Garden and Stress Physiology Lab, Department of Botany, Annamalai University, Tamil Nadu, India.
The plants were grown in the Botanical Garden between September and December 2006. The seeds were sown separately in raised seedbeds by broadcasting method and covered with fine soil to ensure proper germination. The nursery beds were watered twice a day and weeded regularly in order to ensure healthy growth of the seedlings. The land was repeatedly ploughed and brought to fine tilth and divided into two plots prior to transplantation. Two plots were prepared; forty plants per plot were planted at a distance of 30 × 45 cm and irrigated immediately for better establishment. Subsequent irrigation was done two times a week to keep an optimum moisture level in the soil.
2.2 TDM treatment
One plot was subjected to TDM treatment and another one was kept as control. TDM @ 15 mg l−1 was given to each plant by soil drenching. The treatment was administered during 53, 68, and 83 days after planting (DAP). The plants were uprooted randomly on 60, 75, and 90 DAP, and separated into root, stem, and leaves, and used for estimating the LPO, EL, GB and PRO contents, as well as the PRO-synthesizing (γ-GK) and PRO-degrading (PROX) enzyme activities.
2.3 Estimation of the H2O2 content
H2O2 content was determined according to Velikova et al. [28]. 0.5 g of fresh plant material was homogenized in an ice bath with 5 ml of 0.1% (w/v) trichloro acetic acid (TCA). The homogenate was centrifuged at 12,000 rpm for 15 min and 0.5 ml of the supernatant was added to 0.5 ml of 10 mM potassium phosphate buffer (pH 7.0) and 1 ml of 1 M Potassium iodide (KI). The absorbance of the supernatant was measured at 390 nm in a spectrophotometer (U-2001-Hitachi). The content of H2O2 was calculated by comparison with a standard calibration curve previously made by using different concentration of H2O2.
2.4 Estimation of lipid peroxidation (TBARS content)
LPO was estimated as TBARS [29]. Fresh sample (0.5 g) were homogenized in 10 ml of 0.1% TCA, and homogenate was centrifuged at 15,000 rpm for 15 min. To a 1.0-ml aliquot of the supernatant, 4.0 ml of 0.5% thiobarbituric acid (TBA) in 20% TCA were added. The mixture was heated at 95 °C for 30 min in the laboratory's electric oven and then cooled in an ice bath. After centrifugation at 10,000 rpm for 10 min, the absorbance of the supernatant was recorded at 532 nm. The TBARS content was calculated according to its extinction coefficient of 155 mM−1 cm−1 and expressed in units (U). One U is defined as one micromole of MDA formed per minute and per milligram of protein.
2.5 Estimation of electrolyte leakage (EL)
One gram of tissue was cut into 2-cm segments, rinsed in deionised water to remove the contents of cut cells, and placed in test tubes containing 15 ml of bidistilled water for 24 h at 24 °C. The electrical conductivity of the solution in the tube was determined using a conductance meter. The tubes were placed in a boiling-water bath for 20 min, and then cooled to 24 °C; the electrical conductivity was then measured. The percentage of leakage of the tissue was calculated as the ratio of the conductivity after 12 h to the conductivity after boiling (total ionic conductivity) [30].
2.6 Estimation of the proline content
The PRO content was estimated by the method of Bates et al. [31]. The plant material was homogenized in 3% aqueous sulfosalicylic acid, and the homogenate was centrifuged at 10,000 rpm. The supernatant was used for the estimation of the PRO content. The reaction mixture consisted of 2 ml of acid ninhydrin and 2 ml of glacial acetic acid, which was kept boiling at 100 °C for 1 h. The reaction was stopped by cooling in an ice bath, and the reaction mixture was extracted with 4 ml of toluene; the absorbance was read at 520 nm.
2.7 Estimation of the glycine betaine content
The amount of GB was estimated according to the method of Grieve and Grattan [32]. The plant tissue was finely ground, mechanically shaken with 20 ml of demonized water for 24 h at 25 °C. The samples were then filtered and filtrates were diluted 1:1 with 2 N H2SO4. Aliquots were kept in centrifuge tubes, and cooled in icy water for 1 h. A cold KI–I2 reagent was added and the reactants were gently stirred with a vortex mixture. The tubes were stored at 4 °C for 16 h and then centrifuged at 10,000 rpm for 15 min at 0 °C. The supernatant was carefully aspirated with a fine glass tube. The periodide crystals were dissolved in 9 ml of 1,2-dichloroethane. After 2 h, the absorbance was measured at 365 nm using GB as standard and expressed in mg g−1 DW.
2.8 Estimation of the protein content
The soluble protein was extracted and estimated following the method of Bradford [33] using bovine serum albumin (BSA, Sigma, USA) as the standard and expressed in mg g−1 dry weight (DW).
2.9 Estimation of amino acid content
Extraction and estimation of the AA content was followed by the method of Moore and Stein [34]. 0.5 gram of plant material was homogenised with 10 ml of 80% boiling ethanol. The extract was centrifuged at 800 g for 15 min and the supernatant was made up to 10 ml with 80% ethanol and used for the estimation of free AAs. One millilitre of ethanol extract was poured in a 25-ml test tube and neutralized with 0.1 N sodium hydroxide using methyl red indicator. One millilitre of ninhydrin reagent was then added. The content was boiled in a boiling water bath for 20 min then 5 ml of diluting reagent were added, cooled, and diluted to 25 ml with distilled water. The absorbance was read at 570 nm in a spectrophotometer. The AA content was calculated using a calibration curve, with glycine as standard. The results were expressed in milligrams per gram of dry weight.
2.10 γ-Glutamyl kinase [ATP: L-glutamate 5-phosphotransferases (EC 2.7.2.11)] activity
γ-GK activity was assayed by the method of Hayzer and Leisinger [35]. Plant samples (1 g) were extracted with 50 mM Tris-HCl buffer and centrifuge at 40,000 g for 30 min at 4 °C. To 0.1 ml of the reaction buffer added with 0.1 ml of 50 mM ATP and 1.8 ml of extract and incubated at 37 °C for 30 min, 2 ml of stop buffer was added. The γ-GK activity was measured at 535 nm and expressed in units (U mg−1 protein). One unit (U) of enzyme activity is defined as μg of γ-glutamylhydroxamate formed per minute per milligram of protein.
2.11 Proline oxidase [L. proline: O2 oxidoreductase (EC 1.4.3.1)] activity
The PROX activity was determined according to the method outlined by Huang and Cavalieri [36]. Plant samples (1 g) were extracted with 5 ml of Tris-HCl buffer pH 8.5 and centrifuged at 10,000 g for 10 min at 4 °C. The supernatant was again centrifuged at 25,000 g at 20 min at 4 °C. Three millilitres of assay mixture were prepared by taking 0.1 ml of extract, 1.2 ml of 50 mM Tris HCl buffer pH 8.5, 1.2 ml of 5 mM MgCl2, 0.1 ml of 0.5 mM NADP, 0.1 ml of 1 mM KCN, 0.1 ml of 1 mM phenazine methosulphate (PMS), 0.1 ml of 0.06 mM 2,6-dichlorophenol indophenol (DCPIP), 0.1 ml distilled water instead of PRO. The reaction was monitored at 600 nm at 25 °C using PRO to initiate reaction; the increase of the OD value was noted after 0, 1, 2, 3, 4 and 5 min. PROX activity was expressed in U mg−1 protein (one U = mM DCPIP reduced min−1 mg−1 protein).
For all enzyme assays, enzyme protein was determined by the method of Bradford [33]. Each treatment was analyzed with at least seven replicates and a standard deviation (SD) was calculated; data are expressed in mean ± SD of seven replicates.
3 Results and discussion
3.1 Effect of TDM on H2O2 content, lipid peroxidation, and electrolyte leakage
The triazole treatment increased the H2O2 production considerably in leaf, stem, and root of C. roseus plants (Fig. 1a). Oxidative damage to tissue lipid was estimated by the content of total TBARS. The TDM plants showed a lower level of LPO in C. roseus when compared to control (Fig. 1b). Treatment with TDM significantly inhibited the EL in the leaf, stem, and root tissues of C. roseus (Fig. 1c).
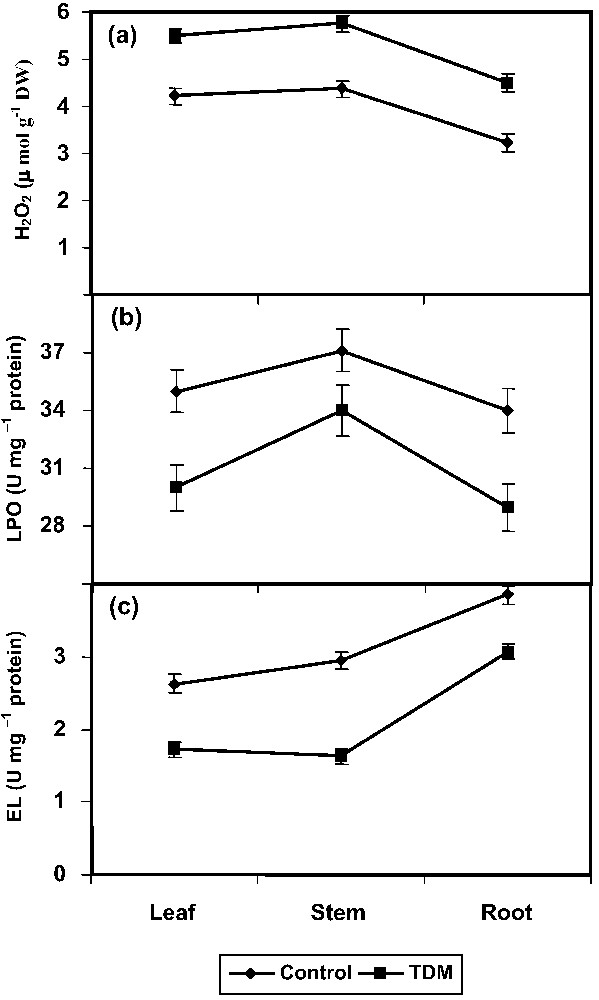
Effect of triadimefon on H2O2 (a), lipid peroxidation (b), and electrolyte leakage (c) of Catharanthus roseus. Values are given as means ± SD of six experiments in each group.
H2O2 is toxic to the plants. Reactive oxygen species (ROS) causes damage to lipids, proteins, and DNA. Peroxidation of membrane lipids occurs when ROS react with unsaturated fatty acids, which leads to leakage of cellular contents, rapid desiccation, and cell death. The ability of plant tissues to mobilize enzymatic defence against uncontrolled lipid peroxidation may be an important feature of their tolerance [28]. Triazole-treated carrot plants had lower level and slower accumulation rate of H2O2 [37]. The triazole compounds enhanced different H2O2 scavenging enzymes, like ascorbate peroxidase and catalase, and also various antioxidants in C. roseus [27]. This enhancement would have helped in scavenging of ROS like H2O2. Triazole compounds not only protect plants from stress, but also induce stress-like symptoms [27]; this might be the reason for increasing the H2O2 content in different parts of C. roseus. The decrease in H2O2 in light stressed pea plants was related to the increase in the antioxidant enzymes [38].
LPO is a measure of the injury to the membrane. LPO is often used as an indicator of oxidative damage. LPO is measured by the TBARS released, which is a consequence of higher oxidative stress [39]. TBARS, the cytotoxic product of lipid peroxidation, are normally considered as the major TBA-reacting compounds that indicate the magnitude of the oxidative stress [40]. Herbicides are known to generate activated oxygen species, which are likely to contribute to the toxic effects of these herbicides [41]. Uniconazole reduced the EL and MDA accumulation and consequently decreased heat induced LPO in rape plants [42]. Similar results were observed in triazole treated Egeria densa leaves [43] and paclobutrazol treated wheat seedlings [44]. LPO has been associated with damages provoked by a variety of environmental stresses. Polyunsaturated fatty acids (PUFA) are the main membrane lipid components susceptible to peroxidation and degradation [14].
Changes in membrane leakage and injury can be measured by the extent of EL in tissues [45]. Triazole treatments inhibited the EL in carrot [37]. Paclobutrazol-treated wheat seedlings maintained a high degree of membrane integrity under heat stress [46] and uniconazole treatment inhibited the EL in soybean [47]. Paclobutrazol altered the membrane properties and facilitated the removal of damaged areas in the membranes of maize [48]. Disruption of cell membrane integrity is an inherent feature of senescence in plants, as observed in wheat leaves [1]. Triazole altered the sterol biosynthesis and changed the composition of sterol in the plasma membrane [49]. This change in sterol composition may induce changes in cell membrane that may be reflected in increased membrane stability, acclimatization, and frost hardiness, as observed in white spruce [50].
3.2 Effect of TDM on the proline and glycine betaine contents
The TDM treatment also increased the proline content in the leaves, stem and root of C. roseus plants (Fig. 2a). Treatment with TDM and uniconazole significantly increased the free proline content in mulberry [51]. Similarly, paclobutrazol increased the proline content in Eruca sativa seedlings [52]. ABA increased the proline content in Phaseolus vulgaris [53]. Triazole induced a transient raise in ABA content and this raise in ABA by triadimefon and hexaconazole might increase the amino acid and proline contents in C. roseus. An understanding the biosynthesis, degradation, transport, roles of PRO during stress and the signalling events that regulate stress–induced accumulation is vital in developing plants for stress tolerance [54].
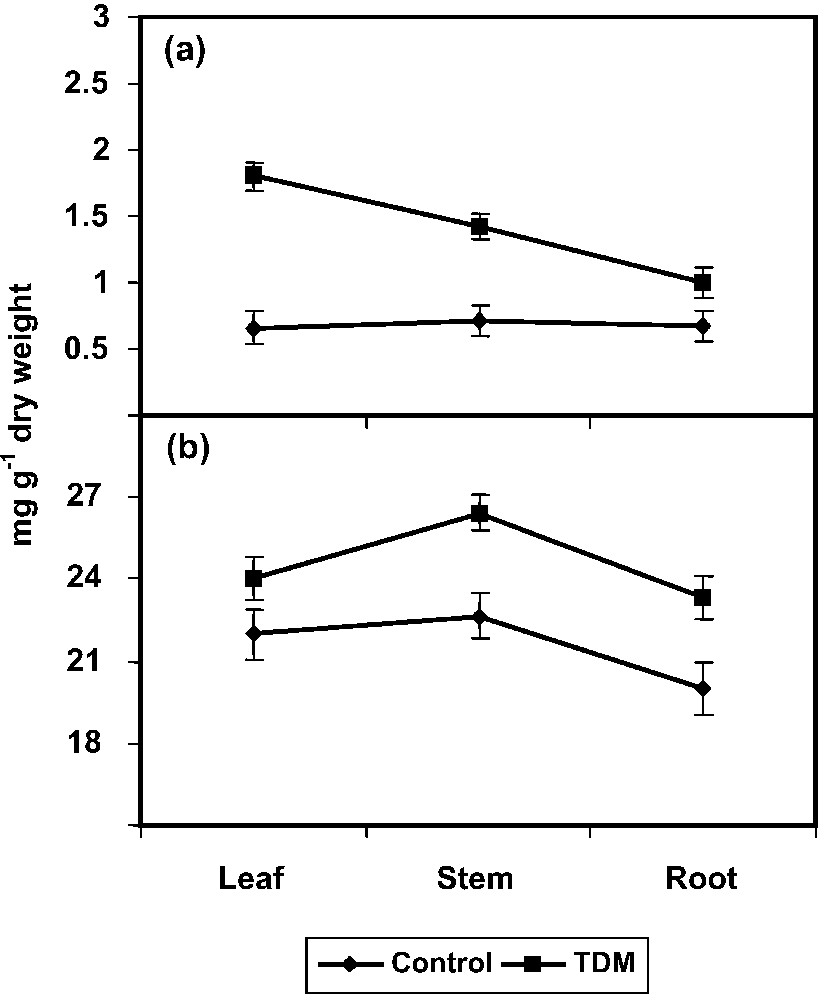
Effect of triadimefon on proline (a), glycine betaine (b) contents of Catharanthus roseus. Values are given as means ± SD of six experiments in each group.
One of the most important mechanisms exerted by higher plants under stress conditions is the accumulation of compatible solutes such as GB. We noticed a gradual increase in the GB content under TDM treatment in C. roseus plants (Fig. 2b). GB accumulation results from the oxidative stress induced by the fungicide application; it is helpful in the stimulation of tolerance mechanisms [17,55].
3.3 Effect of TDM on the protein and amino acid contents
TDM treatment increased the protein content to a significant level in all parts (leaf, stem, and root) of C. roseus plants compared to control (Fig. 3a). The increase in the protein content has been previously described in Echinochloa furmentacea [56] and in Brassica carinata [57] plants treated with paclobutrazol and uniconazole, respectively The amino acid content increased to a higher extent in the leaves, stem, and root of C. roseus plants with TDM treatment (Fig. 3b). Similar results were observed in uniconazole-treated Phaseolus vulgaris [53], and penconazole induced a moderate increase in amino acid in higher plants [43]. Plants respond to a variety of stresses by accumulating certain specific metabolites like amino acids [17,54]. It may perhaps provide extra protection to plants against oxygen radical damage arisen from abiotic stresses [18,19].
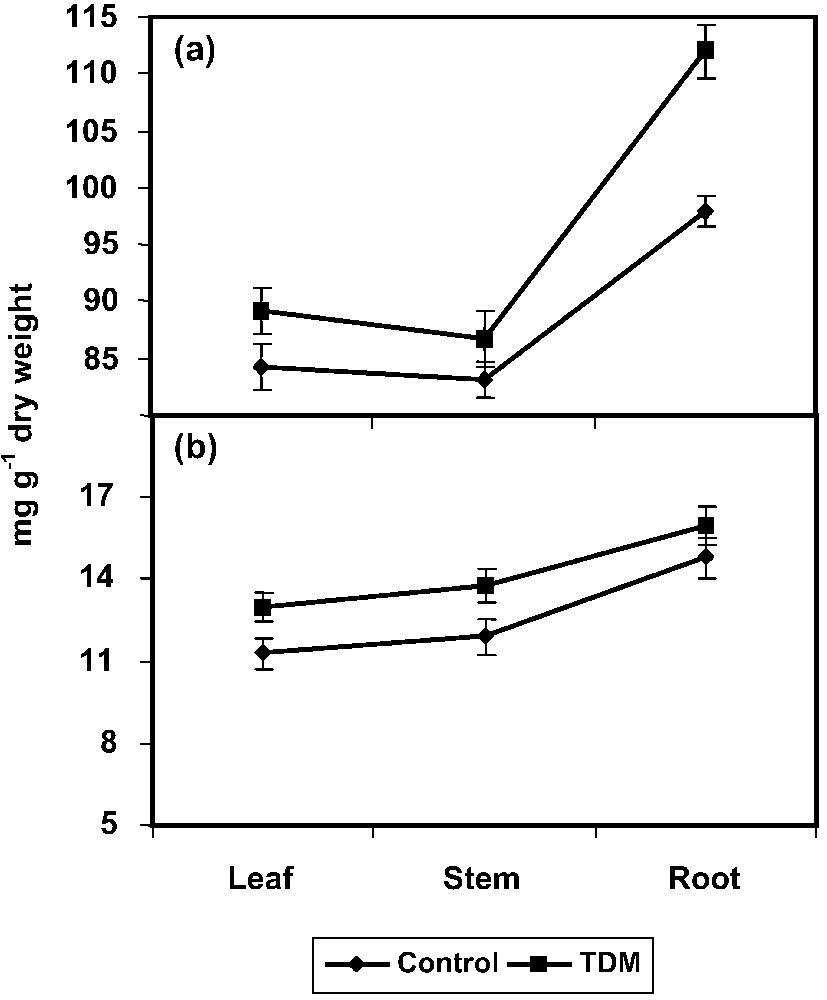
Effect of triadimefon on protein (a) and amino acid (b) contents of Catharanthus roseus. Values are given as means ± SD of six experiments in each group.
3.4 Effect of TDM on γ-glutamyl kinase and proline oxidase activities
The PRO metabolising enzyme, γ-GK increased under the TDM treatment in C. roseus seedlings (Fig. 4a). This enzyme plays an important role in the synthesis of PRO. The γ-GK activity can be inversely correlated with PROX activity and protein content in salt-treated plants [55]. PRO accumulation on TDM treatment seedlings can be attributed in part to the increased level of γ-GK activity [58].
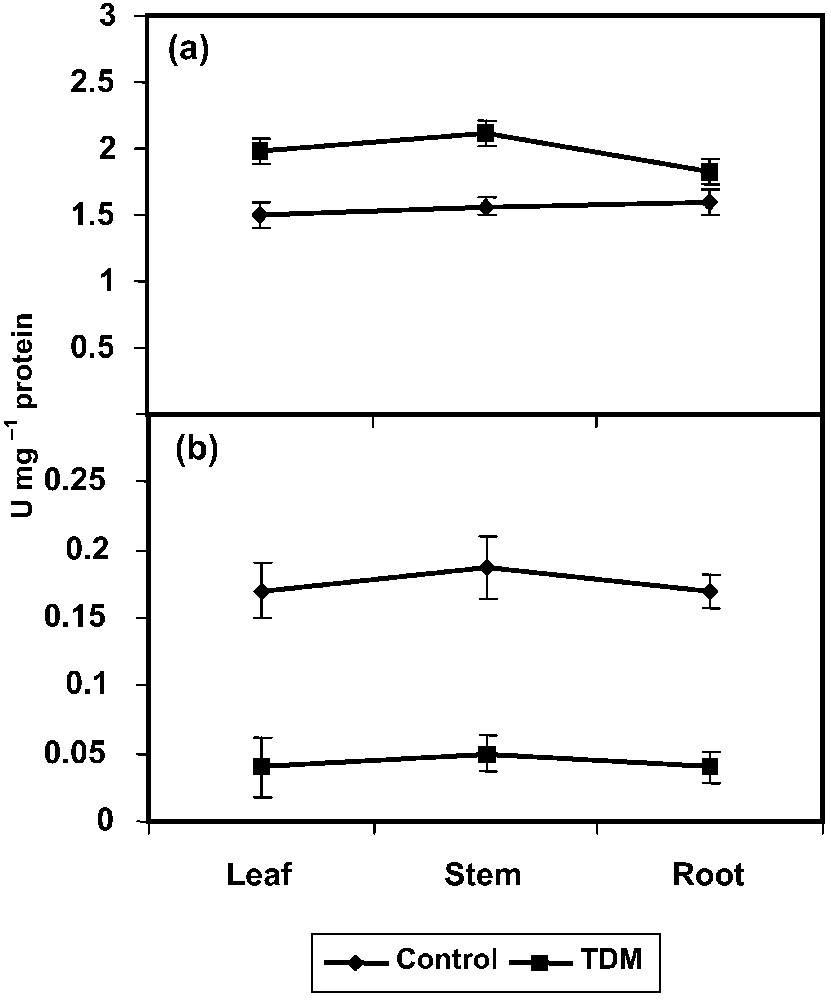
Effect of triadimefon on γ-GK (a) and PROX (b) activities of Catharanthus roseus seedlings. Values are given as means ± SD of six experiments in each group.
PROX activity decreased under TDM treatment in C. roseus seedlings when compared to control (Fig. 4b). This enzyme converts free PRO into glutamate. Reduction in PROX activity and simultaneous increase in PRO level were reported in low-temperature-stressed wheat [59]. PRO may act as a non-toxic osmotic solute preferentially located in the cytoplasm or as an enzyme protectant, stabilizing the structure of macromolecules and organelles. Accumulated proline may supply energy to increase tolerance to salinity [55]. PRO, as an osmoprotectant compound, plays a major role in osomoregulation and osmotolerance [17,54]. Protein hydrolysis under salt-stressed plants is associated with increased PRO content [55].
4 Conclusion
The activation of metabolic processes by fungicide TDM in medicinal plant C. roseus is evident from the above results. It caused inhibition of TBARS, H2O2 and EL in C. roseus plants when compared to control. Protein, amino acid, glycine betaine and proline contents significantly accumulated in C. roseus under TDM applications. Under TDM treatment, the activity of PROX decreased and the γ-GK activity increased. These findings are of great significance in the cultivation of this medicinal plant, as it is previously reported that TDM causes an enhancement of antioxidant metabolism and ajmalicine production in C. roseus.
Acknowledgement
The authors are very much thankful to Dr. M. Iqbal, Assistant Professor, Department of Botany, GC University Faisalabad, Pakistan, and to Prof. Dr. Shao Hong-Bo, Director, Institute of Life Sciences, Qingdao University of Science and Technology (QUST), Qingdao, China, for their critical readings and language corrections of this manuscript.