1 Introduction
The unsaponifiable matter constituent of almond seed oil ranged from 0.3 to 3 wt%. However, it is a source of information on its characterisation and authentication. In fact, the unsaponifiable fraction contains a variety of bioactive substances, which include sterols, hydrocarbons, tocopherols terpenes and others. These minor compounds are more characteristic of each fat and oil, and hence more useful in authenticity tests [1–4]. In addition, it has been reported that phytosterols, which represent the predominant portion of unsaponifiable matter, have multifunctional properties, including anti-inflammatory, antitumor, hypocholesterolemy, antiulcerative, antifungal and antibacterial activities [5–7]. In fact, 1 to 3 g of phytosterols per day is currently considered the amount needed to significantly lower the level of serum cholesterol [8,9]. Several studies have specifically reported that consumption of almonds reduces total and LDL cholesterol in plasma [10,11]. The lowered cholesterol associated with nut consumption has been attributed to the replacement of saturated fat with mono-unsaturated fatty acid (MUFA) [12]. There are several compounds in nuts in addition to MUFA that have the potential to affect plasma cholesterol, including dietary fiber, phytochemicals, sterols and possibly arginine [13,14]. Others authors [15] mentioned that almond constituents, including α-tocopherol, unsaturated fatty acids, phytosterols, dietary fiber and polyphenolic compounds are well recognized for their cholesterol-lowering properties. Eric [16] reported that the consumption of saturated fats increases the risk of cardiovascular diseases by 5% when compared to the consumption of carbohydrates; whereas the consumption of MUFA decreases the risk coronary diseases by 19%. The consumption of polyunsaturated fats decreases the risk by 38%.
Many studies on the occurrence and distribution of sterols triterpens alcohols, and other unsaponifiable constituents of vegetable oils have been reported [17–20]. The sterol composition showed little variation between subspecies and collection sites; however, different harvesting dates showed significant effects on the levels of accumulation of some sterols both in the seeds and in the pulp of some plants [21]. Besides, the profile of sterols is different in all parts of plant and it changes during plant development [22,23]. The levels of total phytosterols in the oil of some seeds ranged from 1.4 to 4.53 wt% [24–26]. However the amount of total sterols present in almond oil ranged from 0.27 to 0.28 wt%. Although many other studies have been published on the analysis of unsaponifiables from vegetable oils, many of them remain obscure and need further investigation.
Even so, no detailed study has been conducted to determine the relationship between the unsaponifiable fraction of almonds, such us sterols and the compounds of the glyceridic fraction, in particularly unsaturated fatty acids.
The aim of the present study is to identify and compare differences among the three cultivars of almond: Tunisian (Achaak), American (Perlees) and Italien (Mazetto) and monitor changes in their seed contents and composition of: sterols, squalene and unsaturated fatty acids during the harvesting period.
The first axis of this study is to examine the difference of phytosterols composition between cultivars. The second is to delineate the sterols profiles during maturation, which are useful for the characterisation of almond oil. The last one is to try to understand the biosynthetic relation which could be existing between cholesterol, 24-methylene cholesterol and linolenic acid.
2 Materials and methods
2.1 Chemical reagents
Analytical grade solvent: petroleum ether, ethanol, diethyl ether, hexane, chloroform and silica gel TLC plates (silica gel 60, , 0.25 mm thickness were purchased from Merck (France)), bistrimethylsilyltrifluoroacetamide (BSTFA) was purchased from Pierce Chemical Company (USA), pyridine from BDH Inc., Toronto (Canada). Standards of sterols, hydrocarbons and 2.7-dichlorofluorescein were supplied by Sigma Aldrich (USA).
2.2 Sampling
Three cultivars of almond (Prunus amygdalus) were cultivated in the same experimental conditions in station GOVPF (Grombalia, Tunisia): American (Perlees), Italian (Mazetto) and Tunisian one (Achaak). The fruits of each cultivar were collected every week, after 40 days of flowering, until complete maturation.
2.3 Analytical method
The isolated sterols and hydrocarbons were analysed by a Hewlet-Packard GC 5890 series II (USA) equipped with a flame ionization detector and a split/splitless injector. The column was a DB-1 (1%-phenyl)-methylpolysiloxane) 0.25 mm length with 0.25 μm film thickness, purchased from J&W Scientific (Ontario, Canada) for analysis sterols and DB-5 (5%-phenyl-methylpolysiloxane) length with 0.25-μm film thickness, for analysis hydrocarbons.
The DB-1 column used for analysis sterols, was kept at 200 °C, and then was programmed at 3 °C/min to 300 °C for 2 min under flow controlled conditions. The injector was at 305 °C and detector at 350 °C; this programme was controlled by computer view sonic graphics series GT775 equipped with HP GC ChemStation Rev.A.06.03. The DB-5 used for analysis hydrocarbons was kept at 60 °C for 3 min, and then it was programmed to be worked at 5 °C/min to 300 °C and to be kept for 1 min. This programme worked under the same computer.
The GC HP 6890 series GC system (USA) was coupled with an Agilent 5973 quadripole mass spectrometer, the whole was connected to a view sonic computer graphics series GT775 equipped with Enhanced ChemStation G 1701 CA version. Helium was used as a carrier gas, 3 μl of each sample was injected, the rate of the carrier gas was 1 ml/min; the oven temperature and the injector have the same temperature in the GC-FID. Electron impact ionisation at 70 eV was used. The source temperature was 230 °C.
2.4 Extraction of the unsaponifiable fraction
Lipids were extracted by using a soxhlet extractor with petroleum ether, during 4 h. 5 g of oil were dissolved in ethanol potassium hydroxide (2 M) and kept at light boiling during 1.30 h; and then the unsaponifiable were extracted four times by petroleum ether and washed four times by ethanol (50%). The washes were added to the unsaponifiable fraction. The fraction collected was concentrated in the rotary evaporator at 60 °C, and then recovered in 1 ml chloroform. The unsaponifiable fraction in the oil was expressed in weight percent. The unsaponifiable were separated into different classes on 0.25 mm silica gel plates (). The mobile phase used was a solvent mixture of hexane/diethyl ether 60/40 by V.
2.5 Fractionation and isolation of unsaponifiable classes
A chloroform solution of the unsaponifiable matter was then applied uniformly along a line 1.5 cm from one edge of a plate coated with a 0.25 mm layer of silica gel and developed with hexane/diethyl ether 60/40 by V. After the development, the plates were sprayed with a 0.2% ethanol solution of 2.7-dichlorofluorescein (w/v) and observed under UV light (254 nm). Six different zones were marked and named a, b, c, d, e and f; and separated according to their order of elution. Fractions a and f according to ascending order of elution were respectively attributable to the sterols and hydrocarbons. Each zone was carefully scraped from the plates and extracted with diethyl ether and the solvent was removed under current of nitrogen.
Sterol compounds (fraction a) were silylated with 50 μl of bistrimethylsilyltrifluoroacetamide at 65 °C for 3 h. The residue obtained was mixed by 50 μl of chloroform and identified by GC-MS and quantified by GC-FID. The twelve sterols identified were named respectively in order of their relative retention time (RRT): A, B, C, D, E, F, G, H, I, J, K and L. Hydrocarbons compounds (fraction f) were injected directly without any treatment.
3 Results and discussion
3.1 Sterol identification
For the purpose of identification and quantification of sterols, we used two complementary chromatographic techniques; GC-FID and GC-MS. The latter allowed the determination of the empirical formula of the studied compounds as well as the determination of principal characteristics of cleavages sterols were identified as trimethylsilyl derivatives (TMS) by both their RRT in GC and their MS fragmentation by GC-MS analysis. The study of the spectra of silylated form of these compounds allowed the determination, without any ambiguity, the tetracyclic structure of the ring as well as the position of the intracyclic double bonds. Indeed some cleavages characteristics of the Δ5 sterols were found, in particular peaks 129 and M-129 resulting from allyl cleavages bonds 1–10 and 3–4 with hydrogen transfer atom. The common major ions were (Table 1): M-CH3, C3H3-TMSiOH, M-TMSiOH, M-TMSiOH-SC and M-TMSiOH-SC-42 + H, (SC: side chaine; TMS: trimethylsilyl; M: molecular ion).
Principal fragments of major saturated (H), monounsaturated (D, G) and diunsaturated (E) sterols of almond oil obtained by MS.
Sterols (TMS ⁎ ) | Molecular ion | Principals cleavages | ||||||
M | M-CH3 | C3H3TMSiOH | M-TMSiOH | M-TMSiOH-CH3 | M-C3H3TMSiOH | M-TMSiOH-SC+H | M-TMSiOH-SC⁎-42+H | |
D | 472 | 457 | 129 | 382 | 367 | 343 | 255 | 213 |
E | 484 | 469 | 129 | 394 | 379 | – | 255 | 213 |
G | 486 | 471 | 129 | 396 | 381 | 357 | 255 | 213 |
H | 488 | 473 | – | 398 | 383 | 359 | 257 | 215 |
⁎ TMS: trimethylsilyl, SC: side chain.
The diagram shown in Fig. 1 describes the main common cleavages of sterols. Indeed, the ions of mass 255 (M-SC-TMSiOH) and 257 (255+2H) allow the determination by difference with molecular mass the empirical formula of the side chain as well as the determination of the series to which each one of these compounds belongs. Sterols A, D, G and H have a saturated side chain and they belong to series of cholestane (A), ergostane (D) and stigmastane (G, H). Analysis of their mass spectra allowed the identification A, D, G and H respectively as cholesterol, campesterol, β sitosterol and stigmastanol. Sterols C and E have a mono-unsaturated side chain and thus belonged respectively to the series of ergostane and stigmastane. The analysis of mass spectra permitted the observation a cleavage of the side chain, the most likely position of unsaturation and the presence of methyl groups.

Diagram of the principal common cleavages of trimethylsilyl derivatives of sterols of almond oil.
The different ions obtained following the principal cleavages, illustrated in Table 1 were observed in all saturated, mono and diunsaturated sterols except the peak 129 which was missed in saturated sterols (H). The peak of M-C3H3TMSiOH was missing in sterol with an unsaturated side chain (E). The peak of M-TMSiOH-CL-42 + H corresponded to mass 213 was presented in all unsaturated sterols. The peak of mass 215 is characteristic of saturated sterols. Moreover, the saturated sterols were distinguished from the others by the ions of mass 230 and 305 [27]. The peak of mass 255 was presented in all unsaturated sterols. The peak 257 (255 + 2H) is specific for saturated sterols. However, these peaks 255/257, were irregularly indicated in the literature [28]. If the unsaturation is in position 5, ions of respective masses M-211, M-129 and 129 are typical. These ions are very intense and they often represented peak 100. When unsaturation is in position 7, ions 345 and 299 accompany the common ions. Thus, peak 100 belongs either for molecular ion M or for ion of mass 255/257 [28].
For the compound C (M = 440), the major peaks are those with ; ; (SC). Thus sterol C showed particular peak, different from those of saturated and mono-unsaturated sterols. Furthermore, the absence of peaks 129 and M-129 characteristic of the Δ5 sterols and peaks 345 and 229 characteristic of the double bond in position 7, allowed to one conclude that there is an acetate sterol having two double bonds. In this case, when the unsaturation of the ring is in 5, the ion of mass M-60 allowed the determination the nature of the side chain, as well as the specification whether the ion is in mono or bi-unsaturated series. The position of a second double bond can be determined by the value of peak 100. Indeed, if the peak 100 is the major, the second double bond is in position 22. On the contrary, if the cleavage corresponding to mass 296 is major, the second double bond is in position 24 (28). In our case the ions of mass 380 = M-60 (peak 100) and 255 are majors, which suggests that the double bonds are in positions 5 and 22.
The presence of cholesterol, which for many years was considered to occur only in animals [29], was definitively confirmed; its principal cleavages given by MS are: 443, 129, 368, 353, 329, 255 and 213. However, its level is low and it undergoes more or less significant variations.
3.2 Sterols composition during development
This study showed the presence of 12 sterols which are in the in ascending order of their RRT: cholesterol (A), 24 methylene cholesterol (B), (R) ergosta 5, 22-dienol, acetate (C), campesterol (D), stigmasterol (E), stigmastadiene-5, 25 ol 3 (F), β sitosterol (G), stigmastanol (H), Δ5 avenasterol (I), 5-24 stigmastadienol (J), Δ7 stigmastenol (K), Δ7 avenasterol (L). The major phytosterols are by descending order: β sitosterol, stigmastanol, campesterol and stigmasterol.
β sitosterol is strongly presented in all stages of maturation for all cultivars (Fig. 2M). Furthermore, the behavior of this compound remained rather unusual. It marks fluctuations during the first stages of maturation, and then it decreases enormously during the last harvesting period of cultivar Achaak. However, for cultivars Perlees and Mazetto, the β sitosterol level decreased considerably at the 10th and 12th week during maturation and passed from more than 1100 mg to less than 50 mg/100 g TL (Fig. 2M). Afterwards its accumulation is restored gradually during the maturation period. Stigmastanol evolved similarly to β sitosterol, but with a lower amount.
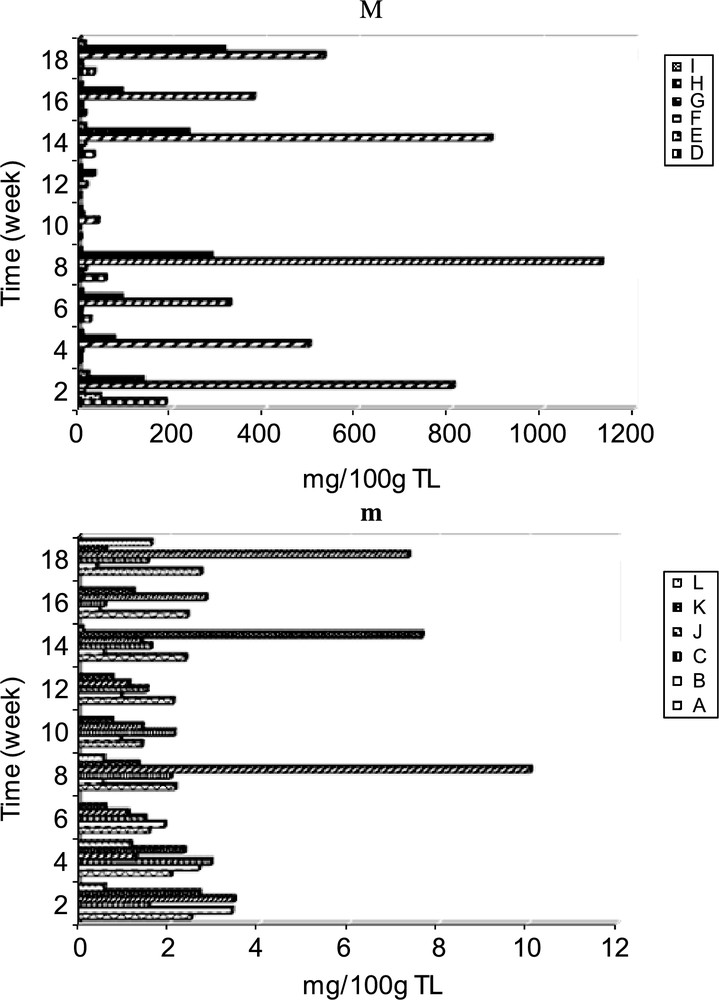
Evolution of majors (M) and minors (m) sterols composition of cultivar Perlees. Time indicates weeks after the 40th day of flowering.
Thus, almond oil is rich in β sitosterol followed by stigmastanol, campesterol and in lesser amount stigmasterol, stigmastadiene-5, 25 ol 3 and Δ5 avenasterol. For most samples, the content of the other sterols was lower than 10 mg/100g TL (Fig. 2m). The Δ5 sterols (I and J) are accumulated with higher amounts (21.53 mg) than those of the Δ7 sterols (K and L) (3.24 mg) at the 2nd week of maturation of cultivar Mazetto. The latter are presented in prunoid oil at traces, contrary to rosoïd oils which contain significant proportion of Δ7 sterols. This was in agreement with the results of others authors [30] by the fact that some higher plants which contain Δ5 sterols often have small amounts of Δ7 sterols which are presumed to be precursors. For the cultivar Achaak, the evolution of Δ5 avenasterol content presented particular variation. Indeed, at the beginning, this compound evolves parallel to the level of those of β sitosterol and stigmastanol, and then reached its maximum value when the lipogenesis is the strongest (13.41 mg at the 10–12th weeks). However for the cultivars Perlees and Mazetto, the maximum level of this compound is observed in early stages of maturation (Perlees: 9.61 mg; Mazetto: 18.08 mg at the 2nd week). The ergosta 5.22 decreases significantly during maturation for cultivars Achaak and Perlees. Quantitative analysis shows that total sterols decrease significantly at the 10–12th of the maturity of three cultivars (Fig. 3). These variations particularly affected the β sitosterol and stigmastanol levels. The decrease of phytosterols during intermediate phases (Fig. 2M) is probably due to a considerable plant needs for these compounds in physiological processes such as their implication in the membrane structure, production of cellular organs or precursors for hormones and vitamins production [16].
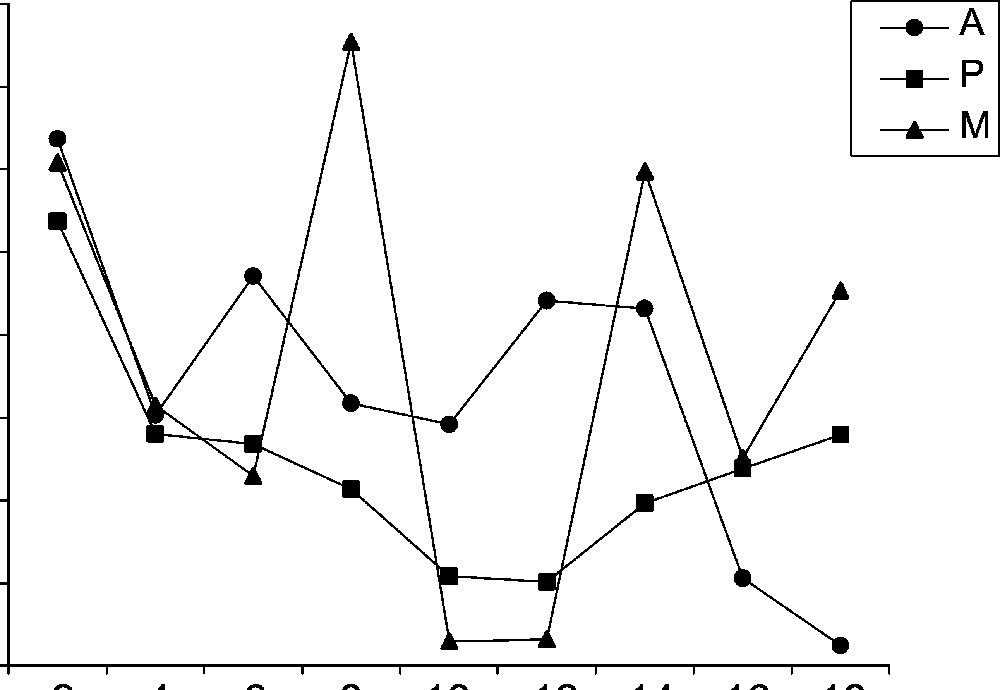
Time course of the total sterol profile for three cultivars (Achaak, Perlees and Mazetto) during maturation. Time indicates weeks after the 40th day of flowering.
3.3 Relationship between sterols D, E and F
The campesterol (D), stigmasterol (E) and stigmastadiene (F) evolved by the same way for the three cultivars. These compounds are presented at high levels at the early stage of the lipogenesis which then decrease gradually during maturity. This accumulation coincides with the maximum of the lipase activity during the maturation. At the end of maturation the level of D, E and F increased for cultivars Perlees and Mazetto and decreased for cultivar Achaak.
Based on these observations, we can formulate the following assumptions:
- (1) Biosynthesis of campesterol (D), stigmasterol (E) and stigmastadiene (F) is governed by the same enzyme (E1) and probably has the same biosynthetic precursor;
- (2) The activity of the enzyme E1 is proportional to the activity of the fatty acid gras synthase in almond kernel. This is may be confirmed by the diminution of D, E and F compounds at the end of maturity of the cultivar Achaak. However, the re-establishment of synthesis of these compounds during the last stages for the cultivars Perlees and Mazetto makes this assumption into question. This paradoxical phenomenon could be explained by: (a) the synthesis of novo of these compounds which thus maintain an enzymatic activity for a rather long time or; (b) the Conversion of other terpenoid or phytosterol compounds into sterols D, E and F during the last phases of the lipogenesis.
It is known [31] that β sitosterol can undergo a dehydrogenation due to the Δ5 desaturase which produces stigmasterol and that campesterol and stigmastadiene possesses a common precursor which is methylen-4–lophenol. This precursor leads to the formation of campesterol (D) via reduction route of NADPH, whereas methylation and migration of one of its double bonds gives stigmastadiene (F). However, it is unknown whether there is a conversion of other phytosterols or terpenoids into these compounds.
3.4 Relation sterol-squalene during maturation
Living cells are able to carry out synthesis of the steroids by using small molecules such as acetic acid [31]. Henceforth, it is proven that squalene was synthesized by using acetate, via the formation of mevalonate, the precursor of phytosterols, tetra and pentacyclic triterpens. The biosynthesis of steroids and triterpenoids thus is carried out via a common precursory molecule: squalene. This observation was reported by several authors [32–34].
According to these findings, we can follow the time course profile of squalene considered as a precursor of phytosterols. This can remove ambiguitie and allows to possibly confirm or infirm our assumption. Thus, during the decline phase of sterol (at the 10–12th week of maturity), a significant accumulation of squalene of cultivars Achaak and Mazetto was observed, while Perlees cultivar registered a less amount for this compound (Fig. 4). However, at the end of maturity, the squalene content was high for Achaak and markedly low for Perlees and Mazetto cultivars. Thus, let us conclude that there is no transformation of squalene into phytosterols, in particularly sterols D, E and F.

Squalene composition of three cultivars of almond during maturation. Time indicates weeks after the 40th day of flowering.
This lets us consider that enzymatic activities decrease and that the almond kernel is unable to synthesize of novo sterols at the latest stages of maturation. Thus, the preexistent phytosterols and possible terpenoids are simply converted into other sterols via dehydration, dehydrogenation, methylation or migration of double bonds. Indeed, β sitosterol undergoes dehydration to give stigmastadiene or it is transformed into stigmasterol after dehydrogenation step or it undergoes a reduction and leads to stigmastanol formation. In this case, β sitosterol decreases and favors an increased formation of these compounds.
The overall results confirm well our assumption and suggest the occurrence of an interconversion of other pre-existent phytosterol and terpenoid compounds into phytosterols D, E and F during the latest stages of maturation. These results were in agreement with others authors who mention that in sorghum for example, pentacyclic terpenoids may replace sterols in certain-controlled functions [35].
3.5 Effect of unsaturated fatty acids on the cholesterol level
Fig. 5 shows that during the maturation, there is probably a transformation of 24-methylene cholesterol to cholesterol. Knowingly the depressive effect of unsaturated fatty acids (UFA) on the accumulation of the cholesterol level [36] we wonder whether this effect is direct, or implies an intermediate compound such as 24-methylene cholesterol or another derivative. Such a reaction could block the transformation of the latest compound and consequently decreases the cholesterol level.

Evolution of cholesterol and 24-methylene cholesterol during maturation of cultivar Mazetto. Time indicates weeks after the 40th day of flowering.
On the one hand, the increase of oleic acid (C18:1) was accompanied with a decrease of 24-methylene cholesterol level during maturation. A rather low content of the 24-methylene cholesterol at the 8th week of maturation was observed (Fig. 5) as well as a high level of oleic and linoleic acids (C18:2) (Table 2).
Changes in unsaturated fatty acids composition throughout almond kernel development. Time indicates weeks after the 40th day of flowering.
Week/Fatty acid (%) | C18:1 | C18:2 | C18:3 |
1 | 4.98 | 40.34 | 24.72 |
2 | 6.00 | 38.9 | 24.22 |
3 | 6.99 | 35.1 | 17.98 |
4 | 8.71 | 40.36 | 22.63 |
5 | 10.55 | 41.22 | 26.11 |
6 | 12.19 | 46.17 | 22.8 |
7 | 20.9 | 50.9 | 11.74 |
8 | 31.7 | 47.48 | 3.74 |
9 | 44.36 | 39.18 | 1.28 |
10 | 43.43 | 40.68 | 0.92 |
11 | 50.05 | 37.14 | 0.46 |
12 | 52.37 | 35.92 | 0.26 |
13 | 62.04 | 26.97 | 0.17 |
14 | 64.04 | 25.18 | 0.14 |
15 | 68.87 | 21.13 | 0.11 |
16 | 71.27 | 19.16 | 0.1 |
17 | 70.45 | 19.42 | 0.09 |
18 | 72.36 | 17.66 | 0.12 |
19 | 74.79 | 15.62 | 0.08 |
20 | 72.99 | 16.85 | 0.13 |
21 | 72.43 | 17.56 | 0.17 |
22 | 73.98 | 16.44 | 0.19 |
23 | 76.15 | 14.23 | 0.15 |
On the other hand, a marked decrease of linolenic acid (C18:3) content is observed from the 8th week of maturation. The level of this acid passed from 12 to 4% and vanished for most times of maturation. At this time, the synthesis of 24-methylene cholesterol reaches its minimum level while cholesterol continues to increase (Fig. 5). This suggested that among the UFA, the C18:1 exerts an inhibition effect on the accumulation of cholesterol whereas the C18:3 exerts an inhibition effect on the transformation of 24-methylene cholesterol to cholesterol and consequently on total cholesterol level. In the last case the absence of this inhibitor or its presence with a very weak concentration from the 8th week (Table 2) removes the inhibiting effect of C18:3 and restores partially the accumulation of cholesterol starting from 24-methylene cholesterol.
According to Table 2 the C18:3 level is decreased and vanishes at the latest stages of maturation. In the same way, the C18:2 content is decreased considerably and reached a level less than 15% at the end of maturation. However, the oleic acid level is increased to more than 75% at the same time. This indicates that almond kernel oil is essentially a highly unsaturated oil type oleic [37]. Thus during this time, the oil composition is changed. This change is certainly accompanied by some modification in its quality, particularly by the absence of the C18:3 and change in sterol composition. So, the consumption of the almond fruits is recommended at the early stage of the development.
4 Conclusion
In summary, our studies showed that during the maturity of almond cultivar, there is the occurrence and biosynthesis of 12 sterols which can be divided into two classes: whereas the one is presented the major sterols (D, E, F, G, H and I), the other is presented the minor sterols (A, B, C, J, K and L). The most predominant compounds of all cultivars were in following order: sterol G, H, D and E. The quantitative analysis and characterisation showed that there are oscillations of accumulation of total sterols during maturation of almond cultivars accompanied with a dramatic decrease at the 10–12th week. This decrease was observed in the same time with the high accumulation of squalene. Moreover, there was a decrease of total sterols at the end of maturation of cultivar Achaak. This decrease was followed by an increase in squalene which accumulates at high content at the last stage of maturation of cultivar Achaak (more than 120 mg/100 g TL). That suggests there is the absence of the synthesis of novo sterols from squalene which was maintained an enzymatic activity until the end of maturity. However, the increased of compounds D, E and F at last stage of maturation of Perlees and Mazetto cultivar can be explained by conversion of others phytosterols particularly β sitosterol into these compounds. An inversely proportional relation between cholesterol, 24-methylene cholesterol was highlighted as well as a relation between these compounds and linolenic acid. The last one may inhibit the accumulation of 24-methylene cholesterol, which in its turn, could not be transformed in cholesterol in the presence of a high level of oleic acid. Linolenic acid is present at high levels only at the beginning of maturation. So, the beneficial and physiological effects on health of this acid are occurring in the early stage of harvesting period of almond.
Acknowledgements
We thank the following institutions for financial support and technical assistance of this work: L'Agence Canadienne de Développement International (ACDI), the Ministry of Scientific Research, Technology and Competence Development of Tunisia, Centre de Biotechnologie, Technopole de Borj-Cedria, Tunisia.