1 Introduction
Character displacement refers to the phenomenon where differences among similar species are accentuated where their distributions overlap [1]. This pattern can be caused by ecological or reproductive processes. Ecological character displacement results from evolutionary change driven by competition among species for a limited resource whereas reproductive character displacement (RCD) is the divergence in sympatry of a trait involved in species recognition [2]. In most cases, the latter is expected to increase differences between parental species to avoid hybridization risk and can contribute to reinforcement in cases where gene flow persists between species [3]. RCD can affect mate coloration [4], morphological trait size [5], acoustic signals [6], olfactory signals [7] or mating preference [8].
Most studies of reproductive character displacement have focused on the evolution of either male mating cues or female preference. The fact that females generally appear to be the choosiest sex in most animals may give rise to a bias in evolutionary research [9]. However some recent studies mentioned the occurrence of RCD in male mate preferences [10–13]. Occurrence of male mate choice in species from different taxa (actinopterygians, birds, insects, mammals) and contribution of female sexual traits and male choosiness to specific reproductive isolation [14,15] may indicate that this phenomenon is more common than previously thought.
In amphibians, male mate choice has been documented in some species [16–18]. However, to our knowledge, the only literature available on reproductive character displacement in amphibian concerns male anuran mating calls [6,19–21].
Within the European newts, hybridization is commonly observed in the genus Lissotriton [22,23]. In particular, hybrids between the palmate newt Lissotriton helveticus (Razoumowsky 1789) and the smooth newt Lissotriton vulgaris (Linnaeus 1758) have been found in several locations within the sympatric zone [24–27] and introgression is documented [26]. The two species co-occur across a broad sympatric area (Fig. 1) extending from western to central and northern Europe over a thousand kilometres in longitude and latitude [28]. The two species frequently breed in the same water bodies [29] even if their ecological requirements are not identical [30]. Breeding periods broadly overlap too [31], in such a way that male secondary sexual characters are fully expressed at the same period [32]. L. vulgaris possesses a denticulate dorsal crest and flaps of skin on the toes of the hind feet. In contrast, L. helveticus has a very low straight-edged crest, conspicuous webbing between the toes of the hind feet and a filament at the end of the tail [32]. However, females of the two species look alike, although L. vulgaris female is quite slimmer, with a more pronounced ventral coloration and a more speckled throat.
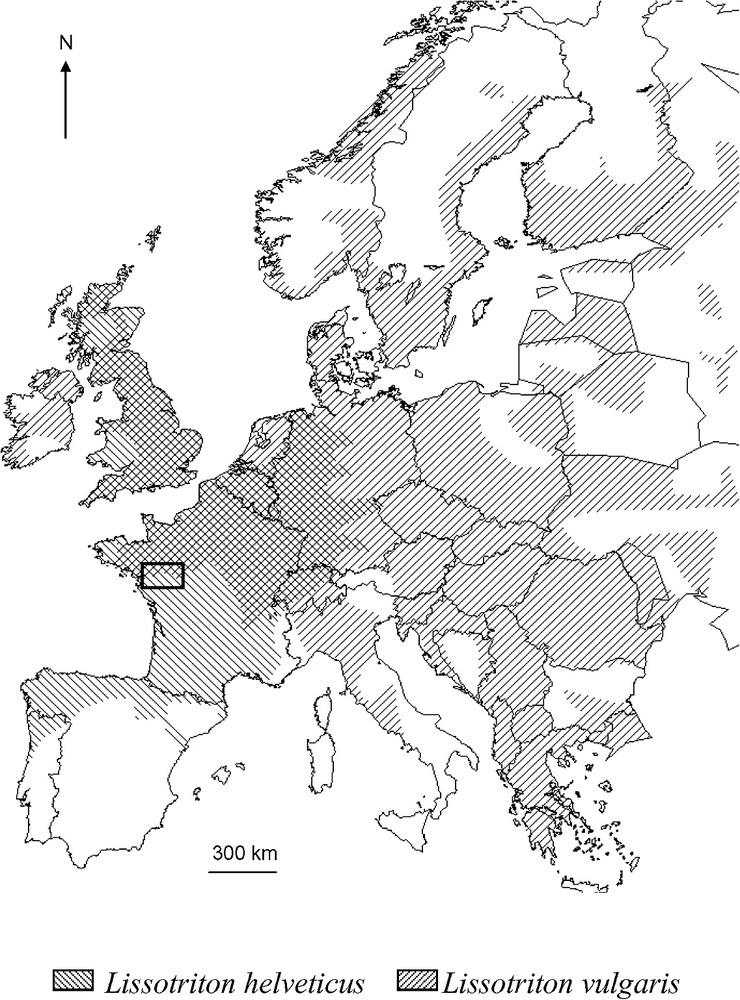
Map showing the distribution of Lissotriton helveticus (left hatchings) and L. vulgaris (right hatchings) and their overlapping ranges (from [28]). Study area is represented by a rectangle.
Because of large niche overlap and reduced hybrid fitness [33,34], we expect phenotypic divergence to strengthen the efficiency of specific recognition in syntopic sites. Interestingly, RCD could occur in males and/or females as both sexes contribute to mate choice in newts. On the one hand, females express preferences for particular males [35–38], control fertilization [39], and engage in multiple matings [36,40,41]. On the other hand, males can terminate courtship at early stages and have been shown to express mate preference for female traits [17]. In addition, sperm is probably limiting [42]. RCD in female specific traits is thus expected to limit the cost for males to mismate.
In Western France (Pays-de-Loire), the smooth newt is almost exclusively found in floodplains and its breeding habitat is entirely nested in the habitat of the palmate newt. Allotopic and syntopic sites alternate according to a mosaic pattern. This particular situation provides an opportunity to test for the occurrence of reproductive character displacement on a small geographical scale. To detect RCD, we took morphological measurements on males and females of the two species reproducing in ponds in this area. We tested whether the presence of L. vulgaris in the pond influenced L. helveticus morphology. Golberg and Lande [43] pointed out that the majority of studies focusing on character displacement did not include environmental data, which increased the risk of failing to detect divergence. Our earlier study suggested that terrestrial habitat could influence adult morphology [44]. Thus, we integrated relevant environmental co-variables in our models to discriminate between phenotypic differences caused by syntopy and those caused by environmental factors.
2 Materials and methods
2.1 Sampling and morphometry
We sampled Lissotriton helveticus and L. vulgaris in Pays-de-Loire (Western France) in the floodplains between Gennes and Nantes and the surrounding plateaus (Fig. 2). Newts were caught in ponds during their aquatic phase from March to May 2006. The elevation of sites ranged from 8 to 110 m. We captured as many sexually mature adult newts as possible from each pond using dipnets (Capture and release Permit delivered by Préfectures du Maine-et-Loire et Loire-Atlantique). On average, thirty individuals per population were caught from twenty-seven ponds. All newts were anaesthetized prior to manipulation by immersion in Tricaine methane sulphonate (0.2 g/l), MS222, for a duration of 5 to 10 minutes. Measurements were taken the same day or the day after capture. Six characteristics associated with size and developments of secondary sexual traits were measured to the nearest 0.01 mm using a digital calliper. We measured the following traits likely to discriminate the two species: snout to vent length (SVL), head width (HW), tail height (TH), maximum tail height (MTH), cloaca width (CW) and male palmate filament length (FIL) (for details on the measurement method, see [44]). Individuals were subsequently released to their original capture sites.
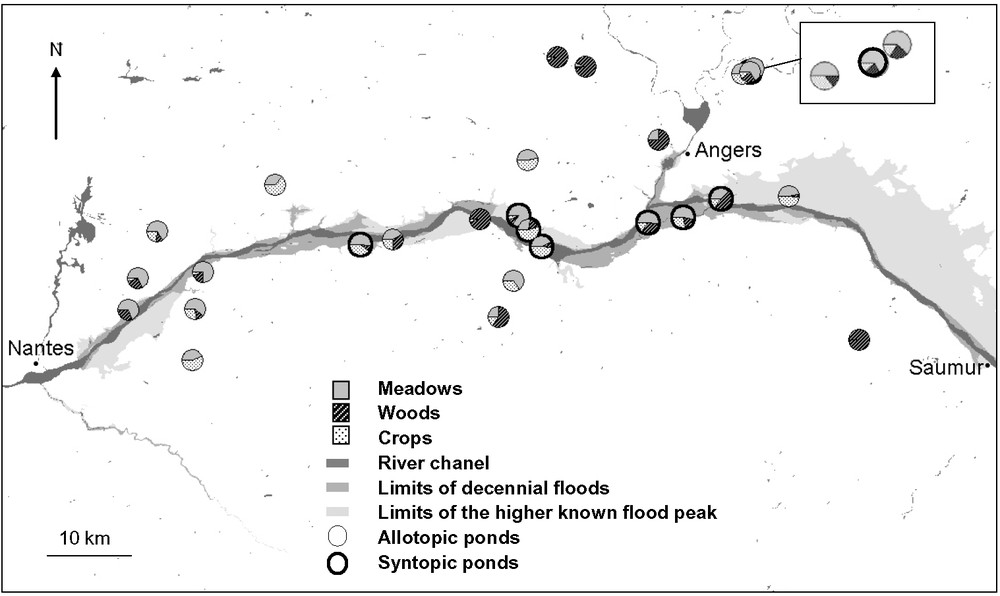
Localization of the 27 populations of Lissotriton helveticus sampled in western France in allotopy and in syntopy with L. vulgaris (dark circled pie chart) with emphasis on landscape covered (only the three majority habitats are shown in the pie chart) and flood risk (BD CARTHAGE ®©IGN-MATE 2005).
2.2 Habitat characterization
We quantified land characteristics within a 400-m radius centred on each pond. This distance corresponds to the average migration distance in newts (see [45]). Terrestrial habitat conditions the amount of light as well as water and vegetation characteristics observed in ponds. We thus measured the area covered by meadows, forests, crops, roads, habitations, streams and pools from aerial photography using the Geographic Information System software MapInfo 6.5. Data were given in percentages for each element of landscape cover. We observed that L. vulgaris mainly occurred in ponds close to the river channel. Thus, we integrated information about flood risk in our analyses in order to control for the potential effect of this factor. The occurrence of floods has been studied categorizing ponds within three classes using the French national database Cartorisque [46]. We determined whether ponds were subjected to decennial floods, or within the limit of the highest known flood peak. Finally, ponds on the plateau were considered as never flooded.
2.3 Statistical analyses
Our procedure is simpler than that of Adams and Collyer [47] because one species does not occur in allotopy within the study area. To investigate the effect of syntopy and environmental variables (percentage of each element of the landscape cover and level of flooding) on Lissotriton helveticus morphological variation, we used Linear Mixed-Effects Models with these factors as independent variables. LM present several advantages over classical regression approaches in that they integrate data from different statistical distributions with the appropriate modelling statistical error. It allows continuous variables (e.g. % of forest) and/or categorical variables (e.g. presence of L. vulgaris). It also permits to add random effects which could not be taken into consideration with basic statistics (like t-test, discriminant analysis, …). Because of the hierarchical structure of the dataset, two nested random effects were included in each model: sampling site nested in the sampling date. Sampling date was included in the models because adult body size increases throughout the aquatic breeding period [32,44], whereas sexual traits grown for the breeding season regress afterwards [32]. As we compared individuals from different ages and because growth is continuous in this species, all morphological traits were converted to relative size measurements by dividing each trait by SVL. Normality assumptions were met for all dependent variables. Each morphological character was tested in a separate model. Each sex was handled independently because of sexual dimorphism. From every complete model, we performed a backward selection, removing, at each step, the least significant factor at the threshold
3 Results
A total of 446 females and 357 males of L. helveticus and 83 females and 43 males of L. vulgaris were sampled in the 27 ponds. Eight ponds were syntopic. As we found no allotopic populations of Lissotriton vulgaris, we could only determine the effects of syntopy on L. helveticus morphology. We detected a significant effect of population and date on all morphometric traits measured on the palmate newt. Morphological variation was explained both by environmental variables and syntopy with L. vulgaris. Table 1 presents the minimal models retained for each morphological trait and each sex. For clarity, non-significant models are not shown.
The effects of environmental variables and syntopic situation on morphological data for male and female palmate newts using Linear Mixed-Effects Models, which includes two nested random effects (sampling site in sampling date). Minimal models were backward selected. denDF: denominator degrees of freedom for F-test.
Parameter estimate | SE | denDF | F-value | P-value | ||
Female | ||||||
Model 1 | SVL | |||||
(Intercept) | 34.2637 | 0.4415 | 419 | 6022.33 | <0.0001 | |
% Forest | 0.0217 | 0.0063 | 22 | 11.77 | 0.0024 | |
Model 2 | Head width | |||||
(Intercept) | 0.2119 | 0.0011 | 419 | 24802.55 | <0.0001 | |
% Forest | −0.0002 | 0.0000 | 22 | 34.31 | <0.0001 | |
Model 4 | Tail height | |||||
(Intercept) | 0.1572 | 0.0023 | 352 | 4743.91 | <0.0001 | |
% Forest | −0.0001 | 0.0000 | 18 | 10.74 | 0.0042 | |
Model 5 | Maximum tail height | |||||
(Intercept) | 0.2145 | 0.0045 | 338 | 4022.596 | <0.0001 | |
% Forest | −0.0004 | 0.0001 | 17 | 10.397 | 0.0050 | |
Model 5 | Maximum tail height | |||||
(Intercept) | 0.1988 | 0.0043 | 338 | 3315.243 | <0.0001 | |
Situation[syn] | 0.0182 | 0.0077 | 20 | 5.627 | 0.0278 | |
Male | ||||||
Model 6 | SVL | |||||
(Intercept) | 29.8614 | 0.2663 | 330 | 12572.00 | <0.0001 | |
% Forest | 0.0159 | 0.0051 | 22 | 9.71 | 0.0050 | |
Model 7 | Head width | |||||
(Intercept) | 0.2150 | 0.0012 | 330 | 29636.04 | <0.0001 | |
% Forest | −0.0001 | 0.0000 | 22 | 16.53 | 0.0005 | |
Model 9 | Tail height | |||||
(Intercept) | 0.1525 | 0.0024 | 284 | 4128.12 | <0.0001 | |
% Forest | −0.0001 | 0.0001 | 18 | 6.08 | 0.0239 |
Males and females responded in the same way for each environmental parameter taken into consideration. According to the coefficients given by Model 1 and Model 6, body size (SVL) increased with forest cover (females:
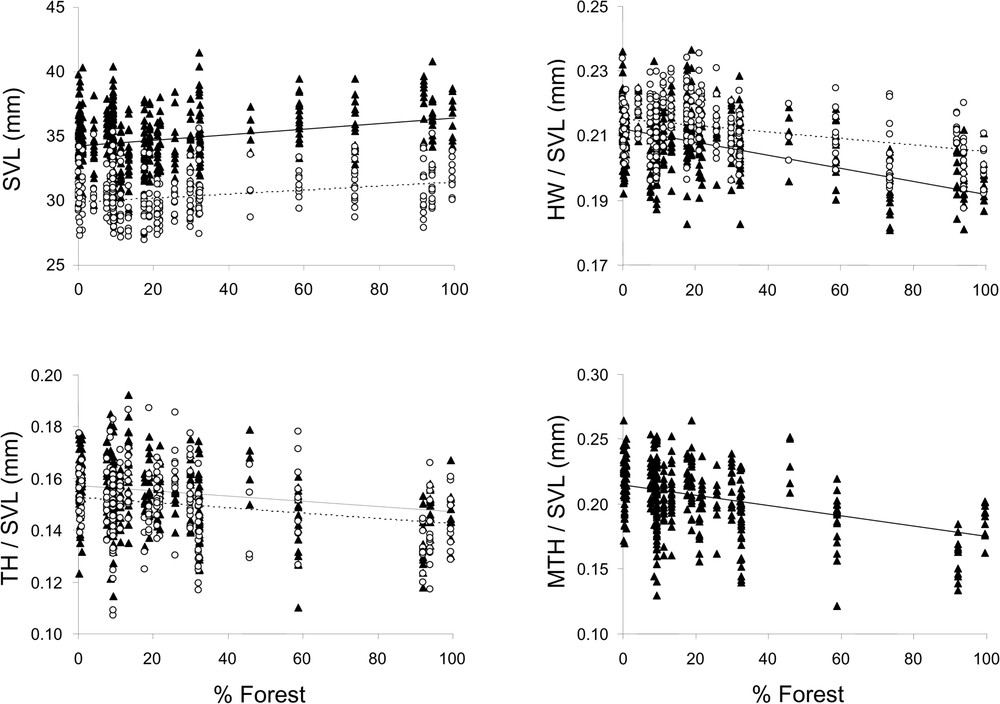
Relationship between environmental variables and morphological traits found in female (▴) and male (○) L. helveticus. The continuous line corresponds to females and the dotted line to males. Linear regressions were calculated from the Linear Mixed-Effects Model including two random nested effects; sampling sites in sampling dates (see Table 1 for statistical results).
We observed a significant effect of syntopy on female morphology. According to the positive coefficient given by Model 5 (value = 0.0182), maximum tail height was larger in syntopy (
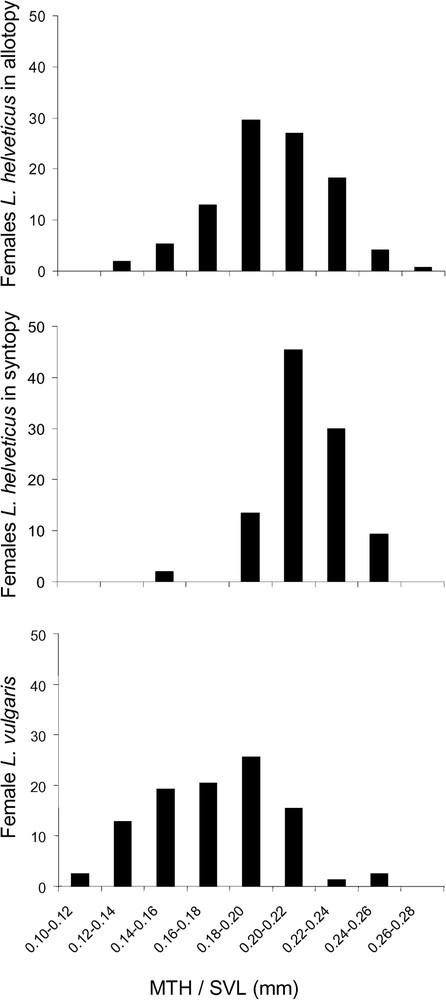
Distribution of measurements for maximum tail heights (divided by SVL) found in females of L. helveticus (in both allotopy and syntopy) and L. vulgaris. The Y-axis represents the frequency of individuals inside each group.
4 Discussion
Natural selection, sexual selection as well as random genetic drift can cause phenotypic differentiation between populations. Recently, several authors have emphasized the importance to include relevant environmental co-variables when investigating the phenotypic consequences of interspecific relationships [43,47]. The large ecological amplitude of the palmate newt [49] clearly required to follow these guidelines. We thus explicitly integrated spatial variation with environmental factors to test for the occurrence of character displacement.
4.1 Effects of landscape on body size and shape
We detected a significant effect of landscape on the size and shape of both males and females. Snout-to-vent length was positively correlated with the proportion of forest cover surrounding the pond in both sexes. Body size was larger in forested than in more open habitats, which confirms the trend observed previously on a smaller sample of sites in the same study area [44]. Earlier studies reported effects of terrestrial habitat on Lissotriton helveticus morphology [50] and abundance [45] suggesting that habitat quality affects growth and survival. Both are expected to be higher in forested than in open habitats because of better foraging efficiency, higher shelter availability [49,51] and lower water temperature during larval development. Temperature is negatively related to the size at metamorphosis that is a good predictor of adult size [52–54]. Unfortunately, there is no available data to evaluate the relative contribution of these factors to morphological variation.
We also found that body shape was negatively correlated with the percentage of forest cover. Relative head width and tail height for both sexes, as well as maximum tail height for females were smaller in forest. Water is commonly darker in forest than in open habitat because of lower light intensity and a higher concentration in organic matter and humic acids [55]. Secondi et al. [44] found that in Lissotriton helveticus male sexual traits decreased with water turbidity suggesting that the expression of visual signals matched the local conditions of signal transmission. We can hypothesize that although shape can be important in species recognition, characters contributing to it like sexual characters would be less developed when visual communication is less efficient.
4.2 Reproductive character displacement
In addition, we detected a significant effect of syntopy on palmate newt morphology. Female L. helveticus developed a higher tail when in syntopy with L. vulgaris. Since female L. vulgaris posses a slimmer tail than females of the other species, morphological divergence in syntopy is consistent with the hypothesis of character displacement.
Ecological character displacement is considered as the main factor explaining differences in body size [43]. However, it could be discarded here for several reasons. The breeding period of Lissotriton helveticus and L. vulgaris extensively overlap [31]. The two species also frequently breed in the same water bodies [29] even if their ecological requirements are not identical [30]. Yet, earlier work suggested that competition for food resources is relaxed between aquatic adults of the two species [56]. In general, species of similar body size avoid competition by occupying different geographical regions or different ponds within a region [57], which is not the case for the two species studied here. The large niche overlap of adults suggests that interspecific competition is not important, possibly as a result of resources like food or vegetation stems used for laying are not limiting. In addition, if character displacement is due to competition, it is likely that males would be also affected, as both sexes are usually found in the same area of the pond [29] and have the same diet [56]. We cannot definitively discard the fact that a confounding factor, such as water quality, density of individuals or presence of predators is responsible for morphological variation between syntopic and allotopic ponds, even if this alternative hypothesis does not fully explain why females react and males do not.
In contrast, experimental crossings revealed selection against hybrid helveticus x vulgaris [33,34,58] and thus a cost to hybridize in these species. Because females are very similar, a divergent change in body shape is likely to enhance species recognition by males. Reproductive character displacement seems therefore the most plausible explanation for the apparent divergence. Even if males develop exaggerated epigamic traits displayed during courtship, they seem to actively select females too. They do not engage automatically in courtship and often interrupt interactions with females [59]. Roberts [59] consistently suggested that males of palmate newts were more efficient in specific recognition than females when using visual cues only. Consistently, our result suggests that heterospecific matings are costly for male newts, which is possibly caused by the waste of time, energy and gametes in low quality reproduction. This last point may be particularly crucial in a group where sperm is limiting [36]. The smooth and the palmate newt produce complex sequences of courtship display that only show few quantitative differences [60] and interspecific courting is common [59, Secondi unpublished data]. Differences in male specific recognition between syntopy and allotopy could thus contribute to enhance reproductive isolation. Additional data on interspecific courtship behaviour and male response to tail height would thus provide a strong experimental test of the RCD hypothesis.
4.3 The spatial scale of reproductive character displacement
Most reproductive character displacements are found in studies comparing allopatric and sympatric populations. Although conclusive this approach is not informative about the scale at which divergence can occur. We found here a pattern of character displacement inside the area of sympatry, between syntopic and allotopic sites. This would imply that gene flow between adjacent populations is sufficiently low to maintain phenotypic differences under selection. Amphibians, and newts in particular, are known to be highly philopatric [61] and to exhibit relatively poor dispersal ability (see [45]). These characteristics tend to limit gene flow between populations and to generate spatial structure at small geographical scales. To this regard, a significant genetic structure (i.e. Fst) has been observed from 100 m in Rana sylvatica [62], to less than 1 km in Hyla arborea [63] or 2.8 km in Bufo calamita [64]. Adaptations are more easily fixed when genetic structure is strong [65,66]. Thus, it may be not surprising that examples of local adaptation are described in amphibians even between close breeding sites (e.g. [67–70]). The local divergence of male mating calls of Hyla cinerea in ponds where H. gratiosa breeds syntopically [6] tends to support this idea. Additionally, cases have been reported where local adaptation in sexual behaviour persisted despite high gene flow [11,71].
5 Conclusion
To conclude, our results suggest that reproductive character displacement can occur within a sympatric zone. Studies of RCD at larger geographical scale can fail to detect character divergence because both environmental and genetic gradients affect phenotypic variation. It may be easier to detect this phenomenon when considering smaller scales for species with limited dispersal abilities. In spite of the likely occurrence of gene flow, our study tends to show that adaptation in small scale mosaic zones occurs in syntopic sites. Studying local adaptation is a fundamental point to determine the relative effect of selection over gene flow and other evolutionary forces i.e. it should help to a better knowledge of forces modelling evolution of species.
Acknowledgements
We thank S. Sourice and V. Hardouineau for technical assistance, J. Dobigeon, T. Duret, R. Robin and E. Beslot for their help in the field and during morphometric measurements. We also thank the Pr. John Endler as well as an anonymous reviewer for helpful comments and critical improvements on the manuscript. We are thankful to Mark Phipps for grammatical corrections on the manuscript. This study was funded by Région Pays-de-la-Loire, DIREN Pays-de-la-Loire and Angers Loire Métropole.