1 Introduction
While the vast majority of eukaryotes reproduce sexually, involving meiosis at each generation, a small category of diverse and intriguing organisms exists that deviate from the general rule by using alternative modes of reproduction. Briefly, these reproductive strategies range from a combination of sexual and asexual reproduction in the organism's life cycle to strict clonal reproduction with no recombination events, as far as is known [1]. This diversity in reproductive mode can be expressed at two levels. Firstly, facultative asexual organisms use both sexual and asexual reproduction depending on environmental conditions. This means that specific environmental cues trigger a change in reproductive mode within the same individual genotypes, involving phenotypic plasticity and relying on changes in expression of genes that regulate the observed reproductive orientation. Canonical examples of facultative asexual animals are found in cladocerans, insects, mites and trematodes [2]. Secondly, asexual and sexual reproduction can coexist at the population level. In this case, reproductive mode variation relies on a polymorphism whereby genetically determined sexual and asexual lineages coexist within the same species [3].
Reproductive mode variation at these two levels raises questions not only in relation to its evolutionary and ecological significance but also on the regulatory mechanisms of reproductive plasticity involved at the molecular level. Furthermore, how exactly polymorphism and phenotypic plasticity are related evolutionarily or functionally remains unclear [4,5]. Here, we propose to review these various aspects on aphids which are among the few organisms to use both sexual and asexual reproduction within the same genotype and involving reproductively specialized lineages (Fig. 1).
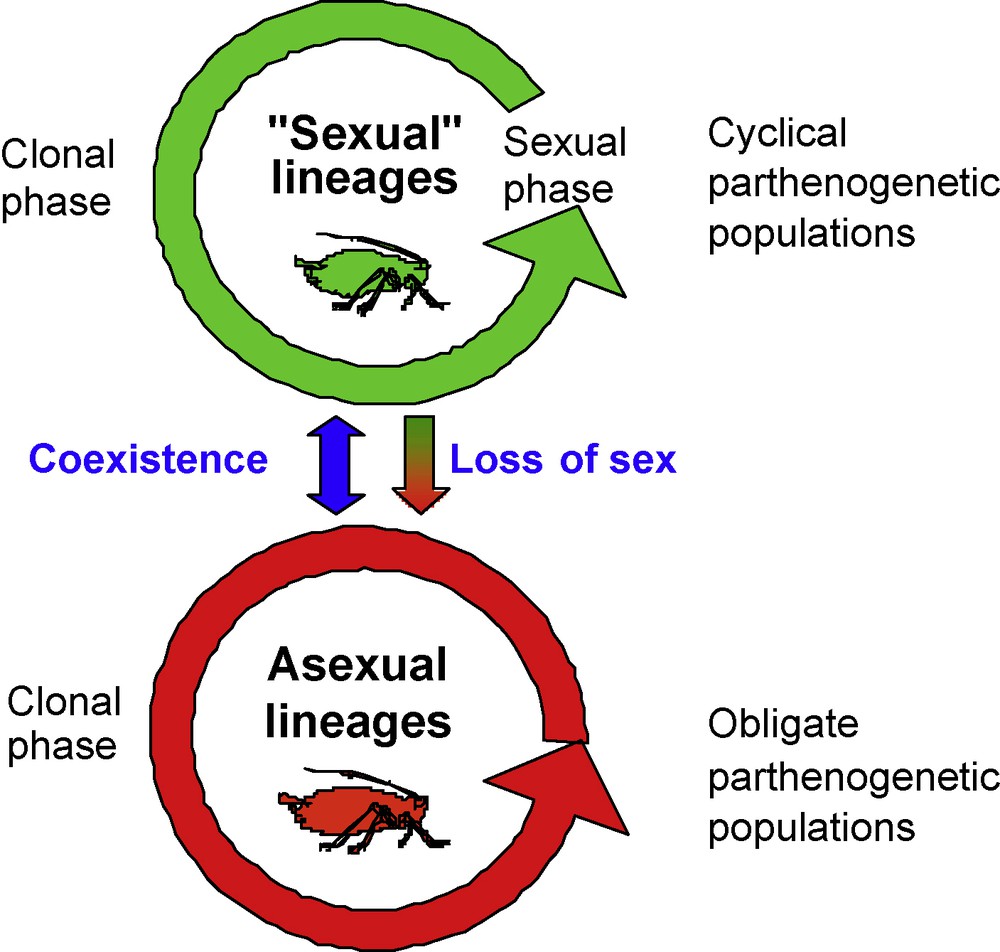
Simplified representation of the two types of reproductive mode variation expressed in aphids. “Sexual” lineages are cyclical parthenogenetic populations that regularly alternate a clonal and a sexual phase in their annual life cycle. The shift in reproductive mode is determined by environmental factors. Asexual lineages may coexist with “sexual” lineages within the same aphid species and refer to obligate parthenogenetic populations that have abandoned the sexual phase in their life cycle.
2 Alternation of clonality and sexuality is the typical aphid reproductive mode
Aphids form a monophyletic group that evolved around 280 million years ago (late Carboniferous or early Permian) [6] and are presently represented by around 4500 species worldwide. The vast majority of aphid species propagate by apomictic parthenogenesis (clonal or asexual reproduction) as the main or exclusive mode of reproduction. Asexual reproduction was therefore seemingly acquired in the very early steps of aphid evolution, presumably in an all-sexual ancestor, and has been kept in association with sexual reproduction until now [7]. The typical aphid reproductive mode indeed involves a succession of usually numerous parthenogenetic generations, followed by a single sexual one within the annual life cycle [8,9]. This reproductive system is also known as cyclical parthenogenesis in animals and is found in other invertebrates such as cynipid wasps, midges, water fleas (Daphnia) and a wide range of trematodes [2]. The asexual phase of aphids occurs during the growing season (spring and summer) and up to 20 asexual generations can be produced if climatic conditions are favorable. In addition, asexual reproduction in aphids is associated with viviparity and telescoping of generations [7]. The former trait means that a newly born aphid has already started to develop within mother's abdomen, in contrast with oviparous organisms which lay eggs that generally hatch after a certain delay. The latter trait is more specific to aphids and refers to the fact that an adult viviparous female not only contain the next generation as fully developed embryos, but also the subsequent one as early embryos within these developed embryos. Asexual reproduction associated with viviparity and telescoping of generation confers aphid populations with an extremely high rate of increase, leading sometimes to outbreaks of billions of individuals within a single field. Therefore, aphid reproductive mode is a key component of the damage caused by these economically important crop pests [10].
The sexual generation is produced in late summer in response to shortened day length. Parthenogenetic females give birth to sexuparae (pre-sexual females) which in turn produce wingless oviparous sexual females and males (winged or wingless depending on species). After mating, sexual females lay frost-resistant eggs that hatch the following spring after several months of diapause [11]. New parthenogenetic females arise from these sexually produced eggs and generate clonal offspring that give birth in turn to other parthenogenetic generations, until the next sexual episode. Asexual and sexual phases generally occur on plants of the same genus or family. In 10% of aphid species however, clonal generations develop on herbaceous hosts while sexual forms mate on woody plants [12]. Cyclical parthenogenesis in such species is thus associated with host alternation, resulting in large population reshuffling induced by obligate dispersal between unrelated hosts (see [13] in this issue for more details on host alternation in aphids). Thus, in theory, aphids combine the best of both worlds in their life cycle. Asexual viviparous reproduction ensures high population increase during the growing season, natural selection giving the fittest genotypes a considerable demographic advantage due to sustained clonality. Oviparous sexual reproduction leads to the production of genetically recombined individuals via cold resistant forms, the eggs, which are absent in the asexual phase of Aphididae (but still present in the sister families Adelgidae and Phylloxeridae), allowing aphid populations to resist harsh winters and to produce an array of new genotypic combinations each year ([7,9] for further details on aphid life cycles).
3 From clonal to sexual reproduction in response to seasonal photoperiodism
It is known from the work of Marcovitch [14] that the shift from clonal to sexual reproduction in aphids is triggered by photoperiodic changes, although temperature mitigates the photoperiodic response in some cases. Clonal aphids perceive the increase in night length (scotophase) that occurs by late summer and throughout autumn. They respond to this photoperiodic change via a transgenerational process involving production of sexual forms (oviparous females and males). Aphids are thereby able to measure accurately the length of the scotophase, and a critical number of consecutive dark and light periods are needed for inducing the production of sexual forms. Scotophase measurement is presumably achieved by an internal clock and counter system, which has to be identified [15]. The photoperiodic signal is detected directly by the aphid brain or via the visual system through the head cuticle, although embryos might also sense environmental cues through their mother's abdomen [16]. It has been shown experimentally that a group of neurosecretory cells localized in the anterior dorsal region of the protocerebrum (pars intercerebralis) is required for perception and early transduction of the photoperiodic signal [17]. How the signal is then transferred to the ovaries for orientation of the reproductive pathways (clonal vs. sexual) is still unresolved but probably involves at some stages hormones of the endocrinal system such as melatonin or juvenile hormones [18–20].
The final consequences of this photoperiodic response take place in the aphid reproductive tract, more specifically in the germ cells or oocytes located in the germarium of presumptive embryos. Under short night conditions, asexual reproduction is promoted: embryos of parthenogenetic females develop within the ovariole from a diploid oocyte after a single modified mitosis division whereupon bivalent chromosomes are paired and aligned through metaphase but maintained as separate strands along their length (i.e. there is no chromosome recombination as such) [21]. By contrast, long night conditions induce the production of sexual females which contain oocytes blocked in metaphase I of the meiotic division. Males are also produced under long nights by a modified mitosis, but at the end of the division, one X-chromosome is eliminated [22]. Males thus differ from females by their chromosome set which contains one X-chromosome but the same set of autosomes. All these morphs proceeding from the same mother are predominantly genetically identical (although it is now known that mutations frequently arise in such lineages, even at short generation times [23,24]). The progeny resulting from mating of these sexual morphs forms an array of diverse parthenogenetic lineages that will expand the next spring.
4 Early progress on regulatory mechanisms of reproductive mode variation in aphids
Much of what is known on the physiological aspects of reproductive orientation in aphids dates back to the 1970s and early 80s, especially due to the pioneered works of A.D. Lees and colleagues at Silwood Park, Imperial College, London. Recently, through the initiative of the International Aphid Genomics Consortium (IAGC) the shared efforts of the aphid scientific community have allowed the acquisition of considerable genomic and post-genomic resources for the pea aphid, Acyrthosiphon pisum (Harris) as a model system, including the recent annotation of its complete genome, allowing searching for candidate genes and determination of putative gene functions by genome-wide comparisons [25]. These resources have been used to tackle different aspects of aphid biology ([26], this issue), including their peculiar reproductive mode. So far, genomic and functional analyses on reproductive plasticity in aphids have mainly concentrated on the early steps of photoperiod signal transduction. Hereafter, we present an overview of the main findings obtained on the regulation of reproductive mode variation in the pea aphid (Fig. 2).
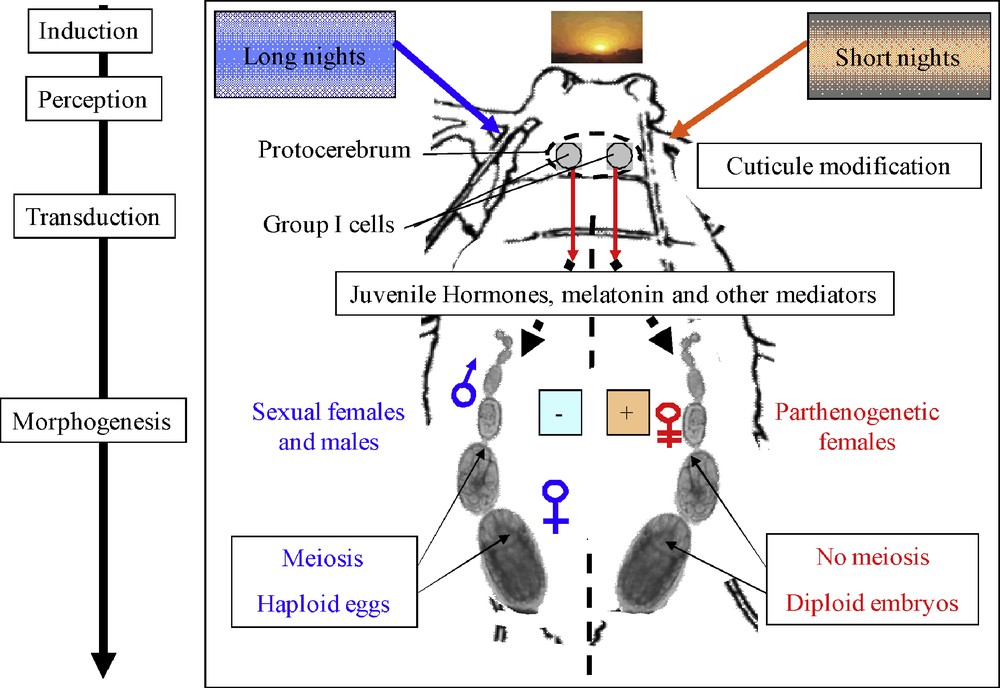
Schematic representation of the early steps of sensing and transduction of the photoperiod in aphids. Night length is responsible for the fate between the production of oviparous sexual females and viviparous parthenogenetic females. The photoperiod signal is sensed in the head and the transduction occurs through a group of neurosecretory cells. Neuroregulation probably triggers hormonal modifications that will be sensed by targeted cells in the ovarioles.
Since the aphid brain is considered as the center of photoperiod signal reception and early transduction, comparative gene expression analyses and protein profiling have been made on aphid heads exposed to either long (sex induction) or short (clonal induction) night regimes. Changes in gene expression and protein profiles were studied during the course of the transgenerational process, tracking variation at different developmental stages of the two generations involved [16,27]. In addition, more specific analyses have been conducted on candidate regulatory mechanisms that could control reproductive plasticity in the pea aphid. These include the micro RNA machinery and the epigenetic DNA methylation system [28,29] which are both known to be involved in caste determination in social insects [30,31].
4.1 Visual and photoreception systems
Aphid photoreceptors occupy an extra-optic situation and locate in a light-sensitive region in the protocerebrum, beneath the cuticle [17,32]. However, none of them has yet been characterized and a location of such receptors in the eyes is still not completely ruled out [16]. Transcriptomic analyses of aphid heads exposed to contrasted photoperiodic regimes allowed detection of several regulated genes implicated in the photoreception or visual systems. These studies suggest a particular involvement of rhodopsins (visual pigments) in response to day length shortening, in both the compound eyes and the protocerebrum [33]. The photoactivation of rhodopsins by the light source might therefore well play a role in the transduction of the photoperiodic signal responsible for reproductive shift from asexuality to sexuality.
4.2 Unexpected changes in cuticular transcripts in response to photoperiod
Three independent transcriptomic studies on aphid heads or whole bodies exposed to either short or long nights unexpectedly identified cuticular proteins as being regulated by photoperiodic changes [16,27,34]. Cuticular protein genes were among the most highly regulated, particularly those coding for cuticular proteins that contain chitin-binding domains and whose expression level was up or down to 500 fold. Transcriptomic data thus suggest a softening of the cuticular network between cuticular proteins and chitin that occurs in the heads of aphids reared under short-days [16]. This change in cuticle structure with photoperiodism could be interpreted in two ways (reviewed in [35]). Firstly, aphids perceived photoperiodic variation in a light-sensitive region containing putative photoreceptors and located very close to the surface of the brain. Cuticle modifications mediated by the photoperiod signal could therefore affect the quality and quantity of light through change in cuticle filtering characteristics, resulting in different reproductive orientations. Secondly, the cuticle stores several metabolites among which N-β-alanin dopamine (NBAD) is responsible for cuticle hardening or sclerotization. Transcriptomic analyses show that NBAD biosynthetic pathway is down-regulated in aphids exposed to long nights, suggesting a modification of dopamine concentration in the aphid brain in response to photoperiod. Dopamine plays a critical role in melanization and sclerotization of the cuticle, as well as in neurotransmission. Complementary experiments involving semi-quantitative PCR showed that genes required for dopamine biosynthesis are down-regulated in long night reared aphids, arguing for a down-regulation of the dopamine concentration in aphids giving birth to sexual individuals [35].
4.3 Transduction of the photoperiod signal through the neuro-endocrine system
Global transcriptomic approaches also detected changes in expression in genes involved in the nervous system in response to photoperiodic cues. Several regulated genes showed homology to proteins associated with axon guidance or nervous system development [16]. In particular, the expression of two genes of the insulin pathway is affected by shortening of photoperiod: a putative insulin receptor transcript is down-regulated and a degrading insulin enzyme transcript is up-regulated. These results suggest a down-regulation of the insulin pathway in long night reared pea aphids. In Drosophila melanogaster, a decrease in the insulin pathway is correlated to a decrease in the juvenile hormone pathway [36]. In addition, the insulin pathway is regulated during diapause of Culex pipiens also induced by photoperiod shortening, in relation with the juvenile hormones pathway [37]. As juvenile hormones regulate photoperiod signal transduction in aphids [20], it is possible that insulin acts as an upstream regulator of juvenile hormones in long night conditions. Bioinformatic analyses of the pea aphid genome identified 10 different genes coding insulin-related peptides [38]. Molecular analyses are currently underway to check whether some of these insulin genes are regulated during the switch of reproductive mode.
Rapid progress has thus been made on hypothetical genetic programs and biosynthetic pathways involved in the photoperiod response and in the switch of reproductive mode. Cauterization of secretory cells impairs the production of parthenogenetic morphs under short night conditions. Thus, the default mechanism, when the neurosecretory cells do not function, seems to be the production of sexual morphs, even in short night. One hypothesis is that parthenogenesis under short night has been acquired by the capacity of cells to secrete molecules that change the fate of the oocytes (Table 1). More functional analyses on these different pathways are required to test these hypotheses.
Hypotheses on the molecules regulating the switch of reproductive mode in aphids in response to photoperiodic changes.
Sexual reproduction | Parthenogenesis | Reference |
Short nights | Long nights | Marcovitch, 1924 [14] |
Oviparous females | Viviparous females | – |
Meiosis | No meiosis | Blackman, 1987 [21] |
Low level of juvenile hormones | High level of juvenile hormones | Corbitt and Hardie, 1985 [18] |
Low level of melatonin | High level of melatonin | Gao and Hardie, 1997 [19] |
Low level of insulin | High level of insulin | Le Trionnaire et al., 2009 [16] |
Low level of dopamine | High level of dopamine | Le Trionnaire et al., 2009 [16] |
5 Loss of sex in aphids: reproductive mode variation at population and species levels
Although cyclical parthenogenesis (i.e. regular alternation of clonal and sexual generations) was acquired in the very early steps of aphid evolution, a few species, representing about 3% of the aphid fauna, appear to reproduce by permanent all-female parthenogenesis [9]. These asexual species have therefore secondarily lost the sexual phase from their life cycle and are insensitive to photoperiodic changes that normally trigger the production of sexual forms in most other aphids which have a facultative life cycle. In addition, many species (30–40% of the total) show a variation in reproductive mode within or between populations [8]. This variation ranges from lineages with a regular alternation of clonal and sexual reproduction each year (referred to as sexual lineages for simplicity) to lineages that have completely abandoned sex and persist as pure clonal populations (referred to as asexual lineages). In between, lineages with different investments in sexual reproduction and sex-ratios can be found along the continuum [39].
The possible coexistence of sexual and asexual lineages within the same species and even the same population raises questions about the selective advantage gained by sexual lineages to compensate for their lower population growth rate. Indeed, sexual lineages pay the general two-fold cost of sex because of male production [40], and more specific to aphids, they suffer another demographic drawback over asexual lineages because of their development arrest as overwintering eggs. By contrast, asexual lineages potentially multiply all year round as all-female populations, and because they have no need to seek sexual partners, have, in theory at least, a significant fitness advantage over sexual lineages.
This seeming paradox of sexual maintenance despite lack of competitiveness is not specific to aphids and has been the subject of much debate in recent years and a vast literature now exists on the topic (see, for example, [41] for a review). So far, there is no general agreement as to the resolution of this paradox, although a plethora of hypotheses have been proposed involving deleterious mutation elimination, adaptation to environmental changes, as well as changes in chromosome telomere length and re-setting [42]. Because of the frequent coexistence of sexual and asexual lineages in many distinct species, aphids represent an excellent system to test some of these explanations and to address the evolution and adaptive potential of sexuality and asexuality.
6 Sexual reproduction provides aphids with ecological advantages
Resolving the paradox of sex in aphids requires, as for a more general understanding, consideration of the evolutionary process, both in the short- and the long-term [9]. In cyclically parthenogenetic aphid populations or “sexual” lineages, the sexual phase that takes place by late summer involves the production of sexual forms from clonal females, and egg-laying by mated oviparous sexual females, as earlier mentioned. Since these overwintering eggs are frost-resistant and can survive temperatures as low as −35 °C [11], sexual reproduction in aphids is in some way linked with cold resistance [22]. By contrast, aphids spending winter as live parthenogenetic individuals such as those found in asexual lineages, are susceptible to frost because they do not resist temperatures below −8 °C or −10 °C [11]. With this difference in mind, we expect sexual lineages to be favoured in climatic zones with regular harsh winters, whereas asexual lineages should dominate in zones with mild winter temperature. In areas with fluctuating winter severity, mixed (bet-hedging) reproductive strategies would be promoted, as predicted by modeling works [43,44]. These predictions have now been validated in several aphid species for which the distribution of reproductive variants have been assessed along climatic gradients or in zones with contrasted climatic conditions [45–47]. In addition, we expect that the distribution of sexual and asexual lineages would vary from year to year depending on the harshness of preceding winter, a prediction that has been recently confirmed in the cereal aphids Sitobion avenae (F.) and Rhopalosiphum padi (L.) [48,49].
From the above theoretical and empirical studies, it is clear that aphid reproductive strategies are predominantly selected for by winter climate. However, winter climate alone hardly accounts for the frequent local coexistence of sexual and asexual aphid lineages exposed to the same climatic regime. Ecological factors other than climate may further act on reproductive mode variation, such as environmental heterogeneity, including variation in biotic interactions e.g. parasitic pressure [50,51]. This seems to be the case for sympatric host adapted races of the pea aphid, A. pisum which differ in terms of sexual investment, those living in spatially and temporally heterogeneous habitats being the most sexual [50]. Similarly, it has been found that aphid crop pests show a higher incidence of asexuality, a result that could be linked with environmental uniformity and reduced complexity that especially characterize current agricultural landscapes [49].
7 The evolutionary fate of asexually reproducing lineages of aphids
While asexual lineages of aphids are counter-selected in zones with regular harsh winter, they can show high ecological success in vast areas with a mild climate (e.g. oceanic or tropical climates). Several papers reported in various aphid species the existence of asexual lineages represented by few highly frequent clones distributed over large geographic and ecological ranges [39,52,53]. These dominant clones also termed “super-clones” may also persist for more than a decade [49,54]. However, the replacement of asexual genotypes i.e. clonal turn-over by fitter ones, is also commonly found in these studies, demonstrating that recombination and genetic diversity provide a greater adaptive potential than plasticity alone [39]. Although we mentioned earlier that no general consensus has emerged for explaining the short-term maintenance of sex, there is a large agreement on the forces favoring sexual reproduction on longer evolutionary timescales, as reflected by the supremacy of sex on the tree of life [55]. Sex (involving chromosomal recombination via crossing-over) provides populations with new genotypic combinations on which natural selection operates, and facilitates removal of deleterious mutations that otherwise accumulate inexorably in asexual genomes [3]. The theoretical prediction that asexual lineages constitute evolutionary dead-ends is confirmed on phylogenetic trees relating sexual and asexual taxa: asexual taxa typically show a sexual ancestor and occupy terminal nodes of the trees. Only rare asexual taxa, referred to as ancient asexuals and in effect “evolutionary scandals” by Maynard-Smith [56], succeeded in persisting as asexual lineages for millions of years (My). The most famous and documented case are the Bdelloid rotifers which have been reproducing without sex for 35 My, although doubt persists as to the actual antiquity of such asexual lineages [57].
This expectation of low “evolvability” in asexuals also applies to aphids, as now briefly summarized. To begin with, comparing the genetic diversity of “sexual” and asexual lineages in distinct aphid species has allowed quantification of a two third reduction in genotypic variation in clonal relatives in comparison with recombining populations (Table 2). Furthermore, experimental evolution studies and sequence analysis have indicated a rapid acceleration of deleterious mutation accumulation in asexual aphids [58,59]. Lastly, phylogenetic methods involving DNA sequencing, applied to test for long-term asexuality in two putatively ancient asexual taxa (the Tramini tribe and asexual lineages of the aphid R. padi) have revealed signs of recombination in both taxa, suggesting a recent loss of sex in these asexual lineages [60,61].
Comparison of genotypic diversity in recombining and clonal lineages of three aphid species.
Aphid species | Recombining lineages | Clonal lineages | References |
Myzus persicae | 0.97 | 0.27 | Vorburger et al., 2003 [53] |
Rhopalosiphum padi | 1.00 | 0.35 | Delmotte et al., 2003 [60,62] |
Sitobion avenae | 0.97 | 0.28 | Papura et al., 2003 [46] |
8 Asexual aphid lineages arise repeatedly from diverse mechanisms
The presence of coexisting asexual and sexual lineages within species belonging to various aphid genera and tribes is evidence for multiple origins of obligate asexuality in these groups [8,9]. Phylogenetic analyses of sexual and asexual lineages using rapidly evolving markers (e.g. microsatellites, mitochondrial DNA sequences) confirms independent sex loss events below the species level [62,63]. This shows that sexual reproduction is a rather labile genetic system and raises questions on the mechanisms by which asexual lineages originate.
Intensive studies combining ecological, experimental and phylogenetic approaches have demonstrated the existence of at least three not mutually exclusive routes to asexuality in aphids (reviewed in [3]). Firstly, spontaneous loss of sex could occur when the conditions necessary to induce sexual reproduction are not met, as for example in invasive populations colonizing zones where environmental cues are insufficient to elicit the reproductive switch [64]. In this case, any mutation on genes involved in the cascade of events from photoperiod perception to reproductive orientation (see above for candidate genes) would partially or completely alter the sexual function [62]. Alternatively, chromosomal changes, which are very common in aphids, can potentially prevent sexual reproduction by generating a meiotic barrier due mainly to disjunctions on the metaphase plate [64]. Secondly, hybridization between closely related species is a major route to asexuality in animals. This mechanism of asexual lineage generation is also found in aphids, although its importance is unknown [60]. Thirdly, asexual lineages may arise secondarily from pre-existing asexual lineages, formed upon one or both of the above mechanisms, as a result of incomplete reproductive isolation between sexual lineages and their asexual counterparts. New asexual lineages can thus be generated through “contagious” gene flow into the sexual pool. Contagious parthenogenesis has not only been detected in laboratory crosses involving sexual and essentially asexual lineages [65,66] but also in natural populations surveyed with molecular markers and biological assays [67,68]. Micro-organisms such as Wolbachia induce obligate parthenogenesis in many arthropods. Although aphids harbor a large community of bacteria, including some known to manipulate their host reproduction [67], there is no evidence so far for a role of micro-organisms in sex loss in this insect group [69].
The variety of processes involved in transitions to asexuality translates into a wide range of asexual genotypes with different genetic and ecological properties, and deeply affects the outcome of competition between sexual and asexual lineages, both in the short- and the long-term [9].
9 Conclusions
Because of their uniquely evolved reproductive strategies, which explain to a large extent their economical impacts [10], aphids are excellent systems to study reproductive biology in an integrative manner, from molecules involved in alternative pathways of sexual and clonal reproduction to ecological factors that shape their evolution.
The discovery of parthenogenesis in aphids was made by Charles Bonnet (1720–1793), a Swiss naturalist and philosopher, as long ago as 1745 [70]. Yet, despite the great strides made in aphid biology since that time, including in physiological and molecular studies, the exact molecular, cellular and physiological processes implicated in the cascade of mechanisms from the perception of the photoperiodic signal to the production of clonal or sexual forms remain largely unknown. The recent development of genomics and post-genomics has tremendously revitalized the field of aphid reproductive biology that originally prospered some 20–30 years ago. Hypothetical scenarios of reproductive mode regulation by seasonal photoperiodism are available but they now require full validation through functional analysis of candidate genes and effectors. Genome-wide changes in gene expression can also be tracked during the course of reproductive shifts by means of exhaustive micro-arrays and high-throughput sequencing technologies. Coupled with quantitative trait loci (QTL) approaches, these methods are powerful tools in current efforts to identify regulatory networks of polymorphic genes. Their application to aphids will greatly help to test whether reproductive mode variation is determined by a few genes or genome-wide chromosomal interactions and will surely allow exploration of the interplay between phenotypic plasticity and polymorphism at the gene level.
Acknowledgements
We thank J.L. Bonnemain for inviting us to contribute to this special issue on aphids. We are also indebted to many colleagues for stimulating exchanges on evolution of sex theories, in particular B. Bengtsson, F. Delmotte, F. Halkett, H. Loxdale, M. Plantegenest, C. Rispe, P. Sunnucks C. Vorburger and A. Wilson. G. Le Trionnaire is acknowledged for Fig. 2.