Foreword
France, by the diversity of its soils and climates, is a major country of seed production because any kind of culture will find suitable conditions. In 2007, 310,000 hectares have been devoted to seed production. With a turnover of nearly 2 billion €, of which 650 million € for export, France is the leading European seed producer and the third largest seed exporter (www.gnis.fr/). Moreover, France is the largest exporter of agricultural products and food (which directly or indirectly derive from seeds) of the European Union, far above the car industry. In that sector France also occupies the second place globally. Indeed, 20% of agricultural production in Europe is French. From these results, the food sector occupies a pivotal position in the foreign trade of France, accounting for nearly 74% of total French exports. Not surprisingly, then, the seed is the object of intense genetic and genomic studies because it is a key factor of crop yield and for providing food and feed for human and animal nutrition.
The seed is the major form of dispersal of plants in the environment. It results from a complex process, the double fertilization of ovule by a pollen grain. A seed contains an embryo (the future plant), storage compounds necessary for the nutrition and growth of the embryo during germination (endosperm, cotyledons, perisperm), and teguments (a shield to protect the embryo against biotic and abiotic stresses) (Fig. 1). Seed development terminates, for most species growing in temperate climates, with a desiccation phase, after which the embryo enters in a quiescent state permitting its storage and survival for many years. The seed, therefore, occupies a central position in the plant life cycle. Mature seeds are resting organs, having low moisture content (5–15%) with metabolic activity almost at a standstill. For most species, this seed maturity is coupled with the set up of dormancy, a process preventing early germination [1]. For germination to occur, seeds need to be hydrated under conditions that encourage metabolism – for example, a suitable temperature and the presence of oxygen [2,3].
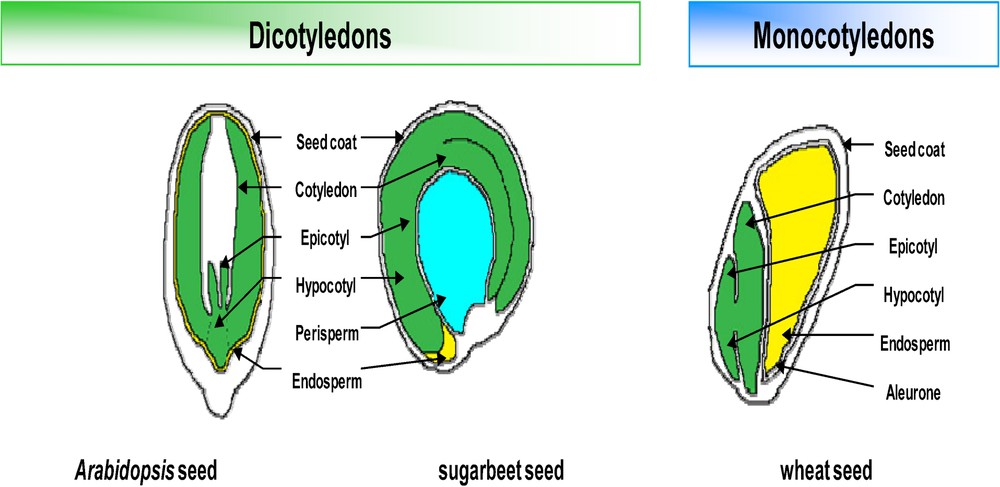
Schematic structure of dicot and monocot seeds, from [28].
Seeds of many plant species are extremely tolerant to harsh environmental conditions provided they are in a state of desiccation. Spectacular cases of seed longevity have been reported. Thus, radiocarbon dating allowed the determination of the age of viable date (Phoenix dactylifera L.) seeds to about 2000-year old [4].
In angiosperms (flowering plants [5]), seed formation results from a double fertilization process, where one of the sperm nuclei fuses with the egg nucleus to form the diploid zygote, while a second sperm nucleus fuses with two polar nuclei giving rise to the triploid tissue endosperm [6]. The embryo is often a plant in miniature possessing a root (radicle), a starting stem (hypocotyl), and leaves (cotyledons). Flowering plants have been classified in two groups. By definition, the embryos of seeds of the dicots possess two cotyledons (e.g., Arabidopsis thaliana (the model plant for genomic and post-genomic studies [7]), Medicago truncatula (the model legume plant for genomic and post-genomic studies [8])), whereas there is only one in monocots (e.g., rice (Oryza sativa, the model cereal for genomic and post-genomic studies [9])) (Fig. 1). Seeds can be sub-categorized as endospermic or nonendospermic in relation to the presence or absence in the mature seed of a well-formed endosperm [10]. In the latter case, other structures, usually cotyledons, are the principal storage organs, e.g., as in Arabidopsis (Fig. 1). In seeds of the Amaranthaceae family (e.g., sugarbeet) a specific feature is that the maternal nucellus is not fully digested during maturation and gives rise to the central perisperm (a diploid tissue), in which starch reserves accumulate (Fig. 1). With the completion of genome sequencing projects in plants and the constitution of large EST collections for several reference and crop plants, the development of high throughput methods for gene expression profiling as transcriptomics and proteomics, allied to genetics (notably concerning naturally occurring genetic variations [11]), has become a major field of functional genomics.
The general aim of these post-genomics analyses is to understand the complex biochemical and molecular processes underlying seed quality, longevity, dormancy and vigor. In particular, several of such studies attempted to characterize the biosynthetic pathways responsible for accumulation of storage compounds in seeds [12,13], as well as their control by plant hormones, notably abscisic acid [14,15]. These reserves are of major importance for two reasons: (i) They support early seedling growth, and (ii) they are widely used for human and animal nutrition. Numerous biotechnological applications are expected toward improvement of nutritional value of seeds (e.g., increasing levels of essential compounds as vitamins, modifying lipid storage composition and structure, decreasing levels of antinutritional compounds) and for various nonedible uses (e.g., combustible fuel production or the use of seeds as a factory for the production of recombinant proteins and molecules of pharmacological interest).
Seed germination, which is a complex and multistage process [16], has also been the target of several post-genomics studies [17]. This process can be divided into three phases – imbibition, increased metabolic activity, and initiation of growth, which loosely parallel the triphasic water uptake of dry mature seeds. Morphologically, initiation of growth corresponds to radicle emergence; subsequent growth is generally defined as seedling growth. By definition, germination sensu stricto incorporates those events that start with the uptake of water by the nondormant quiescent dry seed and terminate with the protrusion of the radicle and the elongation of the embryonic axis. Upon imbibition, the quiescent dry seed rapidly resumes metabolic activity. Recent studies addressed the question of the exact requirements for germination, particularly in terms of de novo RNA and protein syntheses [18], and of the role played by plant hormones (notably gibberellic acid) acting as activators of seed germination [19,20].
Thanks to functional genomics, the past years have seen a tremendous progress in our understanding of several aspects of seed development and germination, both in reference plants and in crops. Indeed, the large amount of data demonstrates the power of descriptive biology to create novel functional insights – for example, metabolic control and redox regulation of seed developmental processes [21], mechanisms accounting for desiccation tolerance [22] or dormancy control [23].
It is anticipated that future research will bring a wealth of post-genomics data, which in conjunction with physiological and genetic studies, will provide a better understanding of the mechanisms controlling seed developmental processes. For example, the investigation of specific tissue expression of seed proteins should be undertaken to understand better, in a systems biology approach, the functioning of a whole seed from the respective roles of its constituents, namely the embryo, the storage tissues, and the seed coat (e.g., see [24,25]). Another pending question is to understand why some plant seeds remain viable for decades, even centuries, while others barely survive winter. Molecular genetics are presently used to address this specific question, which is of tremendous ecological importance for plants, and which may be of general interest in the context of understanding the basic mechanisms of aging in living organisms. Also, further attention should be paid to the mechanisms of epigenetic control of seed development [26] and germination [27]. From an applied perspective, these studies will provide novel specific markers of seed quality and vigor that can be used by the seed industry.
A public meeting on “Seeds” was held on 25 March 2008 at the Institute of France (Paris), jointly organized by the French Academy of Agriculture (www.academie-agriculture.fr/) and the French Academy of Sciences (www.academie-sciences.fr/). The aim was to highlight some peculiar and specific aspects of seed biology, based on new data gathered by genomics and biotechnological approaches. In this spirit, the topics presented by four young researchers from INRA (Nathalie Nesi, Nathalie Munier-Jolain), CNRS (Sébastien Baud) and AgroParisTech (Loïc Rajjou) concerned the mechanisms of storage compounds accumulation in seeds, the mechanisms accounting for the exceptional longevity of seeds in the dry state, and documented an example showing how this genomic information can be successfully used to create new plant varieties with improved properties concerning human nutrition and health. This issue of the Comptes Rendus Biologies presents a series of review papers directly related to oral presentations made at the meeting. These reviews cover all major aspects of seed biology, from very early seed development, to seed maturation and germination.
We are glad to invite you to explore these fascinating aspects, notably concerning some very peculiar features of embryo development and survival in plants, as well as the immense possibilities now offered to create/modify plants at a much faster rate and in a more accurate way than through domestication and classical genetic approaches, owing to the tremendous progress achieved both in plant genomics and plant biotechnologies.
This issue will start with two papers on early embryo development, describing the genes involved and notably those that are essential. Then six papers will review our knowledge on seed maturation in relation with storage compound accumulation (lipids, proteins, starch). Two papers will address the question of the mechanisms involved in the survival of plant seeds in the dry state, from both biological and biophysical perspectives. Finally, two papers will review the requirements for seed germination and seedling establishment.
We hope this present special issue will encourage a growing number of biologists to adopt plant seeds as a novel model system for integrative biology.