1 Introduction
All milk variety contains a certain level of somatic cells. These are neutrophils (PMN cells), lymphocytes, and macrophages. Macrophages comprise the major cell type in milk from uninfected cow [1,2]. When there is bacterial infection, tissue damage, or other inflammation processes affecting the mammary tissue, the number of SCC in milk increases dramatically [2,3]. This increase in SCC results from a transfer of white blood cells (immune cells) from the blood to the mammary gland [4–6]. As a result, the relative proportions of cell types present in milk change significantly, with an increase in the PMN level (up to 90%). Their primary function is the protection of the udder from bacterial challenge [7,8]. During mastitis, PMNs emigrate from the peripheral blood into milk through the mammary epithelium in response to chemotactic stimuli produced locally as a reaction against invading microorganisms or their components [9–11]. PMNs then phagocyte foreign particles or bacteria, discharge their granules' content, and generate reactive oxygen metabolites [12].
These active species that result from the inflammatory response may include superoxide anion (O2−), hydroxyl radical (OH•), hydrogen peroxide (H2O2) and fatty acid radicals. Although these reactive oxygen metabolites are harmful to bacteria, they also have the potential to destroy host cells. Several studies on the inflammation process have shown that oxidants released by PMN induce tissue damage [13,14]. Oxidants are known to be cytotoxic through many different mechanisms, such as protein and amino acid oxidation, lipid peroxidation, and DNA damage.
Beyond the presence of valuable macro- and micronutrients, milk also contains antioxidant factors. These are represented by naturally vitamins (i.e. E and C), beta-carotene, and enzymatic systems, mainly represented by superoxide dismutase, catalase, and glutathione peroxidase [15–17]. These antioxidant factors are important for the control of the reactive oxygen metabolites concentrations produced by somatic cells [18,19]. To our knowledge, the interaction between milk oxidative state and cellular counting has not yet been fully considered in bovine species. Therefore, the purpose of the present study was to examine possible relationships between the total and differential bulk milk somatic cell counts, a marker of degradation of the hygienic conditions, and the activities of various antioxidative enzymes.
2 Materials and methods
2.1 Sample collection
Forty-three samples of bulk tank milks were randomly selected from eight dairy farmers in the region of Sfax (Tunisia) in winter between November 2005 and February 2006. Milk samples were collected aseptically during the routine evening milking into sterile bottles and were immediately carried to the laboratory. During milk collection and preparation, efforts were made to avoid microbial contamination. The raw unpreserved samples were stored overnight at +4 °C and analyzed for absolute and differential somatic cell counts on the following day. An aliquot of 15 ml was taken from each collection and stored at −20 °C for enzymatic assays.
2.2 Absolute and differential somatic cell counts
Total and differential BMSCC were determined microscopically in smears stained with May–Grünwald and Giemsa reagents. For each milk sample, two preparations were done. The differential cell staining allowed the accurate determination of the cell type, according to the methodology of Gargouri et al. [20] adapted to cow milk. For each slide, the enumeration concerned 16 areas. The working factor was 393,174 in all cases, and the average values were used for statistical analyses. The working factor was obtained taking into account the field diameter (0.18 mm), the observed total area (100 mm2), and the milk volume (0.01 ml).
2.3 Enzymatic assays
Catalase (EC 1.11.1.6) activity was measured according to [21]. Briefly, the assay mixture consisted of 100 mM of phosphate buffer (pH = 7.0), 500 mM of H2O2 and 10 μl of milk, in a final volume of 1.0 ml. The reaction started by adding H2O2, and its decomposition was monitored by following the decrease in absorbance at 240 nm for 1 min. The enzyme activity was calculated by using an extinction coefficient of 0.043 mM−1 cm−1. Catalase activity was calculated in terms of micromoles of H2O2 consumed per milligram of protein and per minute of incubation [μmol H2O2 (mg min)−1].
SOD (EC 1.15.1.1) activity was assayed by measuring its ability to inhibit the photoreduction of nitroblue tetrazolium ‘NBT’ [22], 10 μl of milk combined with 50 mM of phosphate buffer (pH 7.8), 39 mM of methionine, 2.6 mM of NBT and 2.7 mM of EDTA. Riboflavin, was added last to obtain a concentration of 0.26 mM, and switching on the light started the reaction; changes in absorbance at 560 nm were recorded after 20 min. In this assay, the activity was expressed in relative units per milligram of protein [U mg−1]. One unit of SOD activity is defined as the amount of protein that inhibits the rate of NBT reduction by 50%.
Glutathione peroxidase (EC 1.11.1.9) activity in milk was performed by estimating the content of oxidized glutathione formed by the action of glutathione peroxidase as described by [23], but modified using the H2O2 as substrate with the presence of DTNB. One unit of activity catalyzes the oxidation by H2O2 of 1.0 μmol of reduced glutathione to oxidized glutathione per minute at pH 7.0 at 25 °C. The specific enzyme activity was expressed in units per gram of protein [U g−1].
The protein concentration (mg ml−1) in all samples was evaluated according to [24] with bovine serum albumin as standard.
2.4 Statistics
Statistical evaluations were performed using SPSS software (Version 13). Two separate types of statistical analyses were done. Firstly, to allow presentation of absolute values of measured parameters, milk samples were divided into three groups, according to their absolute BMSCC. Group 1: ; group 2: to ; group 3: . The frequency distributions for BMSCC and different cells were skewed, and thus transformation was applied prior to the analyses. Secondly, results for milk enzymes were used to determine correlation coefficients (r) between absolute log10 BMSCC, differential BMSCC and catalase, GSHPx and SOD activities. A level of was accepted as statistically significant.
3 Results and discussion
3.1 Absolute and differential somatic cell counts
SCC ranged from to in bulk milk (Table 1). A high variability in the cell count was observed. These SCC represent a wide range of raw milk quality that can be encountered in the Tunisian dairy industry. The health status of the animals' udders (mastitis or inflammation) is the most important cause of elevated milk SCC, and age of animal, stage of lactation, season, milk yield, husbandry practices used and climate are also known to influence milk SCC [25,26]. Therefore, 100% of the total samples of this study had more than or herds with probable subclinical mastitis and 70% more than or herds, with probable clinical mastitis [27]. This result, which does not comply with most SCC limits legally determined in several countries, indicates the high incidence of infected cows in Tunisian dairy herds. Thus, careful attention to proper milking practices could help to minimize this problem. It is also recommended that the fluid milk industry consider implementation of premium-quality payment programs to motivate dairy producers for low SCC milks.
Absolute and differential somatic cell count and compositional parameters according to bulk milk somatic cell counts (BMSCC)
Milk according to BMSCC () | ||||
Mean ± SEM | Group 1 <1000 | Group 2 1000–1500 | Group 3 >1500 | |
N | 43 | 20 | 12 | 11 |
BMSCC | ||||
BMSCC mean (×103/ml) | 1326 ± 134 | 726 | 1314 | 2430 |
Log10 BMSCC | 6.05 ± 0.03 | 5.85 | 6.11 | 6.35 |
Macrophage (%) | 7.61 ± 0.64 | 7.29 | 8.80 | 6.91 |
Lymphocyte (%) | 64.39 ± 2.24 | 74.49 | 63.40 | 47.11 |
Polymorphonuclear (%) | 28.00 ± 2.24 | 18.22 | 27.80 | 45.98 |
A significant () difference between the three categories of milk was found for their individual cell types (Table 1). The relative proportion of lymphocyte and PMN percentages changed significantly, but macrophages did not. The dominant cell population in all groups was the lymphocyte, but its percentage declines with total number of cells (74 to 47%). The inverse trend was observed for the PMN proportion (18 to 46%). The massive recruitment of these two cell types, in response to intramammary infection, confirms the poorer udder health status of dairy cows [7,28,29]. The results from this experiment differ from others. Dosogne et al. [1], Pyörälä [30], and Lindmark-Månsson et al. [2] found that macrophages were the most predominant cell population in normal dairy milk. A possible explanation of these different results could be the higher level of BMSCC found in this study.
The BMSCC was significantly () correlated with LYM and PMN. Correlation coefficient between PMN and BMSCC () was slightly smaller than that between LYM and BMSCC (). This showed, as suggested by [5], the great variability in PMN in milk samples of similar total SCC. The correlation between bulk tank MAC levels and total BMSCC (), although highly significant, was lower than the corresponding correlations with LYM and PMN. Moreover, PMN comprise the major cell type in milk.
3.2 Relationships between milk antioxidant enzymes and absolute or differential BMSCC
3.2.1 SOD activity
The mean value of SOD activity in all milks was , a value not significantly different from that reported by [17] in bovine milk or [31] in human milk. The low SOD activity in cow milk was also mentioned by Filipović et al. [32] and Kasapovic et al. [33]. This activity ranged from 2 to 3 U mg−1 of protein. Other studies showed the nearly absence of SOD activity in bovine milk [34]. These observations confirmed those reporting that SOD in bovine milk is approximately 100 times less than that in bovine erythrocytes.
In this study, the SOD was not affected by BMSCC and differential BMSCC (Fig. 1). Hicks [35] found that the SOD activity is not correlated with the somatic cells of milk and not influenced by an elevated cellular numbering. Koryka-Dahl et al. [36] and Holbrook and Hicks [37] have examined the SOD in bovine milk and reported that the enzyme was identical, from the point of view of its electrophoretic properties, to those of bovine erythrocyte SOD, with no evidence that SOD was derived from bacterial or somatic cells present in milk. They also reported that all the SOD activity was located in the milk's serum phase, because no activity was associated with the fat fraction. It seems that reactions of dismutations achieved by the SOD are weakly dependent on BMSCC, suggesting that PMNs have minor, if any release of the superoxide anion (O2−). Therefore, oxidative stress generated with elevated somatic cell number seems to touch weakly the first antioxidant barrier, and the formed free radicals do not require SOD intervention. This pleads in favour of the formation of the hydrogen peroxide (H2O2) by immunity cells, free radicals not requiring a dismutation and playing an important role in intercellular immunities signals that come with the elevated SCC. According to a study conducted in vitro by [38], the PMNs activated by Escherichia coli can damage mammary epithelial cells by the oxygenated radicals. SOD incorporation did not prevent the cytotoxic effect of these radicals. This points out the importance of the other antioxidants, as the catalase and the GSHPx.
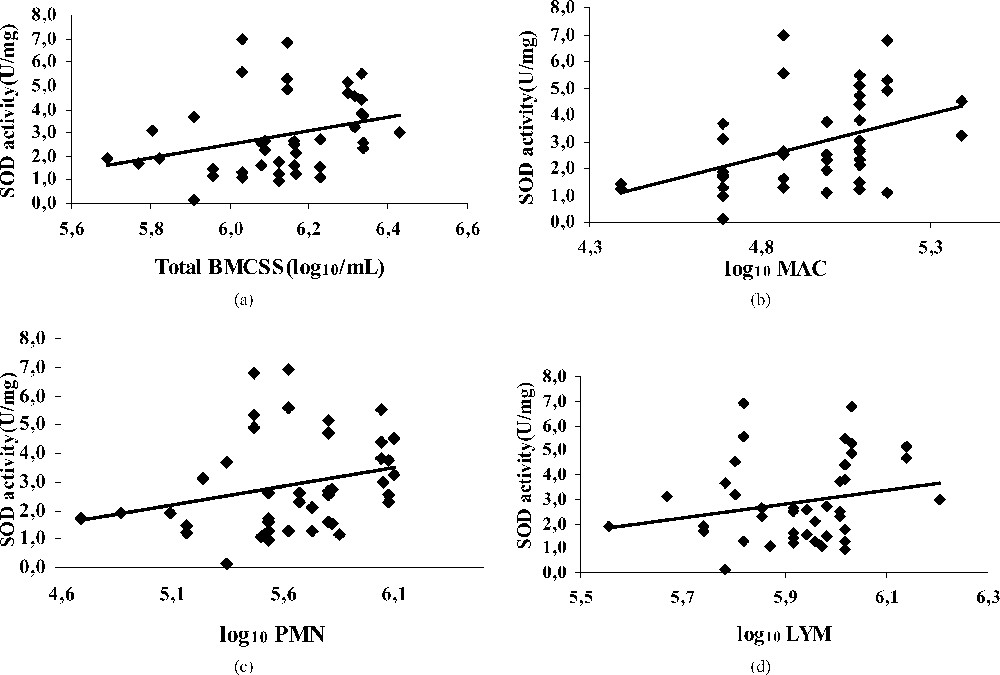
Regression plot showing the relationship between SOD activity [U/(mg)], and BMSCC (a), polymorphonuclear (b), macrophage (c) and lymphocyte (d) in bulk tank milks.
3.2.2 Catalase activity
The mean value of catalase activity for all milks was H2O2 (mg min)−1. The catalase activity increased more with neutrophils than with macrophages and lymphocytes (Fig. 2), indicating its role in free-radical decomposition in the case of bacterial infection resulting in an important increase of milk somatic cells. Our results confirm those of Kitchen et al. [39] and Read et al. [40], who found a positive correlation between the catalase activity and the milk somatic cell counts. On the contrary, Phillips and Griffiths [41] signalled no relationship between those two parameters.
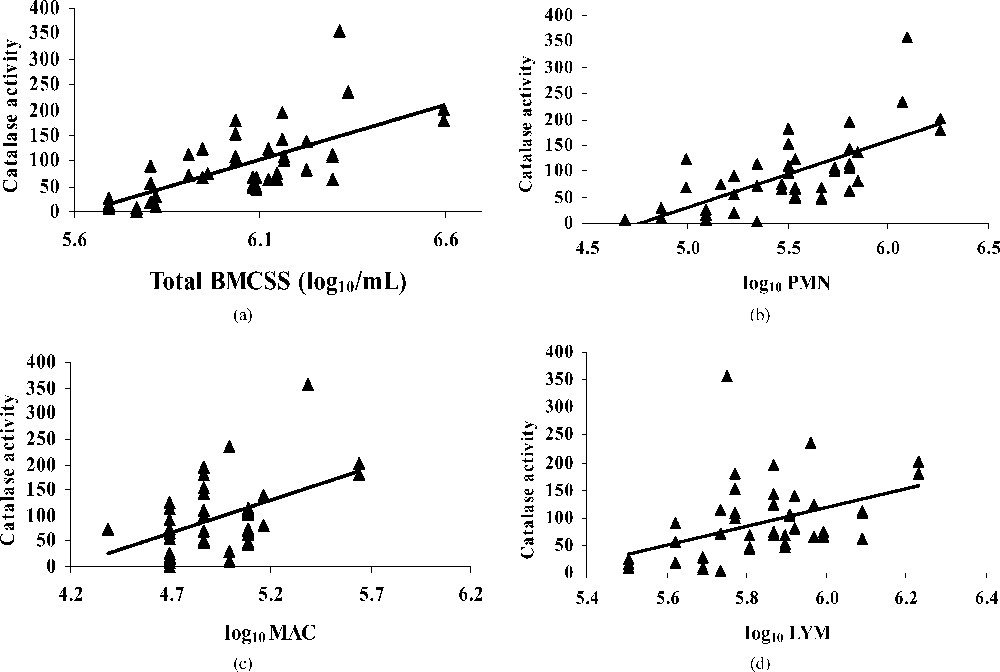
Regression plot showing the relationship between catalase activity [μmol H2O2/(mg min)] and BMSCC (a), polymorphonuclear (b), macrophage (c), lymphocyte (d) in bulk tank milks.
Silanikove [42] signalled that catalase plays a central role in milk redox control. Especially during mastitis, catalase activity increase markedly [39–43], making it a useful indicator of mastitis.
Catalase has been reported to scavenge hydrogen peroxide and is a part of intracellular defence systems against oxidation. The high catalase activity in relation with BMSCC might be an adaptive mechanism to the superfluous hydrogen peroxide released by PMNs in high milk SCC. The intervention of this antioxidant is crucial to reduce mammary cell damage [38]. However, some concern may be raised, because many of the common bacterial contaminants produce higher concentrations of catalase [44] and could interfere with the analysis of the native enzyme in milk.
3.2.3 GSHPx activity
GSHPx is one of the key antioxidative enzymes in milk. It catalyses the reduction of different peroxides, providing protection against oxidative damage [45,46]. However, knowledge of the role of the GSHPx in bovine milk is limited, and only assayed by some authors [47]. In our study, the mean GSHPx activity of all groups of milk was 33.02 U g−1. This value is comparable to those signalled by Hojo [48], Debski et al. [49], and Bhattacharya et al. [50] in human milk (31–39 U ml−1).
Our results show a positive correlation between the activity of this enzyme and the BMSCC (Fig. 3a), especially with neutrophils (). The increase of this activity is synonymous of the presence of free radicals (H2O2) in the loaded milk. In the same way, some authors related this activity to the concentration of polyunsaturated fatty acids [31]. In our study, the fat content in high somatic cells milk is raised (data not shown), which can explain this result.
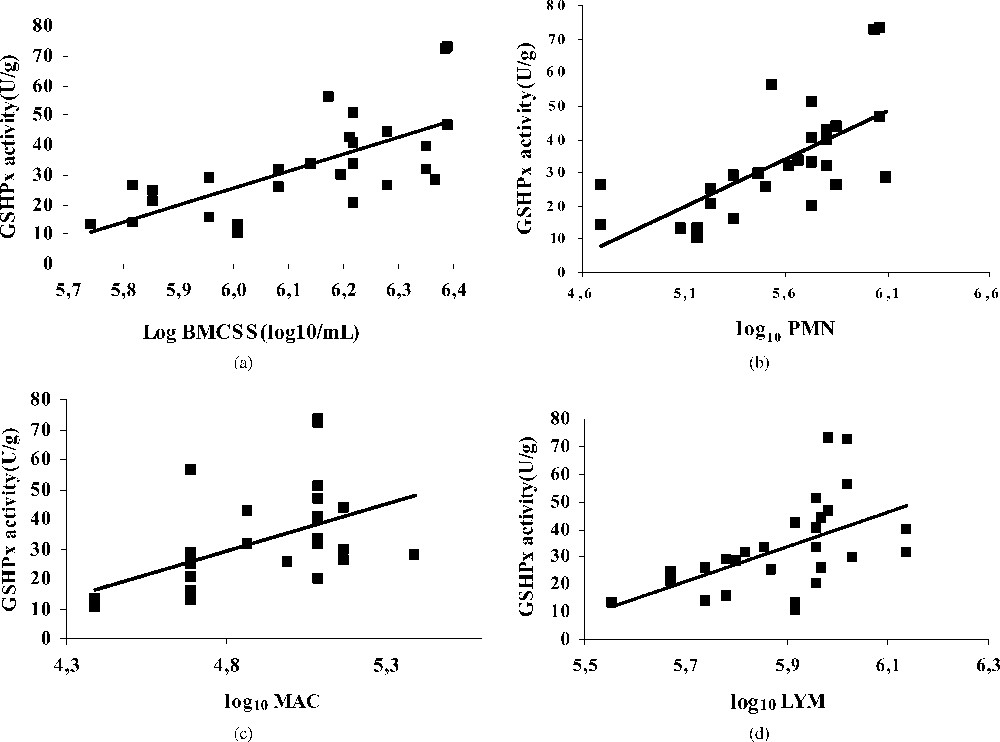
Regression plot showing the relationship between GSHPx activity [U/g] and BMSCC (a), polymorphonuclear (b), macrophage (c), lymphocyte (d) in bulk tank milks.
Neutrophils are the principal cell type found in mammary tissues and milk during the early stage of the inflammatory process associated with bacterial infection [51]. These cells migrate from the blood to the mammary gland in response to a variety of inflammatory mediatory pathogens in order to phagocyte and kill bacterial pathogens [52]. Neutrophils are essential for effective host defence, but they are also known to be involved in various inflammatory diseases [13,53]. Activated PMN reach the infected site and release toxic reactive oxygen species via an oxygen-dependent pathway.
In the case of acute mastitis such as those caused by Escherichia coli, the amount of reactive oxygen metabolites released by PMN may overwhelm the cow's endogenous antioxidant protection mechanisms. Therefore, it is plausible that supplementation with exogenous antioxidants may facilitate the cow's recovery from mastitis and protect the secretory epithelial cells [38].
We show therefore that the free radicals formed in milks with high SCC are essentially H2O2, which intervenes in immunity reactions and requires the catalase and the GSHPx to be neutralized. Thus, concentrations and activity of antioxidants in milk determine the degree of protection of the mammary gland against oxidative stress.
3.3 Interrelationships between milk enzyme activities
The coefficients of linear pairwise regression for the enzyme activities in bulk milk are shown in Fig. 4. There is a statistically significant and positive correlation between SOD and catalase (, ) and between catalase and GSHPx (, ). However, the correlation between SOD and GSHPx is almost equal to zero ().

Pair correlation between SOD, catalase and GSHPx activities in bulk tank milks. NS, non-significant; .
These results suggest that the SOD-catalase and catalase-GSHPx tandems are the main coherently pathways for reactive oxygen metabolites neutralization in the mammary gland. Indeed, it is well known that catalase and GSHPx enzymes have been considered the primary scavengers of hydrogen peroxide [54,55]. In contrast, the parallel SOD-GSHPx pathway could act in the regime of free fluctuating or ‘sleeping’ mode and may become fully involved only with an acute oxidative stress.
4 Conclusions
The present study deals with the relation between BMSCC and antioxidant enzymes in the mammary gland. The preliminary result confirms that there is a remarkably high level of BMSCC on Tunisian dairy farms. The BMSCC is higher than the recommended threshold of SCC for healthy cows. The relative proportion of lymphocyte and PMN changed with the total BMSCC. The preponderant cell population was the lymphocyte one, but PMN became dominant for milk with more than . The higher activities of antioxidant enzymes were observed in milks with a high proportion of PMN. This may indicate that PMN caused changes in antioxidative status in mammary glands, followed by oxidative damage. During the inflammation of the mammary gland, these cells are able to produce reactive oxygen metabolites. In this case, it seems that catalase and GSHPx are the principal enzymatic defence coping with free radicals induced by neutrophils. The result that SCC/PMN, at high level, caused increases of catalase and GSHPx activities in mammary glands indicates that biological membranes were attacked by free radicals.
Lastly, it is important to emphasize that this SCC/PMN-related increase in the oxidative-stress behaviour is representative of bulk milks that correspond to a commingling of milks with very different SCCs. It will be very interesting to confirm these results with individual cow milk or milk from groups of cows with similar milk SCCs. Nevertheless, further works are required to clarify the mechanism by which increasing BMSCC/PMN generates reactive oxygen metabolites and affects oxidative stress in mammary glands.