1 Introduction
Aluminum (Al) is one of the most abundant elements in the environment. Human exposure is largely oral with daily intake average of 30 to 50 mg, and most consumption is expected to be due to contaminated-water intake [1,2]. Al is incorporated into the human body including the central nervous system (CNS), where it is considered as a neurotoxin associated with neuronal dysfunction. For example, Al was associated with degenerative mechanisms leading to Alzheimer's disease [3]. In these patients, toxicological and physiological observations highlight an anatomical correlation between the areas of the cerebral cortex with strong content of Al [3].
Several bodies of evidence suggest that Al affects neuronal function by causing changes in neurotransmission and oxidative stress, such as, for example, a reduction in cholinergic activity [4], enhanced activity of glutamate decarboxylase [5], changes in GABA transport [6], altered membrane associated proteins (Na–K ATPase and PKC) and endogenous antioxidant enzyme activity [7]. Moreover, certain studies suggest that Al disrupts iron homeostasis in the brain neurons through the neuronal transferrin receptor [8]. However, these neuronal changes induced by Al intoxication could well be the consequence, or at least partially, of indirect effects on glial cells. Previous studies have shown the role of glial cells in Al neurotoxicity and suggested direct effects of Al on glial cell function including astrocytes [9,10]. In fact, some studies have reported altered astrocytic GFAP content in brains of animals following Al exposure [11,12]. Moreover, exposure of cultured astrocytes to AlCl3 increases the activity of the glutamine synthetase [13].
In the present study, we will evaluate the effects of chronic exposure to AlCl3 on the glial system using immunohistochemistry for GFAP in adulthood and since intrauterine age in rats. The extent of the glial changes will be assessed together with alterations of animal behavior by measuring locomotor activity and anxiety.
2 Materials and methods
2.1 Animals
Male and female wistar rats (200–300 g of weight) were used and divided into four groups. Group 1: 3 months old rats (n = 5) were subjected for 4 months to an orally chronic intoxication with drinking water containing 0.3% (3 g/l) AlCl3. Group 2: rats were chronically exposed to Al during gestational and lactation stages. During their gestational life, and through maternal milk the pups received Al from their mothers exposed to drinking water contaminated with 0.3% AlCl3. Following weaning, rats continue to receive drinking water contaminated with 0.3% AlCl3. At adult age (4 months old), 10 rat pups were used for the experiments. Groups 3 and 4: seven (n = 5) and four (n = 5) months old rats were used as controls for group 1 and group 2, respectively. Control rats were not subjected to any treatment.
2.2 Methods
Immunohistochemistry: We studied the cellular and subcellular distributions of the glial fibrillary acidic protein (GFAP). Animals were anesthetised with sodium pentobarbital (40 mg/kg i.p.), and perfused transcardially with chilled physiological saline and paraformaldehyde (4%) in phosphate buffer (PBS, 0.1 M, pH 7.4). Frontal sections 15 μm were cut with a microtome and collected in PBS. The slides were selected and preincubated during 2 h in PBS with 0.3% triton and 0,1% bovine serum albumin (BSA). Under agitation, the slides are then incubated overnight at 4 °C in a solution of anti-GFAP antibody (1/5000) containing PBS (0.1 M, pH 7.4), Triton (0.3%), and BSA (1%). The slides are then washed three times with PBS (0.1 M, pH 7.4) containing BSA (1%) for 5 min. The slices are then incubated with the secondary antibody (rabbit anti-immunoglobulins, 1/2000) for 2 hours at room temperature. After three washes, the slides were incubated for 1 h 30 min in PBS buffer containing Triton (0.3%) and the Avidin-biotine peroxidase complex (Kit ABC, Vector; 1/500). GFAP was revealed following the enzymatic reaction of the peroxidase in presence of the 3,3’diaminobenzidine (DAB; Sigma, Oakville,Canada; 0.03%) and hydrogen peroxide (0.006%) in Tris buffer (0.05 M pH 7.5). The sections were then collected, dehydrated and mounted in Eukit for optic microscopy observation. The specificity of the immunoreactive materials was tested following subjection of the slides to the same immunohistochemical procedure as described above and using the pre-immune serum or omitting of the primary antibodies. These tests showed that the primary antibodies for GFAP display specific labelling.
Locomotor activity: The “open field” test was used to evaluate the locomotor activity. It consists of a device of 25 identical squares made out of wood (20 cm per side). The animal is placed in the middle of the field and the ambulations (the number of squares traversed by animal) were record for 20 min.
Anxiety test: Animals are placed in a cage with two compartments, communicating with each other. One is enlightened and the other is obscure. With this test, we evaluate the time spent in each compartment for a period of 20 min. The relative % of time spent in each compartment is evaluated.
Statistical analysis: Statistics were performed by one-way analysis of variance followed by Dunnett's multiple comparison tests using the Graph Pad Prism 4.0 software (Chicago, USA). A “p” value less than 0.05 was considered statistically significant.
2.3 Results
After application of the anti-GFAP antibody in control rats, our data show a significant GFAP immunoreactive labelling in the cortex. Astrocytes with fine processes extend in all cortical layers (Fig. 1A). High magnification showed that the intensity of immunolabelling is limited (insert in Fig. 1A). In brains of chronically Al-intoxicated rats, GFAP immunoreactive labelling was intense compared with controls. The number of astrocytes is increased following Al treatment in animals treated in adulthood and since intra-uterine age (Fig. 1B, 1C and supplementary data). However, in this latter group astrocytes display prominent GFAP-immunoreactive cell bodies and processes (insert in Fig. 1C).
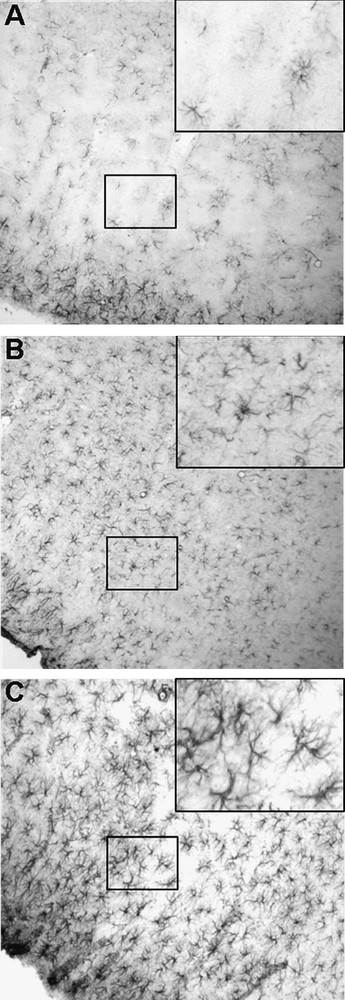
Immunohistochemistry using anti-glial fibrillary acidic protein antibody in the cerebral cortex of 4 months old control rats (A), and rats treated with Al in adulthood (B) or since intrauterine age (C). In controls the intensity of GFAP immunolabelling is limited (insert in Fig. 1A). Note that in chronically Al-intoxicated rats, the density of astrocytes is increased. In the group treated since intrauterine age, astrocytes display prominent GFAP-immunoreactive cell bodies and processes (insert in Fig. 1C). (×100).
Moreover, chronic intoxication by Al induced increased GFAP-immunolabelling in hippocampus particularly at the level of the dendate gyrus, the stratums radiatum and the granulosum, and in the cerebellum mainly at the level of the granular layer and the Purkinje cells (data not shown).
Control rats exhibit an important locomotor performance using the open field test which reflects a high exploratory activity in a new environment. Our results show that locomotor activity is significantly reduced in Al-treated groups either since intra-uterine age or in adulthood compared with controls (Fig. 2).
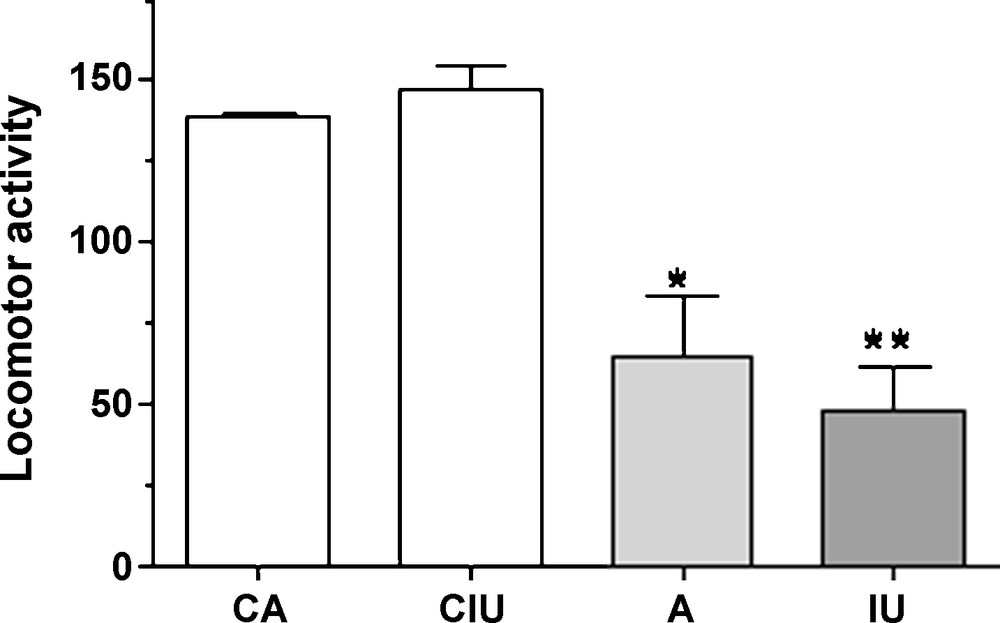
Locomotor activity for 20 minutes in 7 (CA) and 4 (CIU) months old control rats and in Al-treated animals either in adulthood (A) or since intrauterine age (IU). A significant decrease in locomotor performances was observed in Al-treated rats compared with controls.
While control rats prefer to remain in the dark compartment, those subjected to Al treatment in adult age show a slight decrease of the time spent inside the dark box which is not significantly different from controls. However, rats exposed to Al since intra-uterine age prefer to spend most of the time in the enlightened compartment (Fig. 3). Our data show that both astrocytic and behavioral changes are more important in rats exposed to Al since intra-uterine age. No significant changes in GFAP-immunlabelling (data not shown) and behavior have been observed between control groups (Figs. 2 and 3).
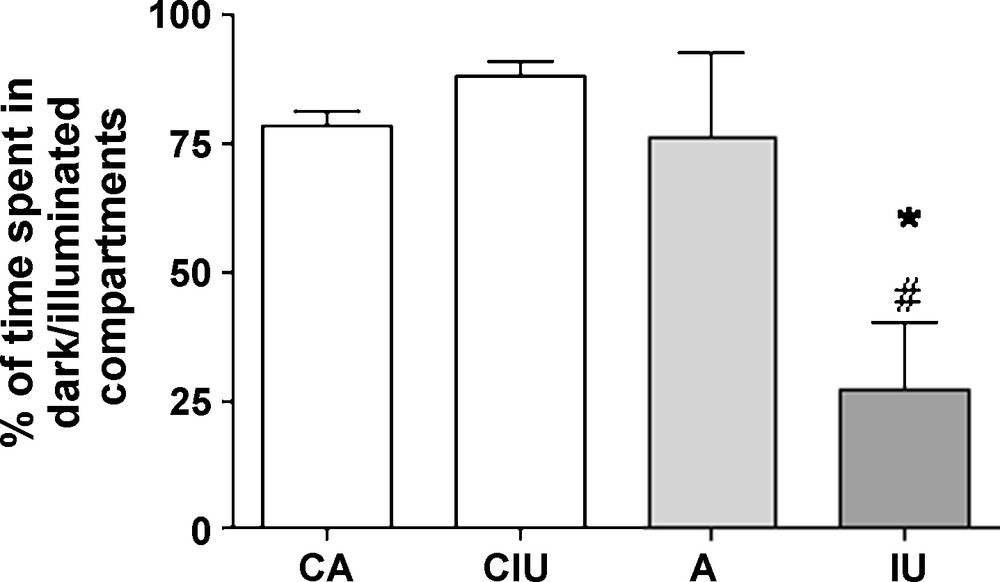
Percentage of time spent in the dark box versus the illuminated box in 7 (CA) and 4 (CIU) months old control rats and in Al-treated animals either in adulthood (A) or since intrauterine age (IU). A significant decrease of the time spent in the dark box was observed in IU-treated group compared with the other groups.
3 Discussion
In the present study we evaluate the effect of chronic exposure of rats to 0.3% AlCl3 solution on the behavior and the glial system of adult rats either treated in adulthood for a period of four months or exposed to Al since intra-uterine age followed by a postnatal Al-exposure period of four months. Our findings demonstrate that treatment of rats with Al induced a significant increase of GFAP-immunoreactive astrocytes particularly in the cerebral cortex. These findings support other previous reports showing increased GFAP content in frontal cortex of rabbits following Al exposure [11,14]. The latter study reported an increased expression of other marker of glial activation such as nestin and ED1 following treatment with nanoscaled aluminum oxide suggesting that exposure to Al produce inflammatory effects in the rat brain [14]. However, other studies reported either unchanged [15] or reduced GFAP in the frontal cortex [12] in rats. These discrepancies between our data and others could be related to different dosage of Al used, to the length of the treatment, or to the route of administration. In fact, previous studies reported effects on SNC following Al administration to animals intra-cerebroventricularly (icv) for up to 6 weeks [15], while others reported effects following administration of Al either intra-peritoneally (ip) or icv for up to 1 week [12]. In the present study we administered Al orally for a longer period (4 months). The fact that changes were apparent with different routes of administration of Al implies that sufficient levels of this neurotoxin may be able to cross the blood brain barrier. It seems that the duration or the doses used are the most likely to explain the extent of GFAP increase within the brain. In fact, while exposure of animals (either with 17 mg ip, or 0.27 μg icv) only for one week did not induce any increase of GFAP expression throughout the brain (rather they find a decrease) [12], treatment of animals for 6 weeks with low dose (5.4 μg icv) induce an increase of GFAP in thalamus but not the frontal cortex [15]. Moreover, Al-exposure of animals with a high dose of 10 mg for one month induced increased GFAP content in the frontal cortex but not the cerebellum or the hippocampus [11]. In our study we treated for a longer period (4 months) and with a higher doses (0,3%); a treatment that induced increased GFAP content in frontal cortex as well as in cerebellum and hippocampus.
It has been shown that the cerebral cortex, the hippocampus, and the cerebellum are the structures most implicated in the lesions induced by Al intoxication [16,17]. In the CNS, the extracellular environment of neurons is dependent upon astrocytes which are functionally connected via gap junctions [18]. Our study shows that Al induced a significant increase of the density of astrocytes and their processes forming a syncytium-like structure suggesting that Al may increase contacts between astrocytes within the brain and supporting the view that Al impairs gap junctions [19]. However, effects on GFAP are more important in rats exposed to Al since intra-uterine age with GFAP immunolabelling being more aggregated compared with changes observed in adults, suggesting that undifferentiated brains may be more susceptible to Al neurotoxicity. Following Al exposure, a previous in vitro study has reported altered GFAP immunoreactive content with an aggregation of GFAP-positive elements in the cytoplasm of astrocytes [19].
Several body of evidence suggests that Al is preferentially taken up by glial cells [9,10]. The prominent GFAP-immunoreactive cell bodies and processes seen in our study could probably reflect increased activity of astrocytes related with up-take of Al by these cells insuring thus a clearance of Al from the synaptic cleft. Glial changes seen as a consequence to Al exposure have probably effects on neuronal function. Previous studies showed that Al exposure induced morphological changes of neurons of the cerebral cortex in vitro [20]. The exposure of neurons to AlCl3 (10–100 μM) for one week did not cause neuronal death, but a significant neuritic processes degeneration which appeared probably as a consequence of conformation changes of the β-amyloid protein [20]. Moreover, Al is thought to induce cell death via activation of apoptotic mechanisms mediated through either mitochondrial or endoplasmic reticulum stress processes in rabbits [21]. In rats, a recent study found that Al induces changes in levels of lipid peroxidation products with consequences on synaptic morphology in the frontal cortex together with neurobehavioral changes [22]. Such changes induced by Al may mimic abnormalities that occur in neurodegenerative disease, such as Alzheimer's disease, including neuropathologic and behavioral changes [23]. In the present study we report that AlCl3 intoxication induces a significant reduction in locomotor activity of rats. These results confirm those found by other authors in Sprague-Dawley rats using the same test [24]. In adults mice Swiss-Webster, Al treatment induces a decrease of the total level of locomotor activity [25]. Reduced locomotor activity seen in our study may reflect either increased localized vertical movements such as rearing that would prevent animals from moving forward to cross squares of the open field or to anxiety. However, vertical activity is not increased as previously reported [26] suggesting that reduced activity may probably reflect anxiety as demonstrated by the dark/light test particularly in the rats subjected to Al since intrauterine age. However, using this test, adult rats exposed to AlCl3 did not show any significant behavioral change compared with controls probably due to less exposure to Al or to the relatively mild effects of Al on changes of the glial system compared with adults exposed since intra-uterine age, consolidating the view that differentiated brains are less susceptible to Al neurotoxicity. However, rats treated since intra-uterine ages, which are contaminated through the immature hematoencephalic barrier, exhibited more preference for the light compartment, suggesting that Al causes a state of anxiety in these rats. Other studies showed a significant reduction (> 80%) in the task passive avoidance performance in rats [27]. Our results are in agreement with the studies showing that exposure to Al affects the development and the behavior of rats [27,28].
The mechanisms by which Al induces these behavioral changes in animals are not yet known, but could be the consequence of glial changes with consequences on neurotransmission. For example, exposure to Al induces changes in brain levels of the neurotransmitter serotonin in several brain areas including the cerebral cortex and other brain regions [29]. Glial changes induced by Al toxicity may also induce consequences on neuronal systems involving other neurotransmitters such as dopaminergic [30], cholinergic [4], glutamatergic [5], and GABAergic [6] neurotransmissions. Moreover, Al may have the capacity to promote pro-oxidants events within the astrocytes by inducing nitric oxide synthetase (NOS) causing cell death within the rat brain [31], and to alter membrane associated proteins (Na–K ATPase and PKC) and endogenous antioxidant enzyme activity [7].
In conclusion, we report a high density of astrocytes following Al treatment in rats particularly those treated since intra-uterine age. Our findings show that Al affects the glial system and bring support for the role of Al in brain dysfunction that involves glial alterations.
Acknowledgement
This work has been funded by the PROTAS III: D14/71. SA is a receipt of the IBRO Return Home Programme, Grant 2009.
Appendix A Supplementary data
GFAP-immunoreactive cell count in the cerebral cortex of “4 months” old control rats (C), and rats treated with Al in adulthood (A) or since intrauterine age (IU). The numbers of GFAP-positive cells of each rat brain section were counted using high-power fields (400 × magnifications) for each section from 3 to 5 rats per group. In each field studied, only positive cells with the nucleus at the focal plane were counted.