1 Introduction
Each major change in the human way of life has been accompanied by the emergence of new diseases or the resurgence of others [1], but in recent years the rate of epidemiological changes has escalated. In the past few decades [2], diseases have emerged (e.g., HIV, SARS, H5N1), re-emerged (e.g., tuberculosis, plague) or regressed (poliomyelitis), sometimes with local (e.g., yellow fever, leprosis) or even global (e.g., smallpox) extinction, whereas others have conquered new areas (e.g., dengue hemorrhagic fever from South East Asia toward South America, Rift Valley Fever from Africa to the Arabian peninsula).
Intensified exploitation of natural systems (e.g., agricultural practises, urbanisation, water management) has significantly changed the abundance, demography and spatial distribution of host, vector and parasite species, leading to the establishment of new host/parasite systems and hence, the emergence of new diseases. For example, during the Neolithic period, the commencement of agricultural practises led to the emergence, increased incidence or greater impact of infectious diseases, due to increased settlement size and greater potential for diseases to cross species barriers coincident with the domestication of animals [1]. Dense human populations sustained infections such as rubella or cholera, and pigs, fowl or cattle provided a steady supply of vectors and greater exposure to zoonotic diseases by facilitating transmission between wilderness and domestic environments (e.g., yellow fever, plague). These mechanisms, whereby new host-pathogen associations are formed by novel interactions, have received the greatest attention in terms of explaining the emergence of infectious diseases. In contrast, little attention has yet been paid to cases of disease emergence where all the biological components (host, potential vector or reservoir, and infectious agent) have been already present and interacting in the system. This article aims to investigate this latter scenario.
For infection to occur, two conditions must be satisfied: (i) the parasite and the host must meet frequently; and (ii) these encounters should result in parasite reproduction inside the host. For the infection to be apparent, i.e. for disease to occur, a third condition is required: (iii) parasite multiplication inside the host must cause symptomatic expression. In this paper we are particularly interested in emphasising the role played by microparasite circulation on these three conditions, thereby highlighting how mechanisms that disturb this circulation can be responsible for disease emergence (Fig. 1). The first part of the paper focuses on the first two conditions (encounter and successful transmission) which strongly relate to zoonotic pathogens; hence, we consider mechanisms in reservoir that can change the occurrence of human infection. The second part of this paper proposes mechanisms that affect pathogen expression. Symptomatic infection (the third condition) is indeed rarely addressed. When evoked, changes in infection expression are generally considered to result from a new parasite strain [3]. Here we explore alternative explanations which have, until now, been largely overlooked. Examples have been chosen from both human and animal hosts. The described mechanisms thus permit a global reappraisal of risk estimators and management measures.
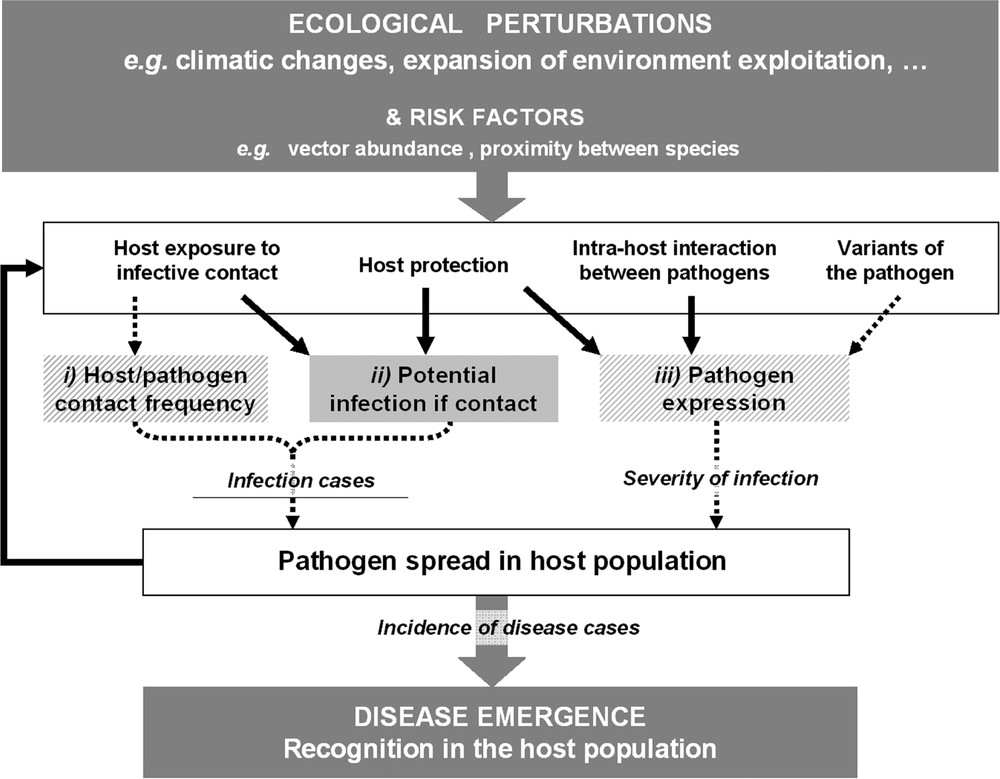
Different scenarios leading to the emergence of an infectious disease. Ecological perturbations induce changes in risk factors that in turn, through various mechanisms, result in new cases of infection or increased disease severity. The dotted arrows and hatched boxes indicate the usual explanations for occurrence of a new disease within a given host population, e.g. the presence of a virulent variant of the pathogen. The thick arrows and plain boxes highlight less-studied mechanisms which can reveal the presence of an infectious agent in a host population, e.g. interaction with another pathogen. The three conditions necessary for a disease to occur are labelled (i), (ii) and (iii) respectively (see main text). We report that increased exposure to the pathogen, due to, for example, proximity between species, has often been considered in terms of the number of host-pathogen contact events but rarely in terms of the nature of these contacts. However the latter is a crucial factor in the success of pathogen establishment – and hence, disease emergence – since it reveals how the presence of the pathogen can or cannot lead to the infection.
2 Human risk in relation to the level of environmental contamination
Nearly 75% of human infectious diseases classed as emerging are transmitted from an animal reservoir [4]. However, different host species imply different micro-environments (e.g. target cells, receptors or immune responses) to which the pathogens cannot be perfectly and equally adapted [5]. For this reason, the presence of a pathogen in the reservoir is not always sufficient for human infections to occur. Crossing the species barrier efficiently can require repeated exposure to infected disease reservoirs or vectors, and high parasite concentration during the infection [6].
2.1 Pathogen release pattern as an increased risk for human contamination
Since high parasite concentration may be required to permit inter-species transmission, the quantity of infectious agents released in the environment at any given time – which is closely related to the pathogen excretion pattern – can be determinant for the occurrence of human disease. For instance, Puumala hantavirus, which causes Nephropathia epidemica, is transmitted to humans through inhalation of aerosolized dejections of infected bank voles Myodes glareolus. As in other host-virus systems, infected individuals excrete much more virus during the first month of infection than during the following chronic phase, so the synchronicity of numerous bank vole infections episodically favours high levels of viral shedding that exceed the threshold for human infection [7]. This example suggests that prevalence of the infectious agent in the reservoir is not an adequate indicator of human disease risk, and the number of newly infected (i.e. highly infectious) animals probably provides a better estimate of the risk of human epidemics caused by aerosol-transmitted zoonotic diseases.
A similar mechanism of increased exposure to the pathogen could be involved in the occurrence of bubonic plague in humans. Using mathematical modelling, Keeling and Gilligan [8] showed that the probability of plague emergence is inversely correlated with seroprevalence against Yersinia pestis (the bacteria responsible for bubonic plague) in the rat (Rattus sp.) reservoir. When faced with infection, a rat population with low seroprevalence will reveal its vulnerability to the disease. Introduction of the bacteria then leads to a large-scale epidemic in rats, resulting in the infection of a large number of rat fleas (the main plague vector) but also in a significant reduction in the rat population. This forces infectious fleas to search for new hosts such as humans, thus transmitting to them the bacteria. Ultimately, this means that a large proportion of seronegative rats may predict a potential human epidemic, since it could precede a huge release of infectious fleas. Moreover, culling rats as a control measure after the detection of human plague cases could be counter-productive, since it would further reduce the rodent host population, promoting human infection by the infectious fleas as they search for an alternative host [8].
2.2 Reservoir dynamics resulting in human infections
Naturally the spread of a pathogen depends on the abundance of its host. Defining the conditions for human contamination also requires a better understanding of the ecological factors associated with the demographic characteristics of reservoirs. For example, Lyme disease (caused by the bacterium Borrelia burgdorferi) has re-emerged in humans in the north-eastern United States with the fragmentation of forest habitat. This is because forest fragmentation resulted in the loss of many vertebrate species, including predators and competitors of the white-footed mice (Peromyscus leucopus), the most competent disease reservoir. In areas of greatest predator and competitor disappearance, white-footed mice can reach very high densities, favouring the transmission of the bacteria to humans [9].
A change in the demography of the bank vole has also been implicated in the emergence of Nephropathia Epidemica and might explain the more restricted spatial pattern of the emergence of the disease in humans compared to the distribution of the pathogen in its reservoir [10]. In northern regions where bank vole populations show large multi-annual fluctuations, the large number of young which is produced simultaneously during years of peak density, and which is also practically concurrently infected, releases a huge quantity of virus into the environment, facilitating human infection. Conversely, more stable vole populations are unlikely to generate such a large increase in virus shedding, thus limiting transmission to humans. As a result, the virus remains undetected because it circulates at low levels within the reservoir population. A similar mechanism could be invoked to explain the restricted geography of human cases of Hantavirus Pulmonary Syndrome during the 1993 and subsequent outbreaks in the United States despite the ubiquity of the Sin Nombre virus in the reservoir species (deer mice: Peromyscus maniculatus) over an extremely wide region [11].
The sampling of areas concentrated around human disease cases in order to detect the pathogen (as is generally practised) may thus generate a biased pattern of virus distribution. Moreover, rapid change in the demography of the reservoir host, due to, for example, an increasing food supply, could lead to a rapid increase in reservoir density which can result in a peak of released virus in the environment and, in turn, to human epidemics even in former “disease-free” areas.
3 Changes in disease expression and pathogen circulation
One of the most common concepts in epidemiology is that the impact of an infection in terms of morbidity and/or mortality is greater when hosts are exposed more frequently to the pathogenic agent, thus increasing the risk of infection – in fact, most management strategies are based on this principle. Classic examples are protection against vectors (e.g., the use of bednets to protect against potentially infected vectors of malaria [12]) or vector control (e.g., [13]). Mass vaccination programs also arise from this principle: as well as individual benefit, mass vaccination provides benefits even for non-vaccinated individuals that are less exposed to the pathogenic agent (e.g., [14]). However, controlling pathogenic agents is complicated (see [15,16] in relation to malaria). As illustrated by following examples, several circumstances can enable a pathogen to pass from a subclinical or cryptic state to the emergence of a clinical expression.
3.1 The limits of avoidance strategy
Due to their short life cycles and high replication rates [17,18], pathogens are able to adapt very quickly to new environments. Preventing their circulation creates an intense selection pressure that may favour the most transmissible strains, which could also be the most pathogenic ones according to the classical virulence/infectivity trade-off assumption [19,20]. In a theoretical framework, Gandon et al. [18] have shown that mass vaccination could increase optimal virulence as a by-product of transmissibility and, ultimately, severity of cases in non-vaccinated individuals (see [21]).
However, evolutionary arguments are not the only ones that question the efficiency of management strategies. The idea that the impact of an infection is greater when hosts are more frequently exposed to pathogenic agents arises from confusion between infection and disease. This assumption is not supported in many cases because infection is not an all-or-nothing process: its severity can vary widely according to host species, infected individual characteristics or infection circumstances. The expression of an infection may not only depend on host intrinsic factors such as age (e.g. dengue severity increases with age, [22]), immune status (especially for pathogens that induce immunity after recovery), stress [23] or some genetic factors [24], but also on environmental factors such as temperature ([25,26] in myxomatosis) and/or humidity [27].
Within the host, periods of mild infection and periods of acute disease may then alternate. Intense exposure during periods of low risk of severe disease can thus be beneficial to the hosts by reducing their risk of becoming susceptible to the severe infection. Which form of infection is most often expressed then depends on how the pathogen circulates. The mechanism can be effectively illustrated using some examples.
3.2 Juvenile protection and the benefit of sustained pathogen circulation
Some pathogens may cause only mild infections when contracted early in life (Table 1) while permitting the young to initiate a protective immune response [40], which later prevents severe disease. Intense circulation of the pathogen within the host population may therefore be beneficial [41] as most young are infected early in life when they are not yet vulnerable to severe disease and, once they have been infected they are then protected if they encounter the pathogen again. A reduction in pathogen circulation, through, for example, fragmentation of the host population [42] or following imperfect vaccination schemes [43], prevents early attenuate infections, potentially leading to the (re-)emergence of the severe form of the disease. This mechanism could explain the emergence of poliomyelitis in the 1950s due to the improvement in hygiene standards [40].
Examples of host-pathogen interactions for which the infection can be more deleterious when not contracted in early-life.
Disease | Agent | Host | Biological mechanism |
Bronchiolitis | Respiratory Syncytial Viruses (HRSV and BRSV) | Mice Mus musculus [28], cotton-rat Sygmodon hispidus [29–31], calves [32] | Loss of protective maternal antibodies. |
Measles | Measles Virus | Humans | Loss of protective maternal antibodies [33]. |
Rubella | Rubella Virus | Humans | Risks for the foetus if contracted by non-immune pregnant women. |
Toxoplasmosis | Toxoplasma gondii | Humans; cats; sheep, goat, rodents, pig, cattle, birds | Risks for the foetus if contracted by non-immune pregnant women. |
Mumps | Mumps Virus | Humans | Increased risk of testicular inflammation after sexual maturity. |
RHD | Rabbit Haemorrhagic Disease Virus | Rabbits Oryctolagus cuniculus [34] | Progressive acquisition of the binding receptors for the virus. |
EBHS | European Brown Hare Syndrom Virus | Hares Lepus europaeus [35] | |
Malaria | Plasmodium falcificarium | Humans | Unknown [36,37]. |
SRAS | SRAS-CoV | Humans | Unknown [38,39]. |
3.3 Waning natural or vaccine-induced immunity
If we consider infections that induce immunity after host recovery then waning immunity has long been neglected; this is because most cases of re-infection in partly immune individuals are mild or even asymptomatic, making them barely detectable. Waning immunity can therefore be confounded with lifelong immunity [41]. Such confusion is compounded by regular immune boosts, which can maintain individual immunity at a high level. However, in the absence of contact with the infectious agent, host protection could decrease as far as total loss of immunity, leading to severe disease in the case of late re-infection. For example, African emigrants that maintained their acquired immunity as long as they stay in a malaria endemic region may contract severe malaria when they return to these countries after several years of absence [44]. As previously described, a decrease in pathogen circulation could be detrimental since it prevents recovered hosts from boosting their acquired immunity, and later re-infections would contribute to the emergence of acute disease.
Waning immunity also concerns vaccine-induced immunity [45,46], and might be involved in the resurgence of diseases in the adult population following mass vaccination of children, as presumed for pertussis [47].
3.4 Immunity at population level and outbreak risks
Disruption of pathogen circulation can reveal an infectious agent even without assuming the previous mechanism of loss of individual protection. This concerns infections inducing long-lasting immunity in the host after recovery: as long as the pathogen circulates, the population retains a high level of overall immunity, with numerous recovered hosts and few susceptible ones. Disease cases at the same time are rare and, particularly for wildlife diseases (for which detection is not as easy as in humans or domestic animals), infections could remain undetected. When this circulation is inhibited, the level of population immunity decreases as a result of host demographic renewal: recovered individuals die and are replaced by susceptible ones. Reintroduction of the pathogen into the resulting naïve population causes an epidemic which has a huge impact on the host and reveals the pathogen existence. The emergence of canine distemper in Serengeti lions (Panthera leo) in 1994 is one example of this mechanism [48].
Similarly, de Jong et al. [49] suggest that the long absence of a given HA influenza subtype in the human population, which results in the loss of “priming” immunity at the population level, could aid the rapid and extensive propagation of a virulent virus from this subtype if introduced.
In conclusion, immunity at both individual and population levels has profound effects on severity of diseases and underlies the counter-intuitive concept that severity or impact of disease might decrease with increasing transmission.
3.5 Severity of disease in the context of multiple pathogen circulation
Conventionally, diseases are studied separately: each study investigates a different infection, the pathogen being isolated from the heterogeneous context in which it exists in nature, thereby avoiding any possible interactions between pathogens. Such interactions are illustrated by the progression of AIDS, which could be delayed when people are co-infected with GB virus C [50], whereas co-infection with hepatitis B or C viruses increases the mortality of infected patients [51]. Changes in the circulation of these interacting viruses also influence the intensity of symptoms induced by HIV within a given host population. More generally, this example illustrates that either the appearance of an aggravating co-pathogen or the disappearance of an attenuating co-pathogen could reveal the presence of a previously benign infectious agent through the emergence of the disease. In the same way, modification of the pattern of pathogen circulation, following either a mass vaccination plan against a particular infectious agent or a change in the ecology of its reservoir, can reveal the presence of other pathogens.
Dengue fever can be used to illustrate the co-circulation of different serotypes of the same pathogen, and the possible consequences for the incidence and distribution of severe diseases: whatever the dengue fever serotype, the infected host generally develops benign flu-type symptoms, but in some cases the infection can lead to serious clinical symptoms such as Dengue Hemorrhagic Fever. Following the principal hypothesis (Antibody-Dependant Enhancement, [52]), the hemorrhagic form of the disease may be induced by further infections with different serotypes. The geographical distribution of the different serotypes may also give rise to the appearance of the severe form of the disease.
3.6 Pathogen interaction through changes in host demography
Pathogen interaction is not confined to within-host level. In wildlife species, numerous pathogens cause changes in the demography of their host populations, e.g., through disease-induced mortality [53]. For example, the most virulent strain of RHDV (Rabbit Hemorrhagic Disease Virus) may kill up to 90% of infected rabbits (Oryctolagus cuniculus) [54]. Epidemics of Rabbit Hemorrhagic Disease largely decrease the size and density of rabbit populations. This decrease reduces transmission of the myxoma virus, often causing a delay in the annual epidemic of myxomatosis [55]. Such a delay may have important consequences. According to Fouchet et al. [41], the impact of myxomatosis depends on the time of the year at which the epidemic occurs, depending on whether most of the young are still protected by maternal antibodies. RHDV could then increase the impact of the myxoma virus.
4 Discussion
Disease emergence can be defined as an increase in incidence, geographical spread, or the appearance of novel syndromes associated with newly or previously described pathogens (e.g., [3,56]). Until now, these novel syndromes were considered to result from the recent jumping of species barrier or via genetic variation in a pre-existing pathogen – the latter hypothesis is often suggested but not always tested. Here, we pinpoint that under certain environmental conditions an infectious agent can remain undetected over very long periods, but can be revealed in humans or animals following a change in pathogen circulation. This change may be the result of many different mechanisms including demographic change in the reservoir, host or vector; the application of mass vaccination schemes against a focal or associated pathogen; fluctuations in the frequency of contact between the host and infectious agent; or, co-circulating infectious agents.
Using examples, we have highlighted several mechanisms which may play a role in human disease risk (e.g. population dynamics of the reservoir) but also in the expression of the infection within animal or human hosts (e.g. co-circulation, juvenile protection, waning immunity; Fig. 2). These considerations should encourage a re-evaluation of the measures currently used in the control and early detection of infectious diseases. In particular, science applied to medicine (both human and veterinary) should consider an integrative approach in which the concept of a continuum of pathologies associated with any given pathogen and specific environment is universally applied. Disease management strategies should also integrate these findings.
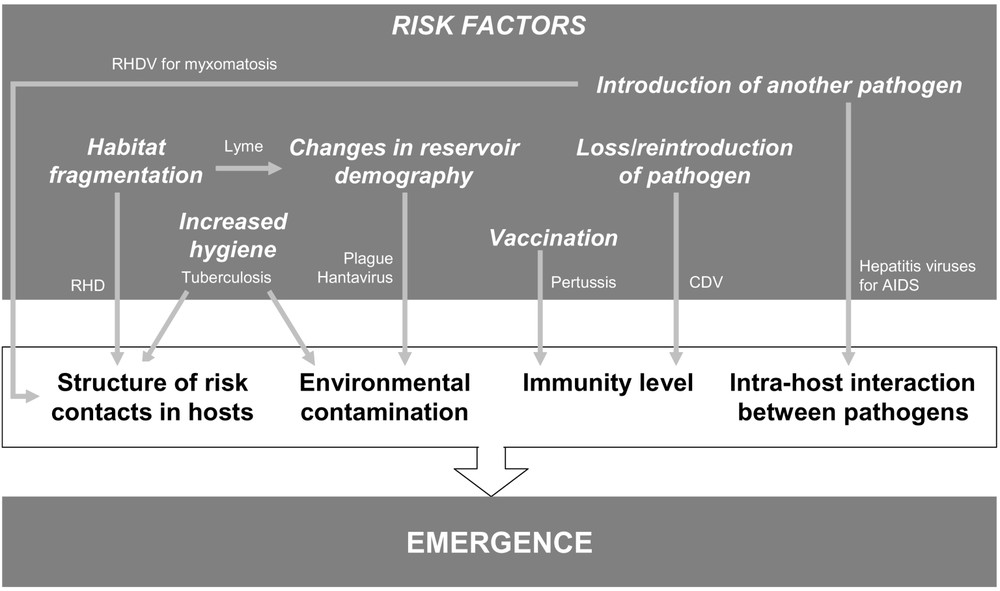
Examples of risk factors that can lead to the emergence of disease caused by an already-existing pathogen, by either increasing cases of infection or increasing their severity. The diseases or pathogens used to illustrate these mechanisms are described (see main text).
More generally, a pathogen cannot be successfully managed without an understanding of how it is integrated into the ecosystem [57]. The best illustration for this is the case of Lyme disease, for which a change in the community composition of vertebrate hosts has led to emergence of the disease in humans. The management response to disease often aims to limit the propagation of the pathogenic agent in accordance with the well-supported concept that the more a pathogenic agent circulates, the stronger its impact. However, experience shows that disease prevention is more complex [43,58]. Contracting an infectious disease involves two overlapping ecosystems, i.e. the extra- and intra-host ecosystems, both of which are continuously changing and inducing different outcomes for an infection. Particular attention should be paid to the characteristics of the host/pathogen interaction and the factors that influence them.
It is now evident that the epidemiology of infectious diseases is a complex and dynamic process, where a set of pathogens and hosts interact in a given environment. Like all biological phenomena, diseases are never static and perturbation events may alter the overall ecosystem: medicine, health policies, social and environmental changes [59], as well as the selective pressures that operate on infectious agents and on their hosts, play simultaneously on the different mechanisms described above, making infectious diseases regularly (re-)appear or decline. Analysis of the multiple causal mechanisms underlying disease emergence suggests that this process may arise due to the disruption of a dynamic equilibrium between infectious agents, their hosts and the environment. Such a disruption would lead to a new dynamic equilibrium characterised by a new set of diseases at the level of a specific host population, and by a new set of host populations infected by each infectious agent. This concept of dynamic equilibrium and its related pattern of diseases was developed by Grmek [60] who coined the term ‘pathocenosis’ to refer to ‘the ensemble of pathological states present in a specific population at a given moment in time’. This useful concept should aid our understanding of the broad contours of animal and human diseases and periodic epidemiological changes in various parts of the globe; and ultimately, allow a better understanding of how to control and prevent them.
Acknowledgements
We wish to thank the ANR SEST “Pathocénoses et émergence des maladies transmissibles : un concept unificateur mis à l'épreuve sur des pathologies exemplaires” for financial support; Ph. Barbazan, S. Bertagnoli, K. Dellagi, H. Louzir, S. Marchandeau and M. Souris for clear discussions; and J. O'Brien, J.-M. Gaillard and R. Judge for helpful comments.