1 Introduction
Traceability and authenticity of oils from vegetable seeds and fruits are of great importance, due to the expanding demand for oils, as well as for their nutritional and medical applications [1–3]. Olive oil is one of the food products that are limited by regulations established by the European Union and the International Olive Oil Council, to control fraud [4]. Determination of adulteration and characterization of oils are based on the analysis of minor [5–7] and major [8,9] compounds. The major components of oil, called also the glyceredic fraction, are triacylglycerols. These are present as 95–98% of total lipid compounds [10]. Moreover, monoacylglycerols, diacylglycerols and free fatty acids can be also present [11]. In addition to these molecules, other minor compounds, called unsaponifiables, can be mentioned [12]. The unsaponifiables are the delicate and the interesting fraction of the lipid matter [13]. They really represent the credible maker to characterize [8] and to detect the admixture of vegetable oils [14]. The unsaponifiable fraction of vegetable oils consists of various classes of molecules, such as sterols (4-desmethylsterols), triterpene alcohols, 4-monomethylsterols, aliphatic alcohols, vitamins and hydrocarbons compounds. Moreover, olive oil has a special flavour that distinguishes it from other edible vegetable oils. The flavour fraction of olive oil is generated from a number of volatile molecules that are present at extremely low concentrations [15,16], in which sesquiterpenes and aldehydes compounds were the major chemical classes [17].
In the last decades, a suite of analytical methods (chromatographic and spectrometric techniques) have been developed to identity and quantify the individual component of these lipid factions. Thin layer chromatography (TLC) [18], high-temperature gas-liquid (GLC) [19] and atmospheric pressure chemical ionisation mass spectrometry (APCI–MS) [20] were used to characterize triacylglycerol composition of vegetable oils. In addition, GC-MS is considered to be the suitable method to analyse the different unsaponifiable subfractions after further isolation of each class using TLC [12]. Nevertheless, little research on the analysis of 4-desmethylsterols (unsaponifiable subfraction) using LC-MS is available [21]. Furthermore, with the advent of high-resolution capillary GC using fused-silica columns, GC-MS was the suitable technique to identify the flavour components of vegetable oils [22]. The isolation of aroma compounds from the lipid matter was generally carried out using SPME or Hydrodistillation as extraction methods [23].
The aim of the present work is to carry out a simultaneous analysis of glyceridic, unsaponifiable and flavour fractions of Tunisia Sayali olive cultivar using different chromatographic methods. The knowledge of the lipid fraction compositions of olive is of a great importance in the detection of olive oil adulteration as well as in the evaluation of the nutritional value of these phytomolecules. It is known that because of their similarities in fatty acid and triacylglycerol compositions, hazelnut oil is used to adulterate olive oil.
2 Experimental
2.1 Oil sample extractions
Virgin olive oil samples were obtained from the Tunisia Sayali Olea europaea L. cultivar. The olive trees (10 plants) were grown on the Agronomy farm of the Office Terres Domaniales Ghzala (OTDG, Bizerte) in the north of Tunisia. Only healthy fruits, without any sign of infection or physical damage, were selected. In this study, two kinds of method were used to extract the oil: the first one was achieved with a hammer crusher. Briefly, olives from the Sayali cultivar were washed and crashed, and the paste was mixed at 25 °C for 30 min. After centrifugation, the oil was transferred into dark glass bottles. This method extracts oil without the intervention of solvent and temperature, but it gives a lower quantity of oil. This method preserves both the typical aroma of olive oil and the chemical structure of some fragile molecules, such as triacylglycerols.
The second method was performed using a Soxhlet apparatus, with petroleum ether as the extraction solvent. The extraction remained 4 hours at 40 °C and was repeated three times for each sample. The extract was dried in a rotary evaporator at 32 °C. Oil was weighed and stored at −10 °C. The oil obtained was used to carry out the unsaponifiable analyses. The advantage of this method is to produce a higher quantity of oil.
2.2 Gas chromatography–flame ionization detection (GC–FID) analysis of fatty acids
For the determination of fatty acids (FA) composition, the methyl-esters were prepared by vigorous handshaking of a solution of oil in hexane (0.2 g in 3 ml) with 0.4 ml of methanolic potassium hydroxide 2N, and then 1 μl of the solution was injected into GC with a FID detector. A fused silica column (50 m length × 0.25 mm I.D.), coated with SGL-1000 phase (0.25 μm thickness; Sugerlabor, Spain), was used. The carrier gas was helium, at a flow through the column of 1 ml/min. The temperatures of the injector and detector were set at 250 °C and the oven temperature at 210 °C. The identification of FA components was performed by comparison of their retention times with authentic standards. The fatty acid content (expressed in % of total fatty acids) was calculated using the following formula; content (%) = PAi/TPA, where PAi = FA's peak area, TPA = total FA peak areas. Three independent replicates have been done for each sample.
2.3 High performance liquid chromatography (HPLC) analysis of triacylglycerols
The analysis of TAG was achieved using RP-HPLC. An amount of 0.5 g of oil was dissolved in 10 ml of acetone; 10 μl of the mixture was hand-injected into the RP-HPLC (HP 1100 Agilent Technologies, Santa Clara, USA) on a silica gel column (particle size 5 μm, 250 mm × 4.6 mm I.D.). Sensitivity of the detector was adjusted to RI = 0.9 units. TAG separation was achieved with an isocratic elution of acetone/acetonitrile (50:50; v/v) at the flow rate of 1.5 ml/min at 24 °C. Identification of TAG peaks was made by comparison of their retention times with authentic standards. The concept of the equivalent carbon number (ECN) has been used to rationalize the retention time of triacylglycerols. The ECN is defined as the total number of carbon atoms (CN) in the fatty acid acyl chains minus twice the number of double bonds (N) per molecule: ECN = CN–2N. The TAG content (expressed in % of total TAGs) was calculated using the following formula; content (%) = PAi/TPA, where PAi = TAG's peak area, TPA = total TAGs peak areas. Three independent replicates have been made for each sample.
2.4 Saponification
Unsaponifable fraction of lipids were determined by saponifying 5 g of oil with 50 mL of ethanolic KOH 12% (w/v) solution; the mixture was heated at 60 °C for 1.30 h. After cooling, 50 ml of H2O was added. The unsaponifiable matter was extracted four times with 50 ml of petroleum ether. The combined ether extract was washed 50 ml of ethanol-water (1:1). The extracted ether was dried over anhydrous Na2SO4 and evaporated to dryness using N2. The dry specimens were dissolved in chloroform for TLC analysis.
2.5 Thin layer chromatography separation
The unsaponifiable matter was separated into subfractions on preparative silica gel thin-layer plates (silica gel 60 G F254) using 1-dimensional TLC with hexane-diethyl ether (6:4, v/v) as the developing solvent. The unsaponifiable fraction diluted in chloroform was applied on the silica gel plates. So as to correctly identify the different unsaponifiable subfractions, reference samples of purified molecules (5-α-cholestanol, lanosterol and 1-eicosanol standards of sterols, triterpene alcohols and aliphatic alcohols, respectively) were applied on the left and the right sides of the TLC plates. After development, the plate was sprayed with 2,7-dichlorofluorescein and viewed under UV light. The band corresponding to each unsaponifiable subfraction was scraped, extracted three times with chloroform-diethyl ether (1:1, v/v), filtered to remove the residual silica, dried in a rotary evaporator and stored at −10 °C for GC-MS/GC-FID analyses.
2.6 Gas chromatography–mass spectrometry detection of the unsaponifiables
GC–MS analyses of the unsaponifiable subfractions were performed using a DB-5MS fused silica capillary column (30 m × 0.25 mm I.D., 0.25 m film thickness; J&W Scientific, Folsom, CA, USA) in an Varian SAR 3400Cx gas chromatograph coupled directly to the mass detector (MS Varian SATURN). Helium was used as carrier gas, with a constant flow rate of 1 ml min−1. The injector and detector temperatures were 250 °C. The oven temperature was programmed from 150 to 300 °C at 4 °C min−1. The final temperature was held constant for 10 min and the transfer line temperature was 250 °C. Electron impact mass spectra were measured at acceleration energy of 70 eV. Manual injection of 1 μl of the solution of sterol was performed in the split mode at a 60:1 split ratio. The sterols, triterpene alcohols, 4-monomethylsterols and aliphatic alcohols compounds were identified by comparing their retention times and mass spectra with those of their pure molecules. The peaks were also confirmed with NIST Mass Spectral Library.
2.7 Gas chromatography–flame ionization detection (GC–FID) quantification
Due to the higher sensibility of the GC–FID detector compared to the GC-MS one [12,13], the quantification of the unsaponifiable components was performed using GC-FID apparatus. The GC system used was a HP 4890A gas chromatograph (Hewlett-Packard, Avondale, PA) equipped with a split-splitless injector, a FID and a DB-5MS (30 m × 0.25 mm I.D., 0.25 m film thickness; J&W Scientific, Folsom, CA, USA) column was used. The initial column temperature was 150 °C and programmed to increase at a rate of 4 °C min−1 to 300 °C and then held for 25 min. The injector and detector temperatures were 280 °C and 310 °C, respectively, and helium was used as carrier gas at an inlet pressure of 12 psi, giving a column flow of 1 ml/min. The individual unsaponifiable component was calculated as milligrams per 100 g of oil using the following formula; amount = 100 − (PAs)(mis)/(PAis)(m), where PAs = compound peak area, PAis = internal standard area, mis = weight (mg) of the internal standard and m = weight (g) of oil taken for analysis.
2.8 Solid phase microextraction (SPME) of flavour compounds
Solid phase microextraction (SPME) was used as a technique for headspace sampling of samples. Supelo SPME devices coated with polydimethylsiloxane (PDMS, 100 μm) were used to sample the headspace of 3 ml of virgin olive oil inserted into a 5 ml glass vial and allowed to equilibrate for 30 min. After the equilibration time, the fiber was exposed to the headspace for 50 min at room temperature. Once sampling was finished, the fiber was withdrawn into the needle and transferred to the injection port of the GC and GC-MS system.
2.9 Gas chromatography–mass spectrometry detection of flavour compounds
GC analyses were accomplished with an HP-5890 series II instrument equipped with HP-Wax and HP-5 capillary columns (30 m × 0.25 mm, 0.25 μm film thikness), working with the following temperature program: 60 °C for 10 min, ramp of 5 °C/min to 220 °C; injector and detector temperatures, 250 °C; carrier gas, nitrogen (2 ml/min); detector, dual FID; split ratio, 1:30; injection 0.5 μl. The identification of the components was performed, for both columns, by comparison of their retention times with those of pure authentic samples and by means of their linear retention indices (LRI) relative to the series of n-hydrocarbons.
The relative proportions of the constituents were obtained by FID peak area normalization. GC-EIMS analyses were performed with a DB-5 capillary column (30 m × 0.25 mm; coating thickness = 0.25 μm) and a Varian Saturn 2000 ion trap mass detector. Analytical conditions: injector and transfer line temperatures, 220 and 240 °C, respectively; oven temperature programmed from 60 to 240 °C at 3 °C/min; carrier gas, helium at 1 ml/min; injection, 0.2 μl (10% hexane solution); split ratio, 1:30. Identification of the constituents was determined by comparing their retention times and mass spectra with those of their pure molecules.
3 Results and discussion
3.1 Analyze of fatty acids
Esterified fatty acids prepared from the lipid fraction of Sayali olive oil were injected into the gas chromatograph. The elution of the compounds from the capillary column is dependent on both the molecular weight and the degree of unsaturation of their carbon chain. Identification of fatty acid components was realized by the comparison of their retention times with authentic standards. The analyze of the chromatogram from our samples showed the presence of eight fatty acid peaks which appeared between 10 and 25 min retention time that explained the various fatty acid molecules in olive oils.
The quantitative characterization of the acidic composition (expressed in % of total fatty acids) showed that oleic acid was the predominant component which represents 77.4% of the total fatty acids. Other compounds were present in a relatively high percentage as palmitic acid (C16:0) (11.0%) and linoleic (C18:2) (5.9%). Within these compounds, several minor fatty acids were also detected such as stearic (C18:0) (2.7%), linolenic (C18:3) (1.7%), gadoleic (C20:1) (0.6%), palmitoleic (C16:1) and arachidic (C20:0) (0.2%) acids. Moreover, our results showed that Sayali oil cultivar was rich with unsaturated fatty acids (90.1%), especially monounsaturated ones (78.3%). Consequently, the high percentage of monounsaturated fatty acids in Sayali cultivar explains the nutritional benefits of olive since numerous researches indicating the beneficial health effects of these components [24,25]. The qualitative characterization of Sayali fatty acids composition was in agreement with those listed in literature [19,26–28]. Concerning the quantitative characterization of the fatty acid compounds, results showed that it differed from one olive oil to another. Indeed, Salvador et al. [26] reported that olive oil from Cornicabra cultivar (Spain) contained about 80% of oleic acidic, 10% of palmitic acid and 4% of linoleic acid. However, Buccioni et al. [27] mentioned that the percentages of these fatty acids in Italian olive oil sample were, respectively, 38.74, 21.35 and 19.6%. Moreover, the content of these major fatty acids from Tunisian cv. Sayali was 77.4, 11 and 5.9% for oleic, palmitic and linoleic acids, respectively. Thus, we explained the difference in the quantitative characterization by the fact that the content of fatty acids is affected by geographical g rowing area and difference in olive varieties [29–32].
3.2 Analyze of triacylglycerols
HPLC technique with a RP-18 column separates TAG according to both length and unsaturation degree of their carbon chain [33]. The oil sample was dissolved in acetone and directly injected into an HPLC column without any preliminary chemical derivatization or purification. Identification of TAG peaks was made by comparison of their retention times with authentic standards. The TAG peaks in the chromatogram appear between 12 and 38 min retention time and this is due to the various TAG species in Sayali olive oil (Fig. 1). The main peak in the HPLC chromatogram corresponds to the triolein (OOO).
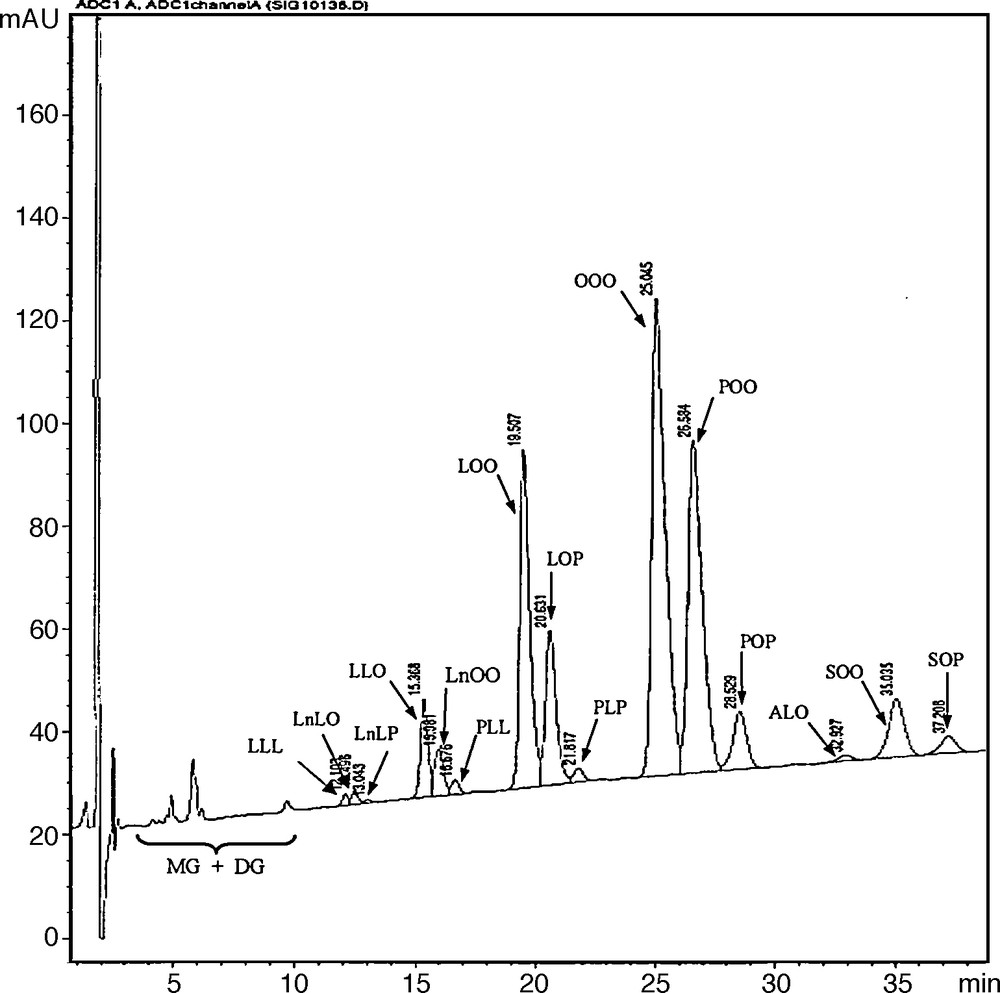
Triacylglycerols profile of Sayali olive oils analyzed by HPLC. (L, linoleic; Ln, Linolenic; O, oleic; P, palmitic; A, arachidic; S, stearic acids; MG, monoacylglycerol; DG, diacylglycerol).
Results from the quantitative characterization of the sample (expressed in % of total triacylglycerols) showed the presence of fifteen TAG which differed in their contents. OOO was expressed at higher level which represented 47.4% of total identified TAG. Moreover, POO (22.1%) and LOO (11.9%) were present in a relatively high percentage. Besides these compounds, several minor TAG molecular species were identified in our sample as SOO (3.7%), POP (2.7%), LLO ≈ LnOO (1.9%), ALO (0.9%) and LnLP (0.2%). In Sayali olive oil, the percentage of the main TAG species were present in this order OOO > POO > LOO > LOP. After this quantitative characterization of TAG molecular species, we deduce that the fatty acids content of Sayali oil predict the TAG composition of the studied olive oil. In fact, the percentage of the main fatty acids determined in Sayali oil were in order of Oleic (77.5%) > Palmtic (10.8%) > Linoleic acids (5.8%). The different TAG species and their percentages from the studied cultivar were in agreement with those listed in literature. Indeed, results from the quantitative characterization of TAG from olive sample studied by Jakab et al. [10] showed that OOO (46.43%) was the highest component followed by POO (18.14%) and LOO (12.34%). Moreover, Ollivier et al. [34] reported that OOO (45.36%), POO (21.69%) and LOO (12.05%) were the main TAG species detected in French olive oils collected from the area of Haute-Provence.
3.3 Analyze of sterols (4-desmethylsterols) and stanols
Sterols and stanols extracted from the unsaponifiable matter of Sayali oil were silylated and then directly injected into GC-MS chromatograph. The sterols identified and stanols are given in Table 1. The results from the qualitative characterization of the sample showed the presence of eleven sterols and two phytostanols (sitostanol and campestanol). The quantification of identified compounds was achieved using GC-FID.
Sterols content (expressed in mg/100 g oil) of olive (Cvs Sayali and Picual) and hazelnut oil samples.
Compounds | Cv. Sayalia | Cv. Picualb | Hazelnut oilc |
Cholesterol | 1.8 ± 1.1 | 0.3 | 0.6 |
24-Methylene cholesterol | 0.5 ± 0.2 | 0.2 | –d |
Campesterol | 3.7 ± 1.7 | 3.4 | 3.9 |
Campestanol | 0.2 ± 0.4 | 0.1 | 0.2 |
Stigmasterol | 1.4 ± 0.9 | 0.5 | 0.8 |
Δ7-Campesterol | 0.3 ± 0.1 | 0.1 | – |
Clerosterol | 0.9 ± 0.6 | 0.9 | – |
β-Sitosterol | 147.5 ± 12.4 | 86.4 | 63.5 |
Sitostanol | 0.7 ± 0.5 | 0.8 | – |
Δ5-Avenasterol | 18.3 ± 3.7 | 6.1 | 3.9 |
5.24-Stigmastadienol | 0.3 ± 0.1 | 0.5 | 0.3 |
Δ7-Stigmastenol | 0.2 ± 0.1 | 0.1 | 0.2 |
Δ7-Avenasterol | 0.3 ± 0.1 | 0.3 | 0.5 |
Results from the quantitative analysis of sterols from Sayali oil (expressed in mg/100 g of oil) showed that β-sitosterol was the predominant component (147.5 mg/100 g oil) accounting of over 82% of total sterols. Beside this compound, Δ5-avenasterol was present in a relatively high concentration (18.3 mg/100 g oil). However, campesterol (3.7 mg/100 g oil), cholesterol (1.8 mg/100 g oil), stigmasterol (1.4 mg/100 g oil), 5,24-Stigmastadienol (0.3 mg/100 g oil) amounted to less than 5% of total sterols content. The Δ7-sterols were the minor sterols detected in Sayali olive oil and they represented by Δ7-avenasterol (0.2 mg/100 g oil) and Δ7-campesterol (0.3 mg/100 g oil). In addition to sterols, the studied oil contains phytostanols which represent the saturated forms of sterols (campesterol and β-sitosterol [35]. In our sample, stanols were found to be minor components of the sterolic fraction. Sitostanol (0.7 mg/100 g oil) and campestanol (0.2 mg/100 g oil) were the unique stanols detected in Sayali olive oil. The qualitative characterization of our samples was in agreement with the results reported by Casas et al. [4] (Table 1). However, the quantitative characterization was different which can be explained by the fact that the level of 4-desmethylsterols is affected by geographical growing area and difference in olives varieties [4,36,37]. A direct comparison of sterols (4-desmethylsterols) and stanols of Sayali olive oil with the hazelnut ones [14] (Table 1) showed that the composition of these compounds were rather similar in both olive and hazelnut oils.
3.4 Analyze of triterpene alcohols and monomethylsterols
After their separation by preparative TLC, methylsterols (triterpene alcohols (4,4′-dimetylsterols) and monomethylsterols) subfractions were analyzed by GC-MS and GC. The identified triterpene alcohols and monomethylsterols were given in Table 2. The quantitative characterization of triterpene alcohols (expressed in mg/100 g of oil) showed that 24-methylene cycloartenol was the dominant triterpene alcohols in Sayali olive oil (146.4 mg/100 g oil) accounting of over 91% of total triterpene alcohols. Moreover, cycloartenol (52.3 mg/100 g oil) was found at minor amount compared to 24-methylene cycloartenol, thus cycloartenol is formed as the first cyclic triterpenoid precursor of phytosterols and works as a substrate for the first methylation reaction, resulting in 24-methylene cycloartenol [38]. However, β-amyrin (10.1 mg/100 g oil), δ-amyrin (2.8 mg/100 g oil) and traroxerol (2.6 mg/100 g oil) were less present in Sayali olive oil. The characterizations of our samples mentioned above were in agreement with those of Azadmard-Damirchi et al. [12] (Table 2).
Triterpene alcohols and 4-monomethylsterols content (expressed in mg/100 g oil) of olive and hazelnut oil samples.
Compound | Cv. Sayalia | Olive oilb | Hazelnut oilb |
Triterpene alcohols | |||
δ-Amyrin | 2.8 ± 1.2 | 12.3 | 1.5 |
Taraxerol | 2.6 ± 1.1 | 7.0 | nd |
β-Amyrin | 10.1 ± 2.2 | 30.7 | 1.2 |
Cycloartenol | 52.3 ± 4.5 | 75.0 | 2.0 |
24-Methylene cycloartenol | 146.4 ± 12.6 | 173.4 | 6.2 |
Monomethyl | |||
Obtusifoliol | 7.7 ± 1.9 | 9.4 | 5.2 |
Gramisterol | 4.2 ± 1.4 | 4.2 | 1.9 |
Cycloeucalenol | 16.2 ± 3.8 | 12.7 | tr |
Citostradienol | 42.1 ± 4.1 | 40.2 | 29.7 |
a Each value is a mean of three determinations.
b Results reported by Azadmard-Damirchi et al. [12].
In Sayali olive oil, the 4-monomethylsterol components were minor compared to triterpene alcohols (4,4′-dimethylsterols) and sterols (4-desmetylsterols). In the present study, the total 4-monomethylsterol components were found at about 8% of total phytosterols. Results from the quantitative characterization showed that cycloeucalenol (42.1 mg/100 g oil) and citrostadienol (16.2 mg/100 g oil) were the most abundant 4-monomethylsterol compounds followed by obtusifoliol (7.7 mg/100 g oil) and gramisterol (4.2 mg/100 g oil) (Table 2). The qualitative and quantitative characterizations of 4-monomethylsterols components differed from one vegetable oil to another. Harrabi et al. [39] suggested that corn oils contained 34% of obtusifoliol, 28% of gramisterol and 44% of citrostadienol. However, Azadmard et al. [14] demonstrated also that cycloeucalenol was present at only a trace level in hazelnut oils. On the other hand, our results show that citrostadienol (59%) and cycloeucalenol (23%) were the major 4-monomethylsterols compounds in olive oils. Therefore, we propose to use 4-monomethylsterols fraction to detect the adulteration of vegetable oils.
3.5 Analyze of aliphatic alcohols
The results from the qualitative characterization of Sayali olive using GC-MS indicated that the aliphatic alcohol fraction of oil was made up of very long chain aliphatic primary alcohols (20–36 carbon). The identified compounds were docosanol, tricosanol, tetracosanol, pentacosanol, hexacosanol, heptacosanol, octacosanol and triacontanol (Table 3). The quantitative characterization of aliphatic alcohols of Sayali olive oil (expressed in mg/100 g of oil) showed that hexacosanol (49.3 mg/100 g oil), tetracosanol (36.5 mg/100 g oil), octacosanol (28.9 mg/100 g oil) and docosanol (20.1 mg/100 g oil) were the dominant policosanol compounds accounting for over 85% of total policosanol. However, pentacosanol (9 mg/100 g/oil), heptacosanol (7.2 mg/oil) and tricosanol (3.5 mg/100 g/oil) were less present in Sayali olive oil. In fact, the results from the quantitative characterisation of samples examined here showed that the aliphatic alcohols with pair carbon chain were more accumulated than the impair ones. Moreover, the qualitative characterization of Sayali oil showed that all the identified aliphatic alcohols fraction were policosanol (aliphatic alcohols with 20–36 carbon atoms). Policosanol is a mixture of bioactive molecules shown to have beneficial effect in treating hypercholesterolemia. Recently, food products enriched with policosanol are currently available in the US market [40]. Thus, the wealth in long chain aliphatic alcohols level makes olive of great beneficial effects since recent published studies focused on its healthy effects [41,42]. Concerning the aliphatic alcohols composition of hazelnut oil, a direct comparison with those of Sayali olive oil is not realizable since, to our knowledge, no information is not available concerning of the aliphatic alcohol composition of hazelnut (Table 3).
Retention time (RT) and mass spectrometric data for aliphatic alcohols components identified by GC-MS.
R.T (min) | Mains fragmentation ions (M/Z) | Compound | Contenta (mg/100 g oil) |
13.41 | 280, 195, 111, 69, 55, 43 | Docosanol | 20.1 ± 2.8 |
16.75 | 308, 223, 125, 111, 57, 43 | Tricosanol | 3.5 ± 1.3 |
19.20 | 336, 252, 153, 111, 97, 57 | Tetracosanol | 36.5 ± 3.2 |
22.04 | 350, 265, 139, 97, 83, 43 | Pentacosanol | 9.0 ± 2.1 |
25.17 | 364, 236, 125, 111, 97, 57 | Hexacosanol | 49.3 ± 4.2 |
28.21 | 378, 208, 111, 97, 71, 29 | Heptacosanol | 7.2 ± 1.8 |
31.19 | 364, 265, 125, 97, 69, 43 | Octacosanol | 28.9 ± 2.9 |
36.70 | 392, 336, 223, 139,111, 57 | Triacontanol | 1.7 ± 0.9 |
a Each value is a mean of three determinations.
3.6 Analyze of flavour fraction
The knowledge of the flavour composition of olive oil is very important since it is related to its authenticity and is used to detect olive oil adulteration [43]. In the present study, flavour components were extracted using SPME and then were identified by CG-MS. In Sayali olive oil, eighteen volatile compounds were detected (Fig. 2). Results from the quantitative characterization of Sayali volatiles showed that (E)-2-undecenal (23.4%), (E)-2-decenal (19.1%) and nonanal (11.2%) were the main flavours. Beside these dominant components, camphor (0.6%), decanal (0.9%), dodecanal (0.3%) and pentacosane (1.1%) were also present. However, camphene, 1,8-cineole and (E,E)-α-farnesene were found as trace (< 0.1%) in Sayali olive oil. After this characterization using SPME, results showed that the aldehyde compounds were responsible for the flavour and aroma in Sayali olive oil. Moreover, results from the qualitative and quantitative characterizations of our sample were different from those listed in the literature [44,45]. This difference can be explained by the fact that the volatile composition is extremely influenced by several factors, such as, degree of olive maturity, effect of variety, agronomic conditions and the extracted method used [22,46]. Furthermore, it is interesting to mention that the flavour fraction of hazelnut oil has never previously been studied; that is why we cannot establish a comparison with those of olive oil.
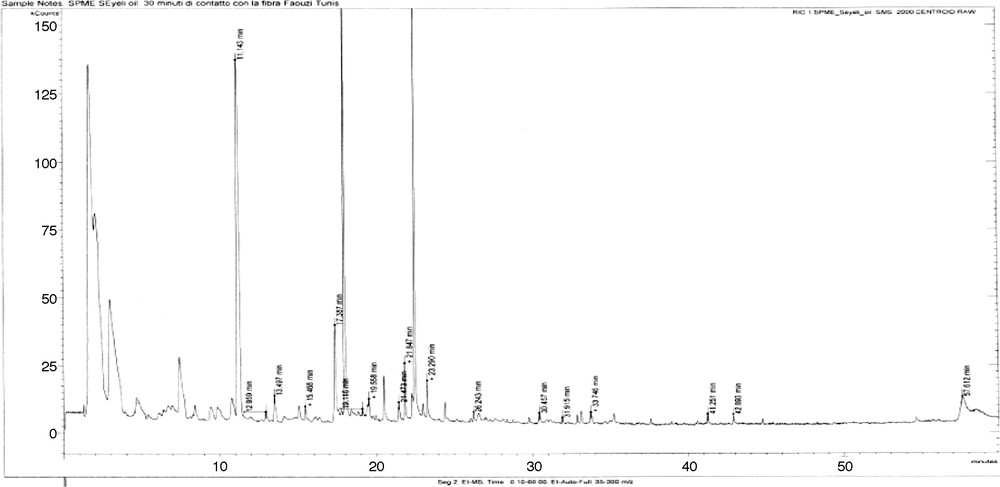
Typical mass chromatogram of the aroma components extracted from Sayali olive oil.
4 Conclusion
In summary, taken together the results from the analysis of the glyceridic, unsaponifiable and flavour fractions of Sayali olive oil: (1) the olive and hazelnut oils have nearly similar fatty acids and TAG profiles; (2) the triterpene alcohols (4,4′-dimethylsterols) and the monomethylsterols were present differently (qualitatively and/or quantitatively) into the two types of oil; (3) the aliphatic alcohol composition of hazelnut was not characterized previously; (4) the olive and hazelnut oils have different flavour tastes. Thus, it is interesting to mention that the unsaponifiable fraction determines both the origin and the nature of lipids. Therefore, we suggest the use of triterpene alcohols and the monomethylsterols as obligatory analyses by the International Olive oil Council during the world commercialization of the vegetable oils.
Acknowledgements
This work has been done as a part of a National Research Project. We thank the Ministry of Scientific Research, Technology and Competence Development of Tunisia for financially supporting this investigation. Part of this work was carried out in both at “Centre d’étude structurale et d’analyse des molécules organiques”. Bordeaux 1, France and at “Dipartimento di Chimica Bioorganica e Biofarmacia”, Pisa, Italy.