1 Introduction
The heterotrichous ciliate Fabrea salina Henneguy (1889) is the dominant protist in hypersaline environments, as it can withstand high salt environments through mechanisms of osmoadaptation and salt tolerance [1,2]. This protozoan has been shown to produce a mucilaginous substance that inhibits the growth of other halotolerant species such as the microalgae Dunaliella and different life-cycle stages of the Anostracan Artemia [3–5]. These competitive advantages have prompted researchers to investigate the ecophysiology of Fabrea, and experimental evidence is now accumulating on the influence of a variety of environmental factors such as nutrient availability, temperature and irradiance level on Fabrea dynamics [6–9]. Furthermore, because of the decline in the fish resource, a rapid increase in intensive aquaculture is taking place worldwide, requiring high-quality nutritious feeds. In this respect, pelagic Fabrea may be an appropriate candidate to be used by aquafarmers as a feed with minimal cost [10] due to its small length, short generation period, and ability to form resting-cysts. These ciliates have been shown to exhibit remarkable resistance to growth under adverse conditions [11–13] such as those found in multi-ponds salterns. The Sfax solar saltern (Tunisia) has been the focus of a series of hydrobiological studies [14] and we have acquired substantial information on the dynamics of F. salina along saline gradients of several ponds [14]. In the present study, the predation by F. salina of the Prymnesiophyceae, Isochrysis galbana, the Chlorophyceae Dunaliella salina, and the yeast Saccharomyces cerevisiae was estimated during in vitro incubation by monitoring prey abundances and length spectra. While the flagellate Isochrysis is commonly used in aquaculture to enrich zooplankton such as rotifers or Artemia [15], the literature on its use as food item for Fabrea salina is to our knowledge very scarce. The long-term objective of this study is to consider the large-scale culture of Fabrea salina as a food source for the growing aquaculture industry in Tunisia.
2 Materials and methods
F. salina and D. salina, were collected using a 5-l Van Dorn bottle below the water surface from multi-pond solar salterns located along the Sfax coast (Tunisia, 34°39′N and 10°42′E) (Fig. 1). F. salina was harvested using a plankton net with a 100-μm mesh size, from the first evaporation pond A16 (salinity close to that of seawater: ) (Table 1). D. salina was collected from the crystallizer pond TS (saturating salt concentrations: ), in which the phytoplankton community was entirely composed of this Chlorophyceae (Table 2). The second food item consisted of pure cultures of the Prymnesiophyceae I. galbana (Tinamenor S.A. Marisma de Pesués, Cantabria, Spain). Algae and the ciliate were acclimated over three months to continuous illumination (2000 lux) and to Walne's medium (modified from [16], Table 3), respectively.

General map of the geographical location of the multi-pond Sfax salterns along the coast to the south of Sfax (Tunisia) showing the ponds from which were harvested Fabrea salina (A16: evaporation pond) and Dunaliella salina (TS: crystallizer pond). Modified from [29].
Mean values and standard deviation (S.D.) of several physical, chemical, and biological parameters in the saltern
Ponds | A16 | TS |
Salinity (p.s.u.) | 78.7 ± 8.8 | 424.5 ± 35.6 |
Temperature (°C) | 23.7 ± 7.1 | 30.6 ± 8.6 |
pH | 7.6 ± 0.7 | 6.6 ± 0.9 |
Suspended matter (mg l−1) | 222.1 ± 148.6 | 3578 ± 2067 |
Water density | 1.08 ± 0.05 | 1.27 ± 0.06 |
Chemical parameters | ||
Total N (mg l−1) | 3.7 ± 2.2 | 10.1 ± 8.7 |
Total P (mg l−1) | 1.3 ± 2.4 | 5.2 ± 3.8 |
N/P ratio | 3.7 | 1.9 |
Chlorophyll-a (mg M−3) | 0.097 ± 0.079 | 0.036 ± 0.01 |
Biological parameters | ||
Bacterioplankton (× 106 cells ml−1) | 7.7 ± 5.8 | 25.3 ± 14.7 |
Phototrophic picoplankton (× 105 cells ml−1) | 4.1 ± 2.7 | 0.0 |
Nanoplankton (× 105 cells ml−1) | 18.6 ± 8.3 | 0.8 ± 0.4 |
Phytoplankton (× 106 cells ml−1) | 0.4 ± 0.3 | 1.2 ± 1.4 |
Ciliates (× 104 cells ml−1) | 4.7 ± 3.5 | 0.0 |
Zooplankton (× 104 ind m−3) | 0.2 ± 0.2 | 0.0 |
Specific composition of phytoplankton, ciliates and zooplankton sampled in ponds A16 and TS of the Sfax saltern
Ponds | A16 | TS |
Phytoplankton | ||
Diatoms | Navicula sp. | |
Pinnularia sp. | ||
Nitzschia sp. | ||
Surirella sp. | ||
Gyrosigma sp. | ||
Dinoflagellates | Oxyrrhis marina | |
Prorocentrum sp. | ||
Gymnodinum sp. | ||
Protoperidinum sp. | ||
Chlorophyceae | Dunaliella salina | |
Ciliates | Urotricha sp. | |
Fabrea salina | ||
Euplotes sp. | ||
Zooplankton | ||
Copepods | Acartia grani | |
Acartia clausi | ||
Harpacticus littoralis | ||
Bryocamptus sp. | ||
Tisbe longicornis | ||
Mesochra sp. | ||
Micosetella sp. | ||
Copepodits | ||
Nauplii | ||
Rotifers | Brachionus urceolaris | |
Brachionus calyciflorus | ||
Other zooplankton | ||
(Mainly larvae) |
Chemical composition of the medium used to culture Fabrea salina. MT: Metric tons
Elements | Concentrations (g MT−1) |
Chlorides | 16560 |
Sodium | 9210 |
Sulphates | 2324 |
Calcium | 350 |
Potassium | 343 |
Bicarbonates | 127 |
Brominates | 19 |
Strontium | 7 |
Boron | 5 |
Fluorine | 1.2 |
Manganese | 1.359 |
Molybdenum | 0.690 |
Lithium | 0.170 |
Rubidium | 0.110 |
Iodine | 0.070 |
Aluminium | 0.062 |
Zinc | 0.035 |
Copper | 0.0036 |
The commercially available dry yeast, Saccharomyces cerevisiae, was also used as a third food item for Fabrea. Cultures for experimental purposes were maintained in exponential-growth phase by regular transplants to a fresh medium. The cultures were synchronised, 24 h prior to the beginning of the grazing experiments. This was carried out for 6 days in 200-ml pre-sterilised flasks. Two sets of control (algae without Fabrea) and experimental flasks (two replicates each) were used during the experiment in a batch system with a salinity of 50 p.s.u. and maintained at 24 °C in temperature-controlled chambers, and under an illumination of 2000 lux. Ciliates were counted three times in each replicate flask.
The initial concentration of Fabrea was 4 cells ml−1 and the three food items were inoculated separately from each other, in the morning, during the six days of incubation at a density of , estimated using a Burcker haemocytometer. The food density was maintained during the experiments by a daily cell enumeration. To stop the experiment, a glutaraldehyde solution (Sigma grade I, final concentration 1%) was added to inhibit protozoan motion [17]. A Sedgwick-Rafter counting cell (Graticules LTD, Tonbridge, Kent, UK), mounted on a Type Leica DM LS2 microscope (20X magnification), was used to estimate the average daily changes in Fabrea numbers; Fabrea length and width were estimated using a micrometer and the biovolume of Fabrea was measured according to [18].
The specific growth rate was calculated using the following formula:
2.1 Statistics
Mean and standard deviation (SD), as well as boxplots are reported when appropriate. Simple linear regression was used when analyzing how each food item could explain the relation between the length and biovolume of Fabrea. One-way ANOVA followed by a post-hoc comparison using Tukey's test [19] was applied to identify significant differences between food treatments for (i) density, (ii) length, and (iii) biovolume of Fabrea.
3 Results
3.1 Fabrea density and growth rate
The density of Fabrea fed with D. salina did not exhibit significant changes from the start until the third day of the experiment. The growth rate was low () and cell numbers did not exceed 2.5 (±1.41) cells ml−1 (Fig. 2a). However, from day 4 until the end of incubation, Fabrea cell numbers increased rapidly (), reaching 17 ± 2.12 cells ml−1 on the 6th day (Fig. 2a). When cultured with I. galbana, the density of Fabrea increased until day 3 (, ) and then collapsed (). From the 4th day until the end of the experiment, Fabrea grew strongly () up to (Fig. 2b). A similar pattern in the distribution of Fabrea was recorded when the protozoan was fed with the yeast S. cerevisia. However, no latency time on the fourth day was observed (Fig. 2c). Indeed, Fabrea grew () until the second day (), then its density decreased until the fourth day (). From day 4 onwards, the ciliate growth rate increased again (), yielding a cell density of . From the first day until the end of each grazing experiment, the specific growth rates (μ) of Fabrea fed with Isochrysis, Dunaliella and Saccharomyces were 0.3, 0.24 and 0.02, respectively (Fig. 3).
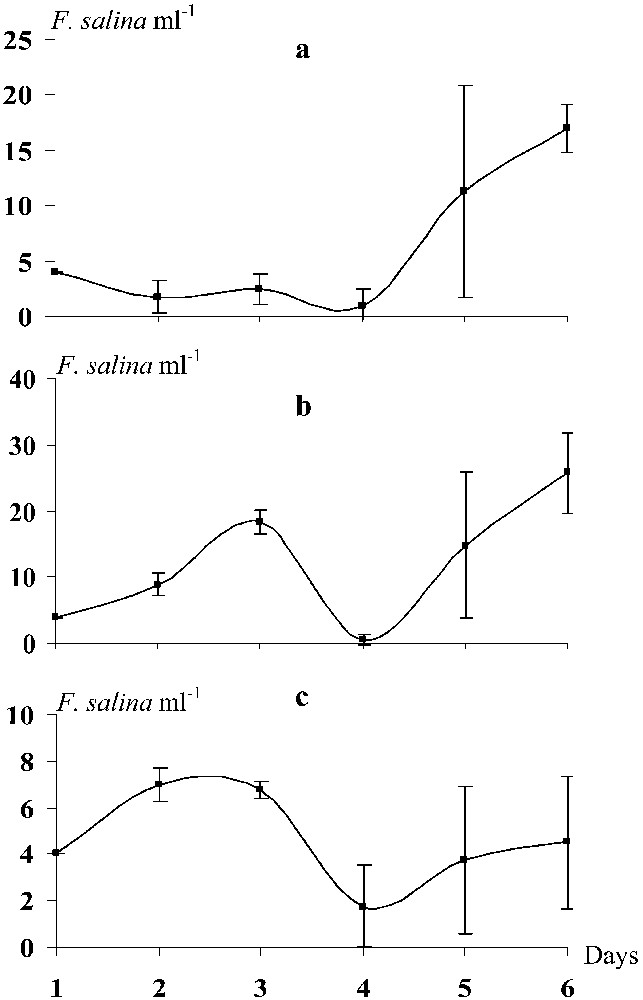
Daily evolution of the density of Fabrea salina reared in the presence of Dunaliella salina (a), Isochrysis galbana (b) and Saccharomyces cerevisiae (c). The vertical bars represent the standard deviation.
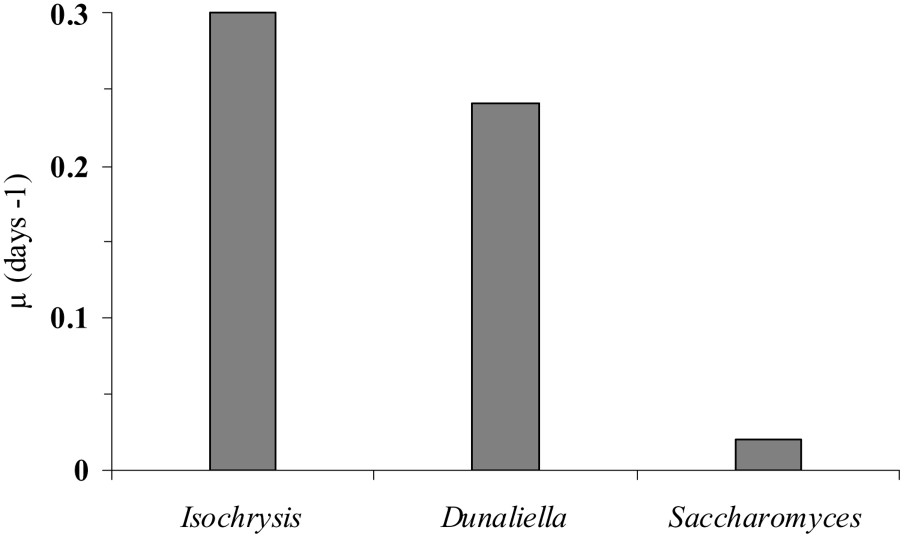
Specific growth rates (μ) of Fabrea fed with different preys from the start to the end of grazing experiments.
3.2 Length and biovolume of Fabrea
When fed with D. salina, the length of Fabrea varied from , recorded in the latency phase to , recorded on the second day, corresponding to a biovolume of (Table 4). The average length of Fabrea was , corresponding to a biovolume of . During the exponential growth phase, the length of Fabrea was high (), with a biovolume of (Table 4).
Mean and standard deviation (SD) of length (μm) and biovolume (× 105 μm3) of Fabrea salina reared separately from each other with different preys
Feeds | Dunaliella salina | Isochrysis galbana | Saccharomyces cerevisiae | |||||||||||||||
Parameters | Length | Biovolume | Length | Biovolume | Length | Biovolume | ||||||||||||
Days | Media | Min | Max | Media | Min | Max | Media | Min | Max | Media | Min | Max | Media | Min | Max | Media | Min | Max |
2 | 281.5 | 140.5 | 290.2 | 15.8 | 7.9 | 16.3 | 165.0 | 148.1 | 207.4 | 4.2 | 2.7 | 6.0 | 140.0 | 110.6 | 160.6 | 4.5 | 2.9 | 7.2 |
3 | 251.9 | 180.0 | 270.1 | 14.2 | 6.4 | 15.2 | 191.0 | 163.0 | 222.2 | 7.5 | 5.1 | 11.7 | 152.0 | 118.5 | 177.8 | 4.7 | 2.8 | 7.4 |
4 | 200.0 | 163.0 | 266.7 | 19.2 | 6.7 | 30.0 | 251.9 | 180.1 | 265.0 | 2.6 | 6.4 | 14.6 | 148.1 | 100.5 | 180.3 | 4.3 | 3.1 | 7.9 |
5 | 260.7 | 190.0 | 278.3 | 14.7 | 8.7 | 15.7 | 219.0 | 89.0 | 104.0 | 10.7 | 7.4 | 13.3 | 177.8 | 120.4 | 210.0 | 7.4 | 4.7 | 8.9 |
6 | 234.6 | 192.6 | 266.0 | 20.0 | 7.9 | 39.0 | 180.0 | 133.0 | 222.0 | 11.8 | 5.5 | 28.8 | 165.0 | 148.0 | 207.0 | 5.6 | 4.3 | 8.6 |
When I. galbana was used as diet, the length of Fabrea varied from on the second day () to recorded on the fourth day (). Mean Fabrea biovolume recorded over the 6 days of experiments (), corresponded to a mean length of . However, at the end of the experiment, the length of Fabrea decreased, to reach .
When fed with dry yeast, the length of Fabrea ranged between and (), corresponding to a mean biovolume of . Conversely to the temporal distribution of the prey-predator couples Fabrea–Isochrysis and Fabrea–Dunaliella, the average length of Fabrea cultured with yeast increased throughout the experiment (Figs. 4 and 5).

Daily evolution of the length of Fabrea salina in the presence of Dunaliella salina, Isochrysis galbana and Saccharomyces cerevisiae. Vertical bars represent the standard deviation.

Boxplot showing the distributions of the variables: density, length, and biovolume of Fabrea for the three treatments with Dunaliella, Isochrysis and Saccharomyces. (The thick line in the middle of the box indicates the median value.)
4 Discussion
The results indicate that under controlled experimental conditions, F. salina was able to grow when its diet consisted of D. salina, I. galbana and S. cerevisiae. Indeed, continuous light, a temperature of 24 °C, a salinity of 50 p.s.u. and a small water volume (200 ml) were sufficient to yield optimal growth of Fabrea. This is consistent with the findings of several authors, who showed that Fabrea can develop with various nutritional items [13]. Moreover, Rattan et al. [20] indicated that Fabrea can even grow with fermented wheat and rice grains. However, Repak [6] indicated that this ciliate does not grow in the presence of the Cyanobacteria Synechococcus spp. Many other protists have been successfully cultured, such as Favella sp., Uronema sp., Gymnodinium sp. [21,22]. A high growth rate () was also recorded when the Tintinnid Favella sp. was fed with the Prymnesiophyceae Prymnesium parvum [22]. One should also bear in mind that it is of fundamental importance to keep growth and ingestion constant to evaluate growth yield in ciliates feeding different preys [23–25]. Although yield is constant, absolute biomass production may be higher in one prey than in another. Both high density and growth rates of F. salina were recorded when the diet consisted of I. galbana (26 cells ml−1, ). Indeed, the peak density recorded on the third day indicated a short-generation period of Fabrea, which may suggest that I. galbana had a high nutritional value, as already reported by Brown [26]. To our knowledge, the culture of Fabrea with Isochrysis has never been reported, so we were unable to compare our findings with those of others. The only available data concerned the genera Strobilidium and Strombidium, which exhibit a maximum growth rate of 2.2 day−1 when fed with Isochrysis [27]. Although the effects of this flagellate on Fabrea development were remarkable, both the length and biovolume of the ciliate were smaller than those recorded when the Fabrea diet consisted of D. salina. This discrepancy may suggest that Fabrea underwent reproduction, as evidenced by the existence of two peaks of Fabrea density recorded on the third and sixth days (Fig. 2b), coinciding with a decrease in cell dimensions on the sixth day (Fig. 4). In support of this, we also found the weakest correlations between the length and biovolume of Fabrea fed with I. galbana (, , ), versus D. salina (, , ) and the yeast (, , ). The highest densities of Fabrea were found when the latter was fed with D. salina and I. galbana. Pandey and Yeragi [13], who worked under similar experimental conditions, reported similar results with D. salina, but they did not consider I. galbana as a food item. They recorded cell densities of 44 and 64 Fabrea ml−1 when grown in 1 and 5-l containers, respectively. Concerning the yeast S. cerevisiae, our results indicate that it was not a good food item for Fabrea, because the ciliate induced the lowest growth rate, cell length, and biovolume. The ANOVA analysis shows that both the density (, ), length (, ) and biovolume (, ) of Fabrea fed yeast differed significantly () from those of Fabrea fed both with D. salina and I. galbana (Tables 5 and 6). This difference may be explained by the deterioration of the medium throughout the experiment induced by yeast, as already suggested by [20]. Indeed, the high Fabrea density recorded on the second day implies a very rapid growth generation period which was strongly stimulated by S. cerevisiae at the beginning of the experiments. Yeast is widely used as a dietary supplement to support the growth of several species such as rotiferans and the anostracan Artemia. Pandey and Yeragi [13] found a density of 20 Fabrea ml−1 when fed with yeast at concentrations of 5 mg l−1. Overall, our results are slightly lower than those reported by Repak [6,7] and Pandey and Yeragi [13]. The maximal length of Fabrea recorded in this study (207 μm) is similar to that recorded by Dolapsakis et al. [28], who cultured F. salina obtained from a Greek saltern in which the salinity varied from 60 to 144 p.s.u. Furthermore, both the length and biovolume of Fabrea recorded in this study were higher than those found in the Sfax saltern. The values reported by [14] from this ecosystem did not exceed 111 μm and , for salinities ranging between 70 and 170 p.s.u., respectively.
Results of ANOVA analysis to assess the effect of different food combinations on the density, length and biovolume of Fabrea
Parameters | F value | d.f. | P value |
Density | 18 | 57 | 6.38×10−7*** |
Length | 33 | 57 | 3.48×10−10*** |
Biovolume | 19 | 57 | 5.61×10−7*** |
Post-hoc comparison using Turkey's test to identify significant differences among treatments
Feeds | Density | Length | Biovolume |
Isochrysis–Dunaliella | 0.12 | 0.00⁎ | 0.00⁎ |
Saccharomyces–Dunaliella | 0.00⁎ | 0.00⁎ | 0.00⁎ |
Saccharomyces–Isochrysis | 0.00⁎ | 0.02⁎ | 0.45 |
⁎ p values below 0.05 indicate significant differences between the two treatments.
In conclusion, our study indicates that the ciliate Fabrea salina was able to grow with different feeds, and may be a good candidate species as food source for the Tunisian aquaculture industry. To improve our overall understanding of the various preys–Fabrea relationships, we are currently investigating the ecophysiological responses of Fabrea to changes in light, temperature, salinity, together with its fatty acid composition.
Acknowledgements
We gratefully acknowledge support from the staff of the Sfax Saltern Campany. We would like to thank especially Dr. Roberto Marangoni for helpful advices and comments on the manuscript. The pure culture of Isochrysis galbana was kindly provided by Dr H. Chavanne (Lazzaro Spallanzani Institute, Milan, Italy). This work was conducted as part of a collaborative project between the University of Sfax (Tunisia) and the University of Franche-Comté (Besançon, France). We thank the Tunisian Ministry of Research and Technology for financial support.