1 Introduction
The mulberry moth, Glyphodes pyloalis Walker (Lepidoptera: Pyralidae) is an important pest on mulberry and has been reported in Iran (Guilan province) since 2002 [1]. Females lay eggs individually on the lower surface of leaves between veins. The eggs hatch within 3–4 days and the hatched larvae feed on parenchyma of leaves and cause folding. Each generation takes 25 days and it can have five generations in a year so that damage from fourth and fifth generations are more important. α-Amylase (α-1,4-glucan-4-glucanohydrolases; EC 3.2.1.1) is a midgut and salivary enzyme that is involved in starch and other carbohydrate metabolism and its activity level is dependent on feeding diet [2]. The enzyme catalyzes the hydrolysis of α-D-(1,4)-glucan linkage in starch components, glycogen and other related carbohydrates to serve as an energy source [3,4]. The α-amylase converts starch to maltose, which is then hydrolyzed to glucose by α-glucosidase. In insects, only α-amylases have been found to hydrolyze long α-1,4-glucan chains, such as starch or glycogen [5,6]. The activity of α-amylase is dependent on food sources. In insects which feed on wool, α-amylases concentration is low whereas in phytophagous insects, α-amylase is abundant and its activity is high [7,8]. Amylase activity has been described in several insect orders, including Coleoptera, Hymenoptera, Diptera, and Hemiptera [9–13]. Few reports on other lepidopteran amylases are available viz. Bombyx mori, Diatraea saccharalis, Ostrinia nubilalis, Spodoptera frugiperda, Chilo suppressalis, Plodia interpunctella, Helicoverpa armigera [14–17].
G. pyloalis feeds on mulberry leaves that are rich source of polysaccharides; hence, it must rely on the effectiveness of its α-amylase for digestion of polysaccharides to survive [11]. An understanding of how digestive enzymes act on their substrate in insects, it is essential to develop methods of insect control. Determination of digestive enzyme reactions to different inhibitors is a winning procedure to develop transgenic plants programs to control phytophagous insects [18–20]. Hence, the purpose of the present study is to identify and characterize the α-amylase activity from midgut and salivary glands of mulberry pyralid larvae to gain a better understanding of their digestive physiology and get access to new management strategies for control of this pest.
2 Material and methods
2.1 Insect rearing
The larvae of G. pyloalis were collected from mulberry trees in Rasht (Guilan province, Iran) and reared on fresh shin ichinoce variety under laboratory condition (25 ± 2, RH 75 ± 5 and 16:8 LD). Adults were provided with 10% honey in cotton wool. Fresh mulberry leaves were provided for egg laying.
2.2 Sample preparation and enzyme assays
Two day old fifth stadium larvae were randomly selected and dissected in distilled water under a dissecting microscope. Midgut and salivary glands were removed and rinsed in distilled water, and then placed in a precooled homogenizer and were ground in 2 mL of universal buffer (0.02 M) (pH 6.5) [21]. Homogenates were then transferred to 1.5 mL centrifuge tubes and were centrifuged at 12,000 rpm for 20 min at 4 °C. The supernatants for each tissue were pooled and then stored at −20 °C for later analyses.
The activity of α-amylase was assayed using the dinitrosalicylic acid (DNS) procedure [22], 1% soluble starch (Merck, Darmstadt, Germany) was used as the substrate. Ten microliters of the enzyme were incubated for 30 min at 35 °C with 80 μL universal buffer and 20 μL soluble starch. In order to stop the reaction, 90 μL DNS was added and the mixture was heated in boiling water for 10 min. DNS is a color reagent and reacts with the reducing groups released from starch by the action of α-amylase. The boiling water stops the α-amylase activity and catalyzes the reaction between DNS and the reducing groups of starch. Absorbance was measured at 492 nm. One unit of α-amylase activity was expressed as the amount of enzyme needed for producing 1 mg maltose in 30 min at 35 °C. A blank with no substrates but with α-amylase extract and a control without α-amylase extract but with substrate were measured at the same time as the reaction mixtures. All assays were performed in duplicate and each assay was repeated at least three times.
A standard curve of α-amylase absorbance against the amount of released maltose was constructed to enable the calculation of the amount of maltose released during the α-amylase assay. Serial buffer (pH 6.5) were made to produce the following concentrations: 0.125, 0.25, 0.5, 1 and 2 mg/mL.
2.3 Kinetic parameters measurements
In each assay, 10 μl of appropriately diluted enzyme preparation was used and six concentrations 0.05, 0.1, 0.2, 0.4, 0.3, 0.6 and 0.8 percent starch was prepared in sodium phosphate buffer (0.02 mol/L) at pH 6.5. This experiment was performed a few time series and three replicates. The Michaelis constant (Km) and the maximum velocity (Vmax) were estimated by Sigmaplot software version 6 and the results of Km and Vmax were the means ± SE of three replicates (n = 3) for every tissue.
2.4 Effect of pH and temperature on enzyme activity
The effect of temperature on α-amylase activity was determined by incubating the reaction mixture at 20, 25, 30, 35, 37, 40, 45, 50, 55, 60 and 70 °C for 30 min, followed by measurement of activity. Optimal pH was determined using universal buffer with pH set at 3, 4, 5, 6, 7, 8, 9, 10, 11 and 12.
2.5 Effect of activators and inhibitors on enzyme activity
To find out the impact of different ions on enzyme activity, assays were performed in the presence of different concentrations of chloride salts of Na+ (5, 10, 20 and 40 mmol/L), K+ (5, 10, 20, and 40 mmol/L), Ca2+ (5, 10, 20 and 40 mmol/L), Mg2+ (5, 10, 20 and 40 mmol/L) and Ethylenediaminetetra acetic acid (EDTA: 0.5, 1, 2 and 4 mmol/L), sodium dodecylsulfate (SDS: 1, 2, and 4 mmol/L) and urea (0.5, 1, 2, 4, 6 and 8 mol/L). These compounds were added to the assay mixture, and activity was measured after 30 min. A control was also measured (no compounds added).
2.6 Electrophoresis
The activity of amylases present in crude homogenates of midgut and salivary glands after SDS-polyacrylamide gel electrophoresis (PAGE) was visualized using the procedure described by Lammli (1970) and Campos et al. (1989) [23,24], with minor modification. SDS-PAGE was performed in a 10% (W/V) separating gel and a 5% stacking gel, both with 0.05% SDS. The electrode buffer was prepared based on the method of Lammli (1970) [23], but SDS was not used. The sample buffer contained 25% stacking buffer (0.5 mol/L Tris-HCL [pH 6.8]), 20% glycerol, 2% SDS, 0.005% (W/V) bromophenol blue. Electrophoresis was conducted at room temperature at 120 V until the blue dye reached the bottom of the slab gel. To prepare gels for α-amylase assay, the gel was rinsed with distilled water and washed by shaking gently with 1% (V/V) Triton X-100 in phosphate buffer containing 2 mmol/L CaCl2 and 10 mmol/L NaCl for 1.5 h. The gel was then rinsed with distilled water and treated with a solution of 1.3% I2, 3% KI to stop the reaction and to stain the starch background. Zones of α-amylase activity appeared as light bands against a dark background.
2.7 Statistical analysis
Data were compared by one-way analysis of variance (ANOVA) followed by Tukey's studentized test when significant differences were found at p < 0.05 [25].
3 Results
3.1 α-Amylase activity
The α-amylase activity was present in both MG and SG of larval G. pyloalis (Fig. 1). The activity level in MG and SG were 0.011 and 0.002 μmol/min, respectively. There was a significant difference in activity between MG and SG.
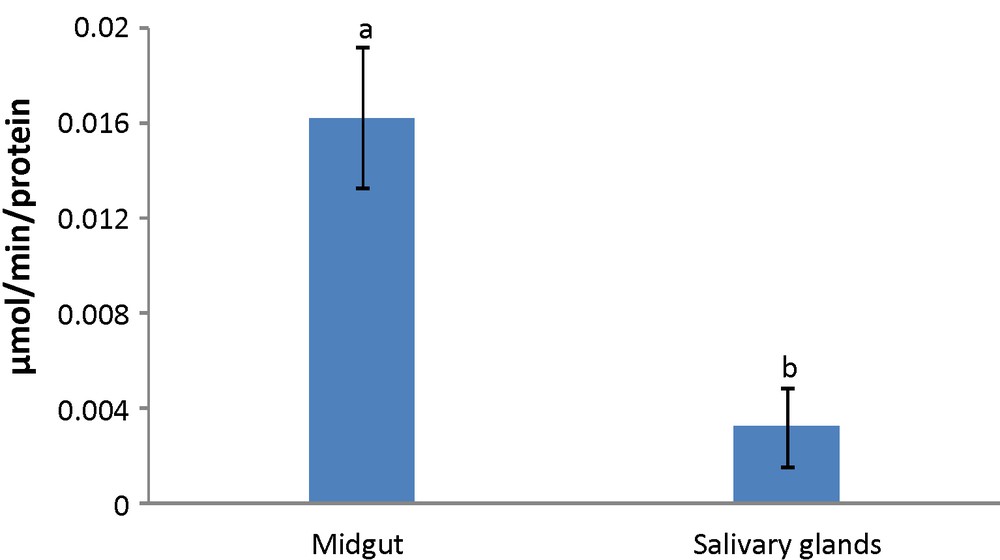
Activity level of α-amylase in fifth instar larvae midgut and salivary glands of mulberry moth. Different letters show significant differences Tukey (p < 0.05), n = 3.
3.2 Effect of pH and temperature on α-Amylase activity
The optimum pH for G. pyloalis was recorded as 9 and 10 in MG and SG, respectively (Fig. 2). The enzyme activity was increased gradually from 4 to 9 and 4 to 10 in case of MG and SG, respectively and decreased thereafter with increasing pH (Fig. 2). Amylase was found to be active over a broad range of temperatures; however, the optimal temperatures for its activity was found at 37 and 40 °C for MG and 37 °C in the case of SG (Fig. 3).

Lineweaver-Burk plots of α-amylase in the midgut and the salivary glands of mulberry moth larvae.
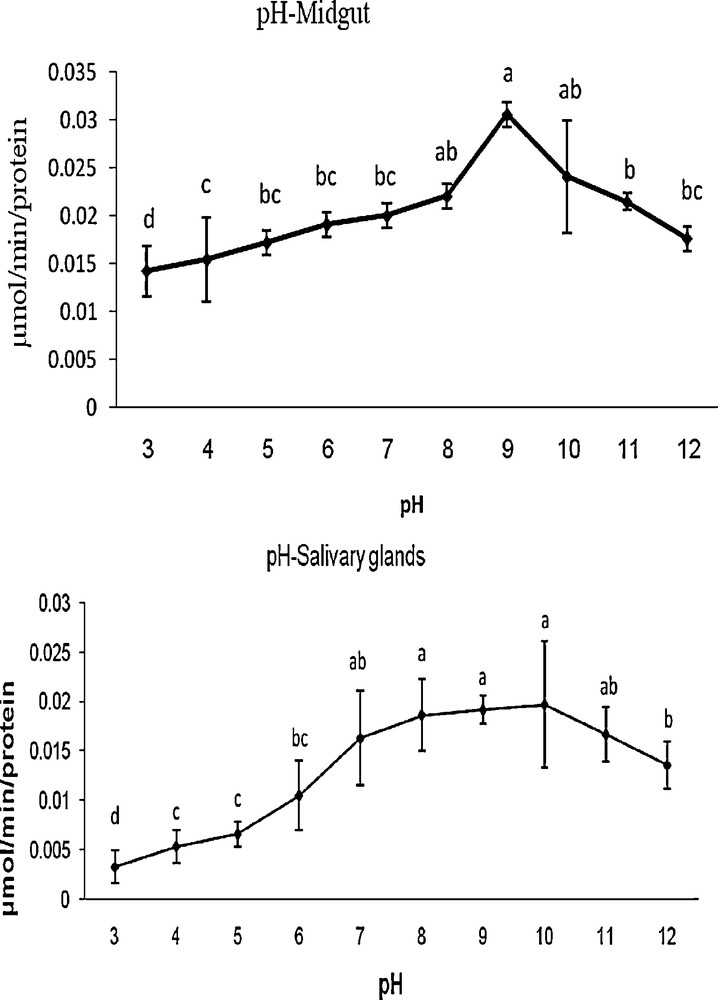
Effect of temperature on activity of α-amylase extracted from midgut and salivary glands in fifth larvae of mulberry moth. Different letters show significant differences Tukey (p < 0.05), n = 3.
3.3 Effect of activators and inhibitors on enzyme activity
Several chemicals were used in this study to find out their effects on activity level of α-amylase in MG and SG of G. pyloalis. The results are depicted in Table 1. Activity level of NaCl and CaCl2 in MG and SG was recorded maximum at 10 and 20 mmol/L concentrations. In case of KCl the highest activity was recorded at 20 mmol/L while for MgCl2 concentrations 10 and 40 were recorded for MG and SG respectively. EDTA showed the maximum activity at 1 and 2 mmol/L for MG and SG, respectively while for SDS was 8 and 6 mmol/L (Table 1). Maximum activity for Urea was recorded at 1 and 8 mmol/L for MG and SG, respectively (Table 1).
Relative activity (%) of mulberry moth α-amylase in the presence of different compounds.
Compounda | Concentration (mmol/L) | Relative activity in midgut (%) | Relative activity in salivary glands (%) |
Control | 100 | 100 | |
NaCl | 5 | 25* | 32/65 |
10 | 120/45* | 101/36 | |
20 | 63/63* | 183/67* | |
40 | 28/4* | 142/85* | |
CaCl2 | 5 | 56/81* | 149/65* |
10 | 60/27* | 46/25* | |
20 | 40/9* | 197/27* | |
40 | 46/59* | 102/04 | |
KCl | 5 | 81/13* | 152/38* |
10 | 84/09* | 125/17* | |
20 | 248/32* | 223/8* | |
40 | 78/04* | 107/48 | |
MgCl2 | 5 | 26/1* | 44/89* |
10 | 50* | 28/57* | |
20 | 36/36* | 38/09* | |
40 | 35/27* | 74/14* | |
EDTA | 0/5 | 23/86* | 95/91 |
1 | 55/68* | 87/07 | |
2 | 39/72* | 112/24 | |
4 | 37/5* | 30/61* | |
SDS | 2 | 25* | 85/71* |
4 | 27/27* | 49/65* | |
6 | 27/27* | 101/36 | |
8 | 37/5* | 97/27 | |
Urea | 1 | 53/4* | 105/8 |
2 | 43/18* | 127/21* | |
4 | 36/36* | 62/58* | |
6 | 45/45* | 72/1* | |
8 | 19/75* | 132/65* |
a The enzyme was preincubated for 10 min at 35 °C with listed compounds at the final concentration indicated before substrate addition.
* Significant versus control Tukey (p < 0.05), n = 3.
3.4 Electrophoresis
Analysis of MG and SG homogenates by electrophoresis indicated bands for MG and SG (Fig. 4).
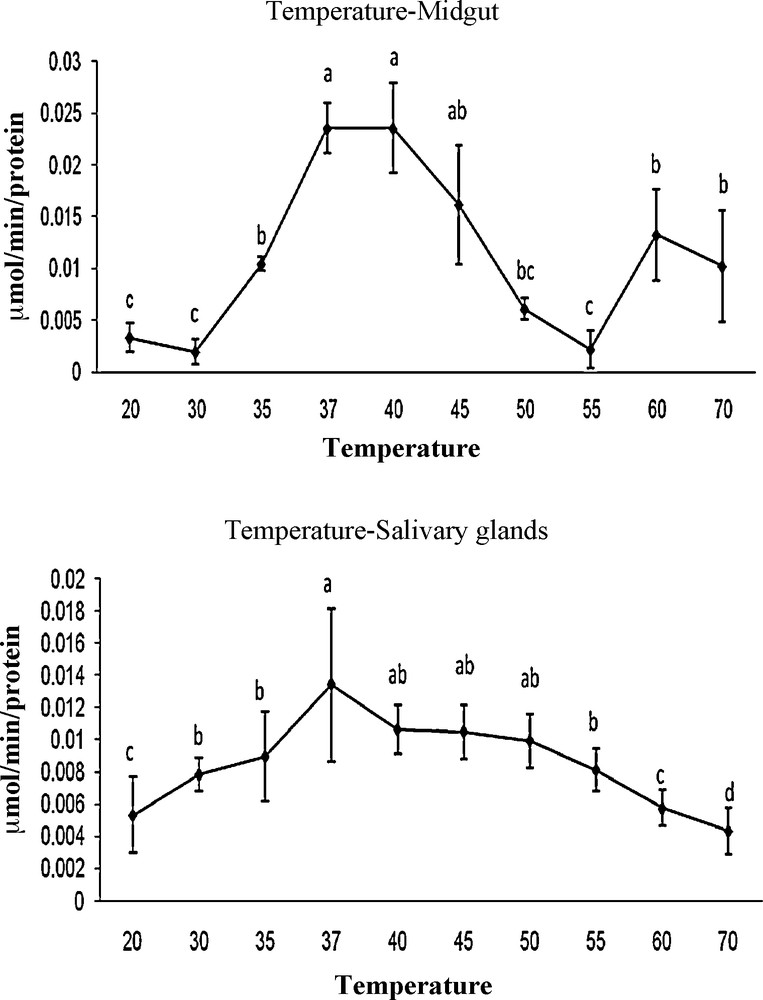
Non-denaturing sodium dodecylsulfate–polyacrylamide gel electrophoresis of midgut (right) and salivary glands (left) from mulberry moth.
3.5 Kinetic parameters (Vmax and Km) of α-Amylase in MG and SG
As can be observed Vmax for MG is 2.36 μmol/min/protein and 2.52 μmol/min/protein for SG. Km values were recorded 0.56% for MG and 0.37% for SG (Fig. 5).
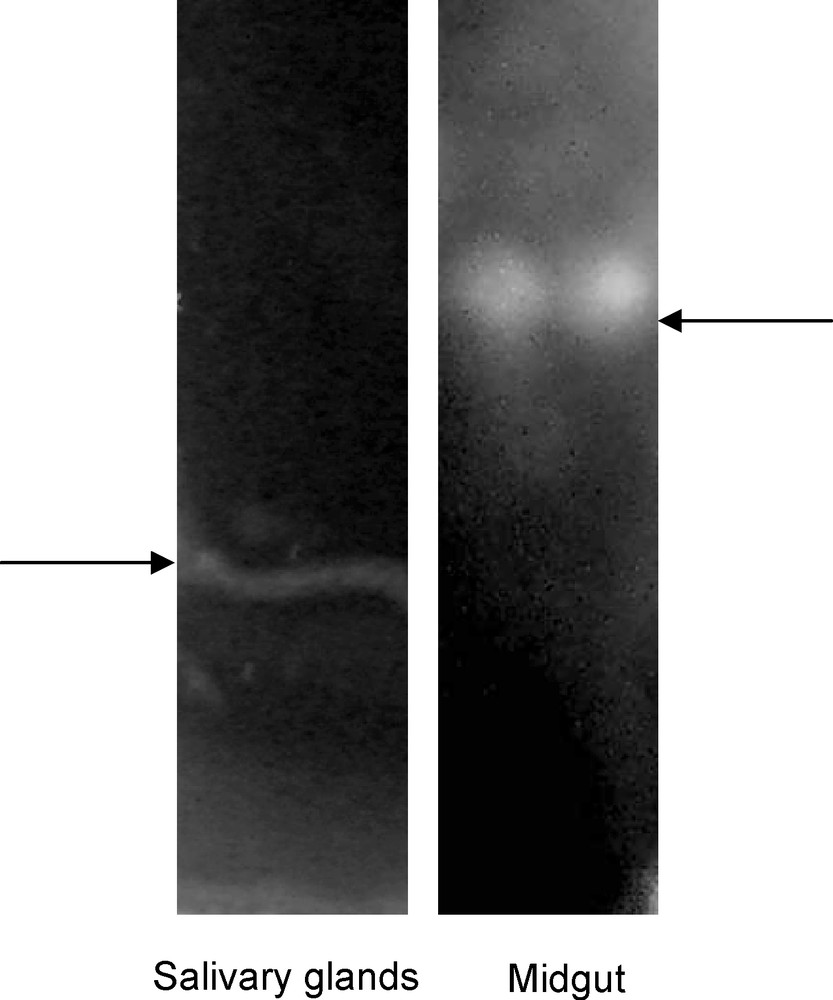
Effect of pH on activity of α-amylase extracted from midgut and salivary gland in fifth larvae of mulberry moth. Different letters show significant differences Tukey (p < 0.05), n = 3.
4 Discussion
This study indicated the α-amylase activity in MG and SG of G. pyloalis. Activity of α-amylase in MG of other insects ranges from 0.0673 to 1.2 μmol/min [26,27]. Our results are more or less similar to other lepidopterans; Antheraea mylitta Drury 0.673 μmol/min, Helicoverpa armigera Hubner, 0.034 μmol/min, Chilo supresalis, 0.66 μmol/min. G. pyloalis digest polysaccharids partially by salivary secretions, complete breakdown occur in midgut [15,28,29]. The activity of α-amylase was shown in a wide range of pH (3–10). The optimal pH was 9 in SG and 10 in MG which agrees with the reports in other lepidopterous insects. Abraham et al, (1992) [27] reported optimal pH of 9.2 for α-amylase activity in A. nylita. In Acherontia atropos pH of 12 was reported in MG lumen., that of Lasciocampa gurecus 10, 8, 11.3 for M. sexta and 10.8 for Lichnoptera felina. It is suggested that high gut pH is an adaptation of their leaf eating ancestors for extracting hemicellulose from plant cell walls [30]. In the present study, the optimal temperature value of α-amylase was 37 °C for MG and SG. This value is extremely lower than that reported for Blatella germanica (50 °C) [31], Bombyx mori (60 °C) [32] and H. armigera [17]. On the other hand, this value was higher than that reported for Tenebrio molitor (25 °C) [33]. But this optimal temperature is consistent with reports on other lepidopteran larvae [11,15,34]. Several inhibitors and activators were employed and the results were compared with other insects [5,6,12,15,35,36]. NaCl decreased α-amylase activity which is consistent with the reports on Chilo supressalis [15]. However, in lygus hesperusl and L. lineolaris [12,37] the activity was reduced for NaCl which is consistent with reports about other insects (e.g., Callosobruchus chinensis, B. mori, and Chilo supressalis) [5,6,15]. Similar inhibitors like K+ and Mg2+ ions on α-amylase activity in G. pyloalis has also been reported in C. supressalis [15]. EDTA and SDS showed inhibitory effect on α-amylase activity both in MG and SG which is consistent with other reports [12,16,38,39]. Ca2+ ions increase the activity of α-amylase in G. pyloalis since α-amylase are metalloproteinase and require calcium for maximal activity [16,40]. MG and SG homogenates showed one band each in SDS–PAGE electrophoresis which differs with other reports. A mixture of different bands showing different isoenzymes have been reported in Drosophila melanogaster [41]. Sitophilus oryzae [42], Tribolium castaneum [43], Rhyzopertha domenica [44], Anthonomus grandis [14] and [16]. Plant α-amylase inhibitors are usually expressed in response to herbivore insects, pathogens and wounding [45–49]. Insects have evolved amylases that are least affected by inhibitors naturally present in plant material. On the other hand, plants interact with herbivores with expressing more potential inhibitors.
While developing sustainable approaches for controlling lepidopteran insects, it becomes essential to characterize digestive enzymes and their inhibitors from different sources [18]. Because of the important role of amylases in insect development, any strategy that impairs the activity of this important enzyme is welcomed in insect control protocol [14]. Pusztail et al. 1999 [50], reported that transgenic plants encoded with inhibitors interfered with digestion and absorption of insect pests and interestingly no nutritional or toxic effects were observed in rat fed on transgenic pea expressing the α-amylase inhibitor. Morton et al. (2000) [51], also reported complete resistant to Bruchus pisorum and Callosobruchus chinensis of transgenic peas. Considering the non-safe use of chemical pesticides and residues in the environment transgenic plants encoded with resistant factors to herbivores may be more safe. Da Silva et al. (2004) [52], believe that the primary use of producing resistant transgenic plants is to reduce the cost and hazardous effect of chemical pesticides.