1 Introduction
Medicago is an extensive genus of the Fabaceae, comprising approximately 83 different species and 18 infraspecific taxa [1]. Two-thirds are annuals and one-third perennials [2]. Annual species of the genus Medicago, commonly known as medics, are native to regions surrounding the Mediterranean Sea [3]. Due to their capacity of symbiotic nitrogen fixation, these plants are often used to improve soil fertility and particularly soil nitrogen content [4,5]. Annual medics combine traits such as vigorous seedling growth and cold tolerance with high forage quality, prolific seed production, resistance to pests and morphological diversity. They are considered as potential species for pasture improvement in the Mediterranean regions [6]. They could also be used as mother plants in row crop production [7], where they could suppress weeds and reduce soil erosion. However, medics exhibit dormancy due to the presence of an impermeable seed coat [8], which plays a determinant role in the germination pattern [9]. This coat-imposed dormancy, well known as “hardseededness”, is an ecological mechanism which allows the seed to germinate only when environmental can support seedling growth, but represents a limit when a prompt and high germination are required [10].
In Tunisia, the annual Medicago species grow spontaneously and represent an important proportion of the native flora [11]. Some medics are present along the country, while others are more endemic. Most of Tunisia is characterized by arid and semi-arid climatic zones, where drought, imposed by uncertain and scarce precipitation, is the major constraint for plant survival and growth. During evolution, many plant species have developed various mechanisms to enhance their drought adaptation. Some of the adaptive responses to water deficit correspond to changes in plant structure [12], growth rate and leaf gas exchange [13]. On the other hand, one of the most important responses of plants to drought and other abiotic stresses is the overproduction of different types of compatible solutes [14,15]. Among these solutes, proline and total soluble sugars are widely distributed and highly accumulate in drought-stressed plants [16]. This trait is considered to be an important component involved in the adaptive ability of various crop species [17,18]. It has been hypothesized that these solutes benefit stressed cells by acting as cytoplasmic osmolytes, thereby facilitating water retention [19], and by protecting and stabilizing macromolecules and structures from damage induced by stress conditions [20]. Although organic compounds are the major compounds of osmoregulation in plant cells during water deficit stress, inorganic ions, such as K+, would also contribute to the osmotic adjustment [21].
Since water availability is usually the main factor affecting productivity in dry regions, strategies aiming at improving sustainable use of water [22] and plant drought tolerance are urgent and should integrate conventional breeding and biotechnological approaches [23]. In this context, exploration of the variability in osmotic stress responses would permit not only to identify some tolerant genotypes, but also to determine useful criteria for genetic improvement of osmotic stress tolerance. As part of this approach, the present study aimed to investigate physiological responses to water deficit stress in two M. truncatula populations and two M. laciniata populations collected from contrasting Tunisian habitats. These differences in drought tolerance may be used as criteria for genotype selection in different climate regions. Moreover, interspecific differences are observed at the geographical distribution level. Medicago laciniata is restricted to the saharian, arid and semi-arid climates. Their plants are 15–35 cm long, with few branches arising from the base, procumbent to ascending. Leaflets are 4–15 mm long, 2–7 mm wide and peduncle has one to two flowers [2]. However, M. truncatula is abundantly present all over the country from the humid to the saharian stages. It is present on the heavy soils, marly or clayey [24]. These plants are 15–80 cm long, procumbent to ascending, branching close to the base. Leaflets are 8–27 mm long and 7–21 mm wide and peduncle has one to five flowers [2]. M. truncatula is considered as a legume model having characteristics that enable its use in molecular and genetic studies [25,26] and is recently emerging as promising molecular farming [27]. Transgenic approaches to improve M. truncatula drought tolerance using stress-related genes were recently initiated [28–30].
2 Materials and methods
2.1 Plant material and growth conditions
Two populations of M. truncatula (Mt-173 and Mt-664) and M. laciniata (Ml-173 and Ml-345) were collected from different geographical sites of Tunisia, taking annual rainfall as an indicator of environmental variability (Table 1). Seeds of each population were scarified with concentrated anhydrous sulphuric acid according to Araújo et al. [28], washed with distilled water and placed in Petri dishes with agarose at 9 g l−1. After vernalization at 4 °C for 24 h, Petri dishes were transferred in a growth chamber (25/16 °C, day/night) for 48 h. For each population, seedlings with uniform size were transferred in plastic pots filled with 10 kg limono-sandy soil. The soil field capacity (FC), measured according to the technique of Bouyoucos [31], was 9.38%. Five seedlings were transplanted per pot.
Collection sites of the four studied Medicago populations.
Collection site | Species | Code of populationa | Latitude and longitude | Annual rainfall (mm) | Bioclimatic stage |
Béja | M. truncatula | Mt-664 | 36°44′N 09°11′E | 664 | Sub-humid |
Enfidha | M. laciniata | Ml-345 | 36°08′N 10°23′E | 345 | Semi-arid |
Gafsa | M. truncatula | Mt-173 | 34°25′N 08°49′E | 173 | Inferior arid |
M. laciniata | Ml-173 |
a The letters are the species name initials and the numbers are the mean annual rainfall in millimetre.
Experiments were carried out in a greenhouse under the following conditions: average day temperature was 24 ± 8 °C, relative humidity was 50–63% and day length was 11–12 h.
2.2 Experimental design
After 26 days of ample watering (at 100% FC), the plants were divided in two lots: the first one was irrigated at 100% FC and the second one at only 33% FC. After 12 days of treatment, one lot of dehydrated plants was rewatered at 100% FC. Regular weightings (every 2 days) enabled to restore the moisture of soil at 100 or 33% FC. The plant weight was neglected. For each population and water regime, plants were harvested 24 days after the treatment was initiated.
2.3 Growth and water content measurements
For each population and water regime, leaf relative water content (RWC), fresh weight (FW) and dry weight (DW) of shoots and roots were determined. RWC was measured in the second youngest fully expanded leaves harvested weekly in the morning as follows [32]:
Turgid weight (TW) was obtained after soaking leaves in distilled water in test tubes for 12 h at room temperature (about 20 °C). Then, leaves were quickly and carefully blotted dry with tissue paper in preparation for determining TW. Dry weights of roots, shoots and leaves were measured after oven drying at 65 °C for 48 h.
2.4 Leaf gas exchange
Measurements of CO2 net assimilation (A), transpiration (E) and stomatal conductance (gs) were determined on fully expanded youngest leaves of each plant using a portable photosynthesis system (LCi). Measurement conditions were as follow: 1200 μmol m−2 s−1 photosynthethetically active radiation (PAR), 378 μmol mol−1 ambient CO2 concentration, and 26 ± 2 °C temperature of leaf chamber. These measurements were made from 12 to 13 h. Data were automatically collected every minute after photosynthesis rate had stabilised.
2.5 Cation assay, determination of proline and soluble sugars
Na+ and K+ were assayed by flame emission spectrophotometry after nitric acid extraction (HNO3, 0.5%) of the finely grounded dry matter. Free proline was qualified sectrophotometrically according to Baltes et al. [33], while the soluble sugars were determined by the anthrone reagent method according to Yemm and Willis [34].
2.6 Lipid peroxidation
The lipid peroxidation extent was estimated by determining the concentration of malondialdehyde (MDA) [35]. Leaf material was homogenized in 0.1% (w/v) TCA solution and centrifuged at 15,000 × g for 10 min. One millilitre of the supernatant obtained was added to 4 ml 0.5% (w/v) TBA in 20% (w/v) TCA. After, the mixture was incubated at 90 °C for 30 min; the reaction was stopped by placing the reaction tubes in an ice water bath. Samples were centrifuged at 10,000 g for 5 min, and the absorbance of the supernatant was read at 532 nm. The value for non-specific absorption at 600 nm was subtracted. The concentration of MDA was calculated from the extinction coefficient 155 mM−1 cm−1.
2.7 Statistical analysis
Data were subjected to an analysis of variance using the STATISTICA software 5.1H. Differences between mean values were determined by Duncan's method at P = 0.05.
3 Results
3.1 Plant growth
In control plants, total dry weight (TDW) was higher and root shoot ratio (RSR) was lower in M. truncatula populations compared to M. laciniata ones (Fig. 1). Water deficit stress caused a significant decrease of TDW and an increase of RSR in all studied populations. The greatest reduction rate of TDW was detected in Mt-173 (55%), followed by Mt-664 (51%), Ml-345 (42%) and Ml-173 (35%). In plants subjected to water deficit, RSR was significantly higher in M. laciniata populations than in M. truncatula ones. In rehydrated plants, TDW recovery was total in both M. laciniata populations, but partial in Mt-173 (77%) and Mt-664 (73%). Rewatering restored RSR to a similar level of control plants only in populations from the arid climate (Mt-173 and Ml-173).
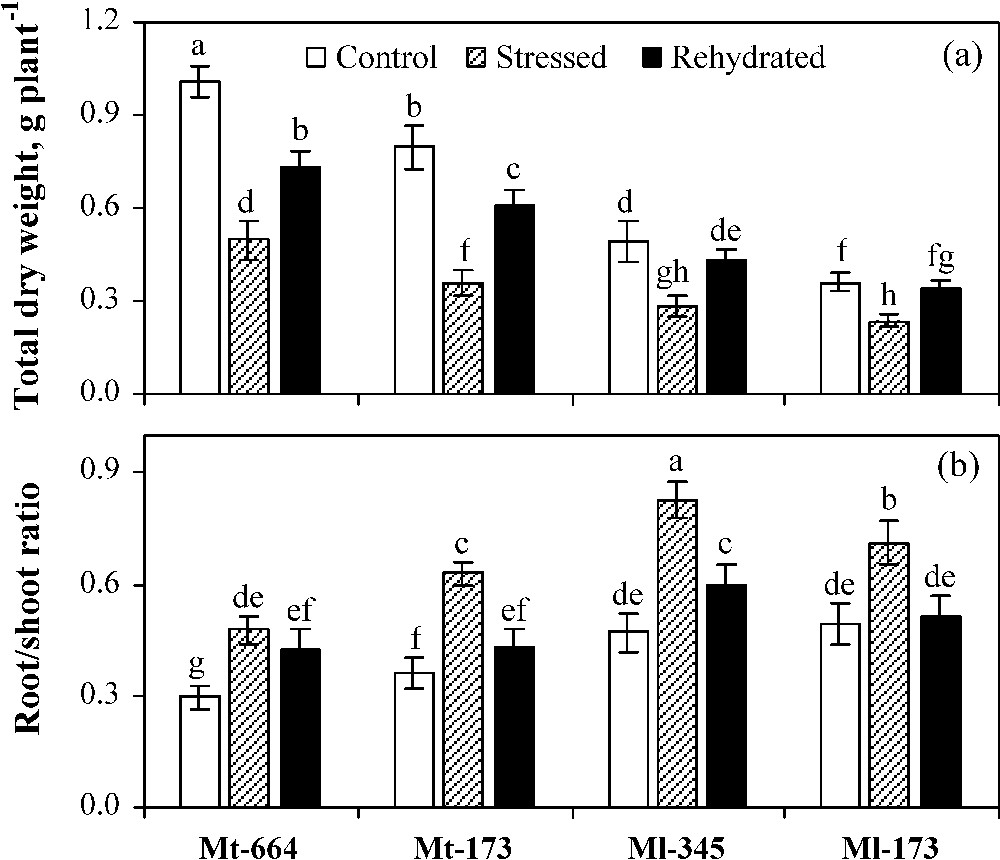
Total dry weight (a) and root/shoot dry weight ratio (b) in the M. truncatula populations (Mt-664 and Mt-173) and the M. laciniata populations (Ml-345 and Ml-173) affected by three water treatments: control, water stress and rewatering. Rewatering treatment was applied on plants previously subjected for 12 days of water deficit. Vertical bars represent S.E. of six to eight replicates. Values with the same letter are not significantly different based on comparison by Duncan's method at P = 0.05.
3.2 Leaf gas exchange
Under well-watered conditions, compared with M. truncatula populations, M. laciniata populations had significant lower A, gs and E and higher significant WUE (Fig. 2a–d). On the other hand, in each species, the lower leaf gas exchange and higher WUE values were registered for the population from arid climate.
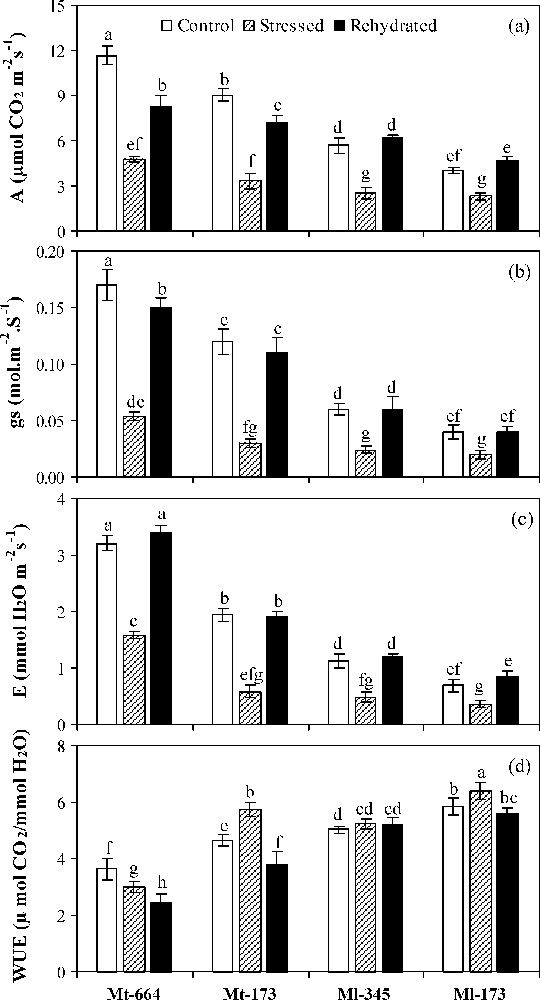
Net CO2 assimilation rate (a), stomatal conductance (b), transpiration (c) and water use efficiency (d) in the M. truncatula populations (Mt-664 and Mt-173) and the M. laciniata populations (Ml-345 and Ml-173) affected by three water treatments: control, water stress and rewatering. Rewatering treatment was applied on plants previously subjected for 12 days of water deficit. Vertical bars represent S.E. of nine replicates. Values with the same letter are not significantly different based on comparison by Duncan's method at P = 0.05.
Water deficit stress led to a significant decrease of A, gs and E in all studied populations. The highest reduction of A occurred in Mt-173 (63%), followed by Mt-664 (59%), Ml-345 (56%) and finally Ml-173 (43%). In all populations, gs reduction rate was more pronounced than for A. The lowest decrease of gs and E by water deficit was found in Ml-173 (50 and 48%, respectively). In stressed plants, WUE increased significantly in Mt-173 and Ml-173, while being unaffected in Ml-345, but decreased in Mt-664.
Upon rewatering, A recovery was partial in M. truncatula populations and total in M. laciniata ones. E and gs were restored to control values in all populations, except gs in Mt-664. WUE was significantly decreased in M. truncatula populations, but reached control values in M. laciniata populations.
3.3 Water relations
In control plants, leaf relative water content (RWC) was significantly higher in Mt-664 and Ml-345 than in Mt-173 and Ml-173 (Fig. 3a). However, the four populations showed similar OP values (Fig. 3b). Water deficit stress caused a significant decrease in RWC and OP of all populations. The reduction rate of RWC was the greatest in Mt-664 (29%) and the lowest in Ml-173 (16%). In stressed plants, the more negative values of OP were observed in Mt-173 (−1.65 MPa) and Ml-173 (−1.57 MPa). Transferring stressed plants on optimal irrigation restored leaf RWC and OP, which reached control values in all populations, except OP in Mt-664.
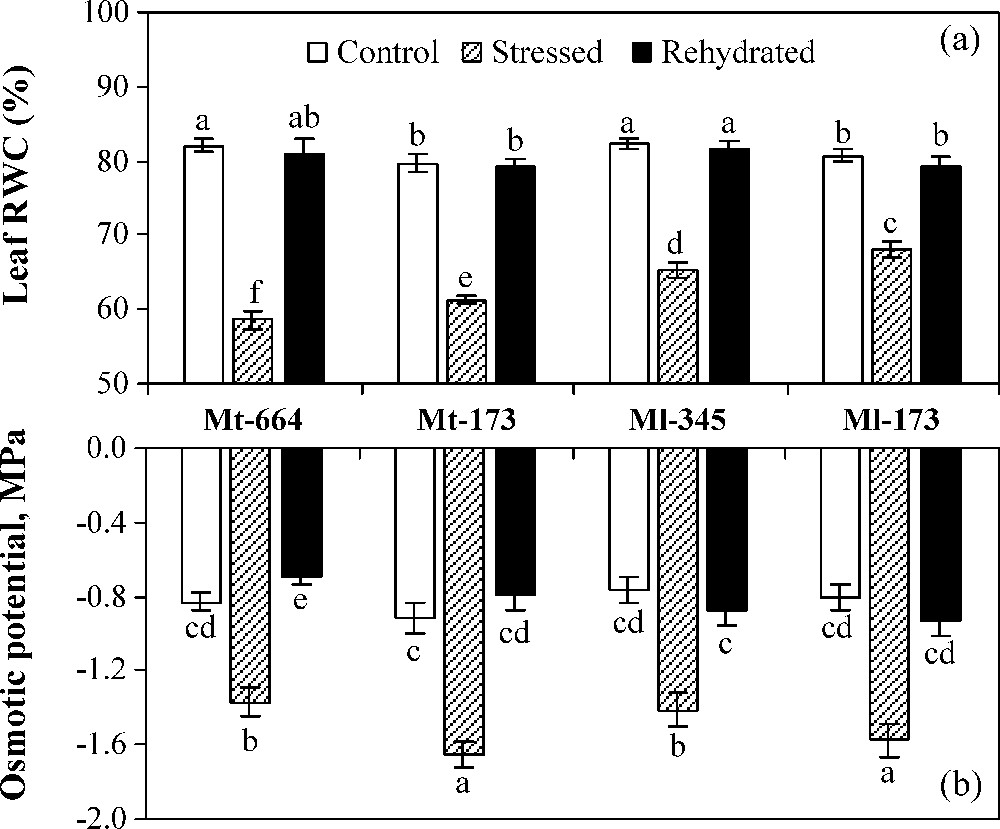
Leaf relative water content (a) and osmotic potential (b) in the M. truncatula populations (Mt-664 and Mt-173) and the M. laciniata populations (Ml-345 and Ml-173) affected by three water treatments: control, water stress and rewatering. Rewatering treatment was applied on plants previously subjected for 12 days of water deficit. Vertical bars represent S.E. of six to eight replicates. Values with the same letter are not significantly different based on comparison by Duncan's method at P = 0.05.
3.4 Solute accumulation
In control plants, no significant differences were found in proline and K+ concentrations between the four populations (Fig. 4a and c). However, soluble sugar and Na+ concentrations were significantly higher in M. truncatula populations compared to M. laciniata ones (Fig. 4b and d).
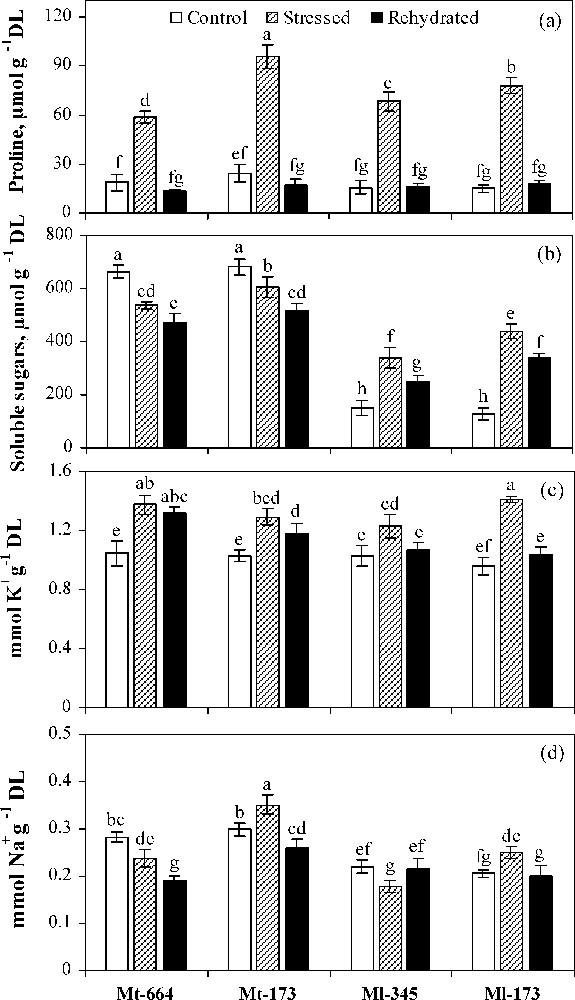
Proline (a), soluble sugars (b), K+ (c) and Na+ (d) concentrations in the M. truncatula populations (Mt-664 and Mt-173) and the M. laciniata populations (Ml-345 and Ml-173) affected by three water treatments: control, water stress and rewatering. Rewatering treatment was applied on plants previously subjected for 12 days of water deficit. Vertical bars represent S.E. of seven to nine replicates. Values with the same letter are not significantly different based on comparison by Duncan's method at P = 0.05.
Under water deficit conditions, proline and K+ were accumulated in all populations. Soluble sugar concentration was increased in both populations of M. laciniata, but was reduced in both populations of M. truncatula. Na+ concentration was decreased in Mt-664 and Ml-345 and increased in Mt-173 and Ml-174. Compared to control conditions, Ml-173 showed the highest increase in proline, soluble sugar, K+ and Na+ concentrations induced by water deficit.
In rewatered plants of M. truncatula populations, among the four solutes, only proline concentration was restored to the control level. However, for M. laciniata populations, except soluble sugars, all solute concentrations were close to the control level after rewatering.
3.5 Changes in membrane lipid peroxidation
MDA concentration was similar for the four populations under optimal watering (Fig. 5). MDA concentration was significantly increased in all populations under water deficit stress. The lowest increase was observed in Ml-173. After rehydration, although the MDA concentration decreased in all populations, it reached the control values only in M. laciniata populations.
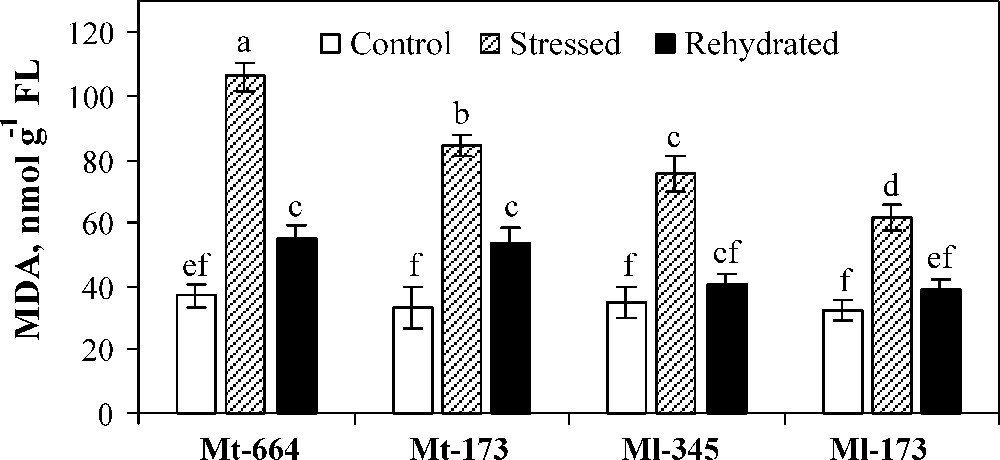
Malondialdehyde (MDA) concentration in the M. truncatula populations (Mt-664 and Mt-173) and the M. laciniata populations (Ml-345 and Ml-173) affected by three water treatments: control, water stress and rewatering. Rewatering treatment was applied on plants previously subjected for 12 days of water deficit. Vertical bars represent S.E. of six replicates. Values with the same letter are not significantly different based on comparison by Duncan's method at P = 0.05.
4 Discussion
4.1 Growth traits and leaf gas exchange
In the present study, M. laciniata populations were found to be more drought tolerant than M. truncatula ones. This was reflected by the relatively lower biomass reduction under water deficit conditions. The variability of drought tolerance between the populations of both species investigated was related to their photosynthetic activity (CO2 net assimilation rate) under drought stress. Indeed, a close relationship was found between A and dry biomass production (Fig. 6 a and b). Such a positive correlation between growth and photosynthetic capacity has also been found in a number of species, e.g., Zea mays [36], Phaseolus vulgaris [37], Gossypium barbadense [38] and Panicum antidotale Retz. [39]. In the four populations, water deficit decreased more gs than A, and the both variables showed a statistically significant relationship (Fig. 6c and d). As it is, the reduction of A in stressed plants may be ascribed mainly to the reduced CO2 supply due to stomata closure. One of the earliest responses to drought is stomatal closure. Stomatal closure allows plants to limit transpiration, but it also limits CO2 absorption, which leads to a decreased photosynthetic activity [40,41]. The stress tolerance of M. laciniata populations to water shortage appears to be associated with their low intrinsic growth rate and stomatal control of gas exchange. To this topic, Kramer and Kozlowski [42] signalled that, generally, plants with greater drought tolerance are featured with a higher survival rate, better water status, slower gas exchange and slower intrinsic growth rate. The advantage of cultivars with a slower growth in harsh environments is that they have low demands and therefore will not exhaust the limited soil water reserve [43]. The response of the four populations to water deficit stress would be also related to the preferential allocation of dry matter towards roots. Indeed, in plants subjected to water deficit, RSR was higher in M. laciniata populations than in M. truncatula ones. This parameter is considered as a criterion of adaptation to drought [44,45], although some findings showed no clear correlation between root trait and water extraction ability [46,47]. Several studies have reported the increase of root/shoot ratio, under water deficit, in several crops, cultivated pasture, desert and Mediterranean annual species [48–50]. Interestingly, the recovery of TDW and A by rehydration were total in 12-day stressed plants of both M. laciniata populations, but less pronounced in Mt-664. This result suggests that in M. laciniata populations both the dark and the light reactions of photosynthesis had not been damaged during the stress period.
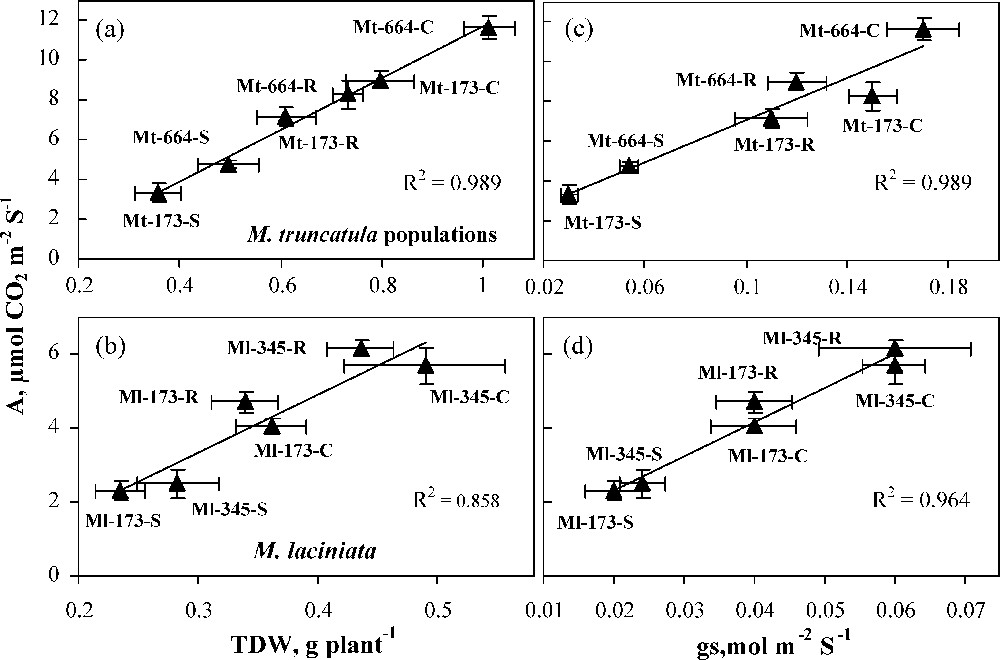
Relationship of net CO2 assimilation rate (A) with total dry weight (TDW) (a and b), and stomatal conductance (gs) (c and d) in populations of the two Medicago species under control (C), stress (S) and rewatering (R) treatments. Bars represent ± SE.
For M. truncatula, water deficit stress restricted more TDW, A, gs and E in the population originating from arid climate (Mt-173) than in that from sub-humid climate (Mt-664). However, under water deficit stress, RSR and WUE were higher in Mt-173 compared to Mt-664. The observed differences in morphological and physiological responses may explain specific adaptation mechanisms in species subjected to water deficit. In agreement with our results, it was reported that the populations of Eucalyptus microtheca [51] and Populus davidiana [52] from dry climate (which grow under a natural habitat of prolonged annual drought), grew slowly under different watering treatments, presented a higher RSR and the lowest values of total dry matter, net CO2 assimilation rate, stomatal conductance and transpiration. All these responses enable the plants to overcome prolonged drought. In contrast, for M. laciniata, the decrease of TDW, A, gs and E induced by water deficit was lower in the population from the arid climate (Ml-173) than in the population from the semi-arid climate (Ml-345). RSR was lower and WUE was higher in Ml-173 compared to Ml-345. Previous studies indicated that WUE is a desirable trait to utilise in crop improvement programmes for water-limited environments [53,54].
4.2 Water relation and osmolyte accumulations
Leaf RWC of well-watered plants varied between 79.6 and 82.3%. However, as a result of 24 days of drought stress, this parameter decreased in all populations, and was ranged between 59% (in Mt-664) and 68% (in Ml-173). The utilization of leaf RWC as an indicator of plant water status [55,56] and plant drought tolerance [57,58] is usual. In Aeluropus lagopoides, leaf RWC was significantly correlated with relative growth rate, net photosynthesis rate, chlorophyll, proline and soluble sugar contents [59]. Leaf OP declined in response to water stress in the four populations. The decrease of OP is generally considered to be an indicator of osmotic adjustment through the production and/or accumulation of some called compatible osmolytes [16,18], although such decreases could also result from a dehydration of the tissue and/or a reduction of the osmotic volume [60]. In stressed plants, the more negative values of OP observed in Mt-173 (−1.57 MPa) and Ml-173 (−1.48 MPa) were not associated with the larger leaf dehydration. This result suggests the involvement of solutes in lowering OP. Osmotic adjustment is the main component of physiological machinery, by which plants respond to soil water deficits [61,62]. Plants with higher osmotic regulators can absorb water from dry soil. In wheat, many reports showed that wheat cultivars with higher K+, proline and soluble sugars at different growth stages were more drought resistant [63,64].
Proline concentration increased in the four populations under water deficit, the highest increase rate being observed in Ml-173 (523.7%). Proline accumulation is an important indicator of drought stress tolerance in bacteria, algae, and higher plants [65,66]. In addition to its role as a compatible compound for osmotic adjustment, proline contributes to stabilizing sub-cellular structures (e.g., membranes and proteins), scavenging free radicals, and buffering cellular redox potential under stress conditions [67,68]. It may also function as a protein-compatible hydrotrope, alleviating cytoplasmic acidosis, and maintaining appropriate NADP+/NADPH ratios compatible with metabolism [69]. Also, rapid breakdown of proline upon relief of stress may provide sufficient reducing agents that support mitochondrial oxidative phosphorylation and generation of ATP for recovery from stress and repairing of stress-induced damages [70]. Under different water treatments, in populations of each Medicago species, a significant correlation was found between proline and osmotic potential (Fig. 7), suggesting the involvement of this solute in osmotic adjustment.
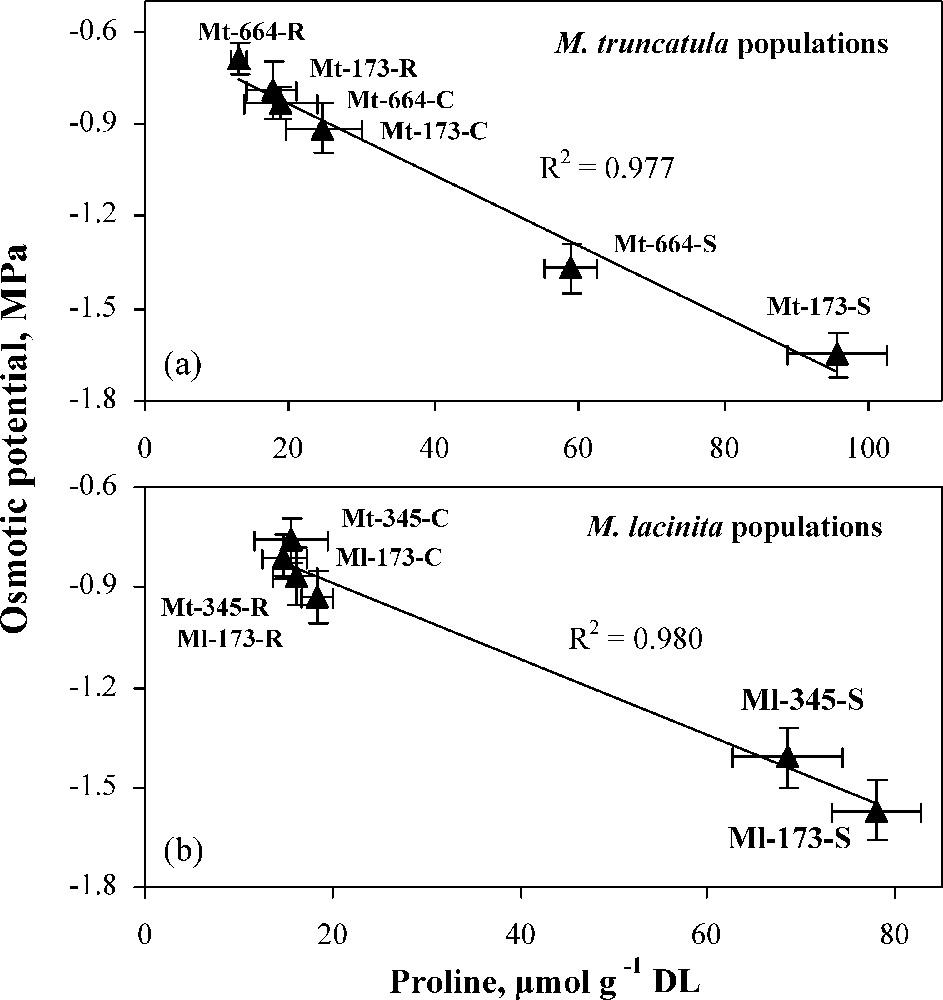
Relationships between osmotic potential and proline concentration in M. truncatula populations (a) and M. laciniata populations (b) under control (C), stress (S) and rewatering (R) treatments. Bars represent ± SE.
The accumulation of soluble sugars in plants in response to water deficit stress is quite well documented and is considered to play an important role in osmotic adjustment [71,72]. According to the Van’t Hoff equation, the measured sugar concentration represented between 34 and 46% of the osmotic adjustment observed, depending on cultivar [73]. In our experience, under water deficit conditions, soluble sugar concentration increased in both populations of M. laciniata but decreased in both populations of M. truncatula.
Although organic compounds are the major constituents of osmoregulation in plant cells during water stress, inorganic ions (e.g., K+) would also contribute to the osmotic adjustment [21]. K+ was found to be one of the major contributors to the osmotic potentials in water-stressed maize plants [74]. Synthesis and accumulation of organic solutes consume more energy than uptake of inorganic ions [75]. Our results showed that in response to water deficit stress, K+ concentration increased in all populations with a higher increase rate in Ml-173. However, Na+ concentration significantly increased under water deficit stress in both populations from the arid climate, but it decreased in the other populations.
4.3 Lipid peroxidation
Malondialdehyde (MDA) concentration has been associated with damages caused by exposure to a variety of environmental stresses [76]. It is a common and an important index for evaluating the status of wheat redox [77]. Lower MDA displays higher anti-oxidative ability, reflecting higher resistance to drought [78,79]. M. truncatula populations were more affected by water deficit stress than M. laciniata ones, and in each species, the population from the arid area showed a higher tolerance to water deficit stress.
4.4 Conclusion
In conclusion, our results indicate that water deficit stress restricted growth of M. truncatula and laciniata populations independently of their origins. However, M. laciniata populations were more tolerant to water deficit than M. truncatula ones, as shown by their relatively lower biomass reduction under water deficit conditions. The higher tolerance of M. laciniata populations to water shortage may be related to their lowest intrinsic growth rate and stomatal control of gas exchange. TDW was less reduced by water deficit in Ml-5 than in Ml-345. The differential reduction of TDW between the two populations was related to their differential reduction in net CO2 assimilation under water deficit. Drought tolerance of Ml-173 was found to be associated with a relatively higher decrease of OP and a lower reduction in RWC due to the accumulation of solutes such as proline, soluble sugar and K+. Ml-173 exhibited also a better adjustment of A and E, and the highest WUE values under water deficit.
Acknowledgements
This work was supported by the Tunisian Ministry of Higher Education, Scientific Research and Technology (LR02CB02) and the Tunisian-French “Comité mixte de coopération universitaire” (CMCU) network # 08G0917.