1 Introduction
As now widely recognized [1], human activities are exerting on the biosphere an unprecedented impact [2]. The public has been first aware of environmental problems through stories of extinctions of Vertebrate species such as the Dodo Raphus cucullatus [3] and the Passenger pigeon Ectopistes migratorius [4]. Range shrinkage and local extinctions are even more striking: among many examples, the Lion Panthera leo was present in Greece in historical times and in Iraq until the 19th century, disappeared from North Africa during the 20th century, and its African range is becoming increasingly fragmented, mostly because of interactions with livestock [5]; the north American Bison B. bison, whose numbers perhaps once reached 60 million individuals, nearly faced extinction in the 19th century because of excessive killing, and now occupies a restricted and fragmented range [6].
The purpose of this article is to review the impact of human activities on terrestrial Vertebrates viewed as a component of global biodiversity. This text is more aimed at researchers interested in biodiversity than to Vertebrate specialists, and I will review simple questions such as: What is the degree of generality of human impact on Vertebrates? Why and how are Vertebrates affected by human activities? What is the relative impact of the various components of global change? What are the consequences and prospects for ecosystems and human societies?
For disentangling the causes and understanding the mechanisms, I emphasize Vertebrate population dynamics as much as human impacts. The parallel case of marine fish being well known [7,8] (for freshwater fish, see [9]), I restrict my attention to terrestrial Vertebrates, with a bias to birds, the best known group from the demographic point of view. For reasons of space, I will obviously not attempt to be exhaustive and will cite a mix of selected illustrative case studies and review articles.
2 Context and predictions
2.1 Some Vertebrate characteristics
Population dynamics studies strongly emerged over the last 40 years in relation with the development of computing and mathematical models: demographic parameters in animal populations are commonly estimated using marked individuals and “capture-recapture” statistical models [10], while population projections, notably by matrix models [11], make it possible to address a variety of biological questions, from evolutionary mechanisms to quarry species management. Through these developments and because they can easily be captured, marked, followed and counted, terrestrial Vertebrates are the only animal groups for which a reasonably comprehensive view of population dynamics and conservation status is available.
According to IUCN, a respected intergovernmental organization using explicit and formal criteria [12], at least 15% of terrestrial Vertebrates are currently threatened (Table 1), possibly more as non documented cases often concern species with small range and population size, and, as such, possibly threatened. There is also a fairly comprehensive record of species extinctions: on the average, more than one species disappeared each year over the last five centuries, with more than one half of those in the 20th century [13,14]. Indeed, one may calculate that nearly 10% of Vertebrates became extinct in the last few thousand years [15].
An overview of the conservation status of terrestrial Vertebrates based on the IUCN 2008 redlist (http://www.iucn.org). Some figures are approximate as obtained from percentages. Besides these terrestrial Vertebrates, there are about 30,000 fish species.
Category | Taxon | ||||
Amphibians | Reptiles | Birds | Mammals | Total | |
Critically endangered | 475 | 86 | 190 | 188 | 939 |
Endangered | 755 | 134 | 361 | 448 | 1698 |
Vulnerable | 675 | 203 | 671 | 505 | 2054 |
TOTAL Threatened | 1905 | 423 | 1222 | 1141 | 4691 |
TOTAL Not Threatened | 2697 | 962 | 8489 | ∼3457 | 15,605 |
Nr of species assessed and not data deficient | 4727 | 1385 | 9928 | ∼4598 | ∼20,638 |
6347 | 8734 | 9990 | 5488 | 30,559 |
This alarming situation results from a special sensitivity of Vertebrates to any change in their perturbation regime, in particular those induced by human activities. Compared to other animal groups, Vertebrates are characterized by the following series of correlative traits [16]:
- • large size that attracts the hunter;
- • small population size that increases the effect of any absolute harvest;
- • large home range that increases the risks of interaction with human activities and land use;
- • low maximum growth rate that reduces maximum sustainable mortality [17];
- • relatively complex social behavior (as in large primates or Elephants, but also in colonial seabirds) and inbreeding (see e.g. [18]) induce threshold effects, below which populations unavoidably enter an “extinction vortex”, i.e. are rapidly doomed to extinction [19].
Associations between these traits make large species especially vulnerable [20]. For instance, large, attractive and long-lived parrots are more threatened than smaller species (Fig. 1, based on data in [21]). Among these correlated traits, the low maximum population growth rate (MPGR) plays a key role as it determines the maximum sustainable increase in mortality [22], exactly as the interest rate on a capital determines the maximum sustainable tax rate. MPGR is closely associated with longevity, as a result of the spread of reproductive effort over life [17]. Based on 119 bird species, Desprez [23] shows indeed that the conservation status deteriorates with generation time, in a genuine “malediction of long-lived species” [10].
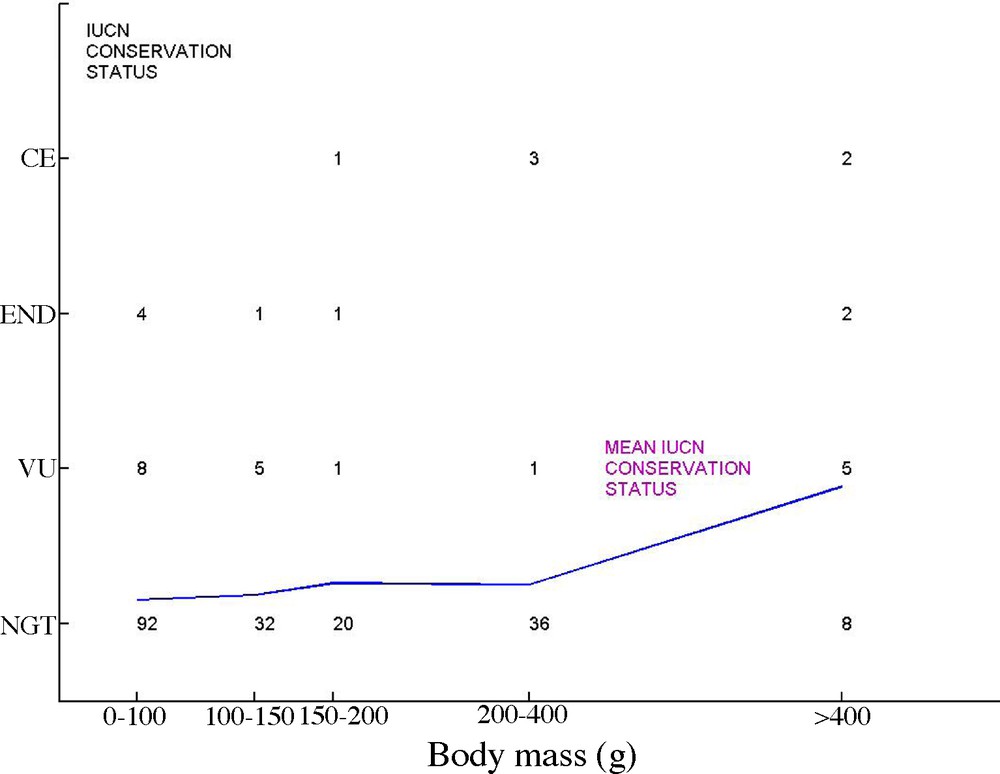
IUCN conservation status of the 222 Parrots (Psitttadae) species of the world, according to body mass (from data in [21]). For each status (NGT: Not Globally Threatened, VU: Vulnerable, END: Endangered, CE: Critically Endangered), and each body mass class (0–100 g, 100–150 g, 150–200 g, 200–400 g, > 400 g), the number of species is reported on the graphic. The line is a mean conservation status index (based on NGT = 0, VU = 1, END = 2, CE = 3) that clearly indicates larger species have more threatened than smaller ones.
Many other aspects may increase the sensitivity to perturbation. An outstanding case is that of island species, which have often evolved in the absence or scarcity of pathogens and predators, many bird species having become even flightless: for an island bird, the relative risk of being threatened species is 40 times higher than for a mainland one [24], the Dodo being but an example of hundreds of extinctions [25].
2.2 Global change
The last element of context concerns the components of global change, too often reduced to climate change. Briefly, environmental changes at the planetary level can be ranked in three main types:
- • exploitation (such as hunting) including incidental exploitation such as the bycatch of seabirds by fishing gear;
- • changes in land use (agriculture, urbanization, tourism…) and general practices, such as the development of transport; such changes are extremely diverse, emerged long ago, accelerated with the industrial revolution and have been rapidly increasing and diversifying up to day;
- • climatic change, for which IPCC scenarios [26] can be used to evaluate expected changes in species range and extinction risks; we still are in the first phase of this change.
It should be noted that all components of global change result from or at least are exacerbated by the increase in human populations.
2.3 Expectations
In this context, one can draw the very simple following expectations:
- • exploitation should have been the dominant source of impact up-to-now or until recently;
- • it may have been superseded by changes in use;
- • climate change may have started showing limited effects;
- • species sensitive to perturbation, in particular long-lived species and “Megafauna” [14], should be the most strongly impacted, i.e. human impact should not be uniformly distributed over all Vertebrates.
3 Direct and incidental exploitation
Among extinctions in the historical period, many are indeed attributable to exploitation. One may cite besides the Dodo and the Passenger Pigeon the case of the Great Auk Pinguinus impennis, the only species among Alcids, which are northern hemisphere seabirds, to have been flightless, as are the unrelated southern hemisphere Penguins (Order Sphenisciformes). The Passenger Pigeon case draws special attention since it was present by the million in pre-Columbian times. However, it was the only temperate Columbid to lay a single egg per year [4], inducing most likely a low MPGR.
Earlier on, the end of the Pleistocene was characterized by massive extinction events, notably that of large mammals. Exploitation by Man seems to have been the dominant factor worldwide [27] in combination with impacts on habitat and climate change [14,28]: models accounting for the particular demography of these large-sized species confirm exploitation was sufficient to be unsustainable [29], and the relatively small changes at the community level do not point to a dominant role of environmental change [27]. More recently, Moas, giant flightless birds related to extant Kiwis, disappeared from New Zealand rapidly after the colonization by Polynesians, both through hunting and habitat destruction [30], while other species disappeared from New Zealand at that time through the impact of introduced rats [31].
A comprehensive review of the impacts of hunting on quarry species would require a paper of its own. For instance, several million ducks are harvested every year both in North America and Europe [32]. Restrictions aiming at sustainability, such as the application to waterfowl hunting of European regulations taking account of the life-cycle of migratory species, are often the subject of sterile debates, while the example of the Roe Deer C. capreolus and other ungulates in France shows that appropriate regulations can induce both sustainability and in the long term and an increase in the hunting bag [33]. The impact of side effects of hunting such as “crippling loss” (unrecorded deaths of wounded animals, generally estimated to 20–40% [34]) and disturbance [35] remains difficult to estimate. Poaching remains a problem in particular for the tropical mammalian megafauna [36,37]: in interaction with habitat loss, exploitation is indeed the major concern for Asian mammals [38].
Often, exploitation is incidental, i.e. animals are killed by some harvesting gear without being the target. The bycatch of seabirds, in particular Albatross Diomedea and Phoebastria spp., by longline fisheries in the Pacific and Indian Oceans is particularly well documented. The estimated increases in mortality match decreases in population size [39], and have been shown to be directly related to the intensity of the fishing activity [40]. This impact concerns widespread species as well as endemic or near-endemic species restricted to secluded archipelagos [40,41] and constitutes one of the most striking examples of the universal spread of human impact on Vertebrates. Despite the low catch rate, typically around 1 per 50,000 hooks set, Albatross cannot stand the bycatch, because of the combination of several million hooks at sea, the wide dispersal of Albatross, and their extreme demographic sensitivity, with a maximum growth rate not exceeding 4% per year in the largest biennially breeding species. A variety of other mechanisms interact [42]. For instance, a bias of capture towards one sex [41] increases the impact at the population level as Albatross are strictly monogamous with a lifelong pair bond. Offal availability may induce a benefit for seabirds [43] that might partly compensate for the bycatch [40] but this potential mechanism still remains to be investigated. As well as seabirds, Sea Turtles, Porpoises, Dolphins etc. are submitted to a heavy bycatch by a variety of fishing gear [44].
Thus, as a conclusion to this section, exploitation started long ago and still has a major impact on Vertebrates, with, e.g., one third of threatened bird species being so by exploitation with a bias towards large species with long generation time [45] and, correlatively, a low MPGR.
4 Changes in use
I use here “changes in use” as an omnibus category covering all changes in land use and life style that have been characterizing human expansion and socioeconomic development over centuries. Currently, Man diverts for its own use about 50% of the Earth's primary production [46], depleting thus a large part of the natural resources of animal life. The acceleration in changes in use since World War 2 is obvious, but some aspects are ancient. For instance, aborigine colonization of Pacific islands and later the development of maritime transport led to the introduction of rats, predators and pathogens, the latter often through the introduction of exotic Vertebrates, to nearly all islands throughout the world [47]. These introduced animals became the main sources of Vertebrate extinctions on islands, and their impact can be viewed as a part of “biological invasions”, which are treated here for the sake of simplicity as a consequence of transport by man rather than as a specific subject. The impact of introduced pathogens on the avifauna of the Hawaii archipelago is well understood [48] and is not an isolated case: island birds have largely been threatened and decimated through introductions of other birds, either through competition or imported disease [49–51]. At the level of continents, among 2372 terrestrial Vertebrates and freshwater fish, only 33 have been successfully introduced from Europe to North America or vice versa [52]. An analysis of the characteristics of successfully introduced species [53] did not find any effect of size, affiliation with man being the main correlate of successful establishment. However, this study did not consider demographic traits, nor correction for phylogenetical dependence [54]. In contrast, among more than 30 bird species successfully introduced to New Zealand, most were Passerines [50] and successful invaders had significantly higher fecundity/mortality ratios [49], i.e. high MPGR. It seems thus clear that some selectivity exists, small seed-eating birds being for instance more susceptible to be introduced and spread than a vulture or an eagle. Cases such as the successful introduction of the Starling Sturnus vulgaris to North America are common, and indicate a tendency for small size, high growth rate species with some affiliation to Man to successfully invade.
In parallel, human settlement developed a variety of land uses, in particular agriculture, and more recently, urbanization, industries and transport infrastructures that destroyed the habitat of many specialized species. Again large species, with greater space requirements and special sensitivity, paid the highest toll. The degradation of old coniferous forest in the western US has impacted the Spotted owl Strix o.occidentalis, through a well documented series of degradation of demographic performance [55,56]. Habitat degradation is indeed usually cited as the main cause of threats to animal life, Vertebrates in particular [57]. The current agricultural intensification in western countries strongly impacts farmland birds, through a variety of mechanisms including changes in food availability and foraging behavior [58], with a stronger impact on specialist than on generalist species [59]. Farming or livestock breeding has often implied a steady and tenacious destruction of large predators, which I cited as a case of exploitation, but directly results from intensification of land use. On can cite the Cheetah Acynonix jubatus [60] or the extreme case of the Tiger Panthera tigris [61], but the debate in France about the Wolf Canis lupus and the Brown bear Ursus arctos [62] clearly shows it is a global problem. The impact of changes in use is not restricted to birds and mammals as strong declines in Amphibian and Reptile populations have been widely observed, although the relative roles of changes in land use and in climate are discussed [63], and the proportion of species whose conservation status is documented is the lowest among Vertebrates (Table 1). The development of agriculture and the intensification under way worldwide are inducing a huge amount of local extinctions of Vertebrates, for which hundred of examples could be cited. They concern not only large emblematic species, such as, e.g., the Little Bustard T. tetrax whose number of breeding males decreased by 92% in France over the last two decades [64], but also common generalist species such as the House sparrow Passer domesticus [65].
As a conclusion to this section, the combination of local extinctions, mostly of specialists, combined with successful introductions or spread of exotic generalist species is but the translation for Vertebrates of a global tendency to a simultaneous impoverishment and spatial homogenization of biodiversity [66,67] in which changes in use play a major role. It is not the abundance of a single emblematic species that is modified, but whole communities. If exploitation has had a dominant impact on Vertebrates until recently, and still has a major one, it is thus clear that habitat degradation and, in a general fashion, changes in use are currently a dominant cause, in developed as well as in developing countries.
5 Climate change
The first step in investigating a potential impact of climate change on Vertebrate population dynamics consists of linking a demographic trait such as survival probability to climatic covariates. Among forerunners of such studies, [68] showed that adult survival in the Alsace population of the White Stork C. ciconia was, during a period of rapid decrease, strongly linked to rain in the Sahel zone, where most Alsace storks overwinter. Many papers followed with the development of long-term animal population research programs [69] and capture-recapture methodology [70]. Several biases may affect such studies: publication bias is probably low as studies of demographic parameters are of particular interest and easy to publish, even in the absence of a relationship with climatic covariate [71]. Bias in the selection of covariates has been examined thoroughly by Grosbois et al. [72]. Among 78 studies examined, they conclude that many alleged relationships did not appropriately account for the number of covariates tested or for trends, and that relatively few studies provided unequivocal evidence of links between vertebrate demography to climatic characteristics.
The largest number of examples concerns seabirds and large-scale oceanic weather patterns, in the South and North Pacific as well as in the Atlantic [73–76]. The paper by Lee et al. [75] is one among several examples in which several parameters vary with a same climatic covariate, inducing stronger population changes than when a single parameter is concerned. The repeated evidence is that of a detrimental effect of climate change on seabird populations, largely through trophic effects. For instance, tropical seabirds are affected by subtle trophic changes several months before El Niño occurrences [77]. The tuning of the populations to resource availability, e.g. through foraging distances and time budget is reviewed by [78]. While some species may react by changing their foraging distribution [79], it cannot be the case in extreme environments with examples of species commuting with extremely tight time and energy budget from breeding grounds to distant oceanic fronts that are literally moving away [80]. This is well documented for The King Penguin Aptenodytes patagonicus [81], other Penguins [82] and Elephant seals Mirounga leonina [83]. Deterioration of habitat through climate change and demographic impacts has also been demonstrated in the Polar bear Ursus maritimus [84]. For the Polar Bear [85] and the Emperor Penguin Aptenodytes forsteri [86], model projected populations are decreasing.
There is thus fair evidence that many seabird species and Vertebrates in circumpolar environments are strongly influenced by large-scale oceanic conditions, themselves driven by climate. As a consequence, the concerns on changes in ENSO and NAO with climatic change [87] directly translate into concerns for Vertebrates, in particular seabirds and marine mammals.
Similar results have been obtained for terrestrial animals, and a full review of relationships between Vertebrate demography and climate is beyond reach. For instance, [88] showed survival in a North American Passerine wintering in the tropics was linked to the Southern Oscillation Index. While Reindeer and Caribou are affected by a general decrease [89], through a variety of changes in their habitat as a result of warming [90], arctic breeding geese, whose populations have been increasing over the last decades, should go on growing with increased availability of suitable breeding habitat [91]. For sedentary bird species, although large-scale climatic integrative variables are commonly used as a proxy, local climate summaries, e.g. obtained by Principal Component Analysis, are logically better predictors, as distant climate can only be linked to a demographic trait through its correlation with the climate in the range of the population under study [92]. Indeed, this is also true for a coastal seabird, the blue-footed Booby [93]. Several studies investigate the effect of extreme events [94] for which further results are expected with the increasing length in time of many long-term programs. Phenological changes, in migrating dates or patterns, and breeding dates have also been observed [95]. Further examples of the relationship between climate and Vertebrate demography or populations are summarized in [96], p. 103.
An expected consequence of climate change is a shift towards the pole and higher elevations of the potential range of species, as well shown by “niche models” [97], while in parallel changes in drought regime will tend to decrease the abundance of many species [98,99]. The response to climate change will result from the sensitivity to temperature (for homeotherms, see [100]) and by the attachment to a more or less specialized environment, in particular dominant vegetation. The magnitude of the shift should match in the long run the temperature shift, but this match should occur with some delay, for various reasons: limited dispersal, colonization limited by social behavior, site tenacity even after perturbations [101], mismatch with vegetation belts which move very slowly, concomitant change in habitats with land abandonment, etc. For reptiles, range expansion northwards is expected both in Europe [63] and North America [102], but will certainly be considerably slowed down, or even prevented by the extreme fragmentation of habitats and distributions of many species, in a clear case of interaction between climate change and changes in land use.
Following a few meta-analyses confirming the evidence for an effect of climate change [103,104] on species ranges, I tentatively assembled in Table 2 a few published results on Plants and Butterflies compared with five examples of tests of shift in elevation and latitude of bird communities. Observed changes, converted to °C using rough conversion coefficients and based on a very general proxy (0.2 °C per decade) do increase with the expected change. The heterogeneity of responses results in a slope not significantly differing from 0 (t = 0.487, P = 0.32, one-tailed test) but several of the individual studies showed significant shifts. Bird data show a lower response than butterflies (ANCOVA, t = 2.356, P = 0.051, two-tailed test–see Fig. 2). As expected [105,106] the response, close to 9.4 km (in latitude) per decade seems incomplete (0.2 °C corresponds to 33 km per decade) but is compatible with the most robust overall estimate currently available, 6.1 km per decade [104]. Many biases affect these results: species and communities are mixed, the rough conversion factor used may be biased, this conversion factor is at any rate a proxy inducing thus an “error-in-variable” problem and a bias of the slope towards 0 [107]. It is thus certainly too early for a comprehensive and deep meta-analysis focusing on latitude and elevation shifts among Vertebrates: the evidence for a slower response than in Butterflies has to be confirmed.
A few examples of shifts in elevation and latitude over the last decades for various living groups. “Level” is C for community data (multiple species) and S for single species data. Some of the data were back-converted to ensure uniform units of change. The conversion are based on 100 m in elevation = 100 km in latitude = 0.6° C change, and an observed change of 0.2 °C per decade [26].
Taxonomic group | Level | Nr of years | Elevation (m) | Distance (km) | Equivalent °C (0.6/100m or 100km) | Expected °C (0.20°/10 years 1960→2010) | Reference |
Butterflies | C | 34 | 212 | – | 1.272 | 0.680 | Various authors in [143] |
Butterflies | S | 92 | 0.552 | 0.630a | |||
Butterflies | S | 73 | 0.438 | 0.528a | |||
Butterflies | S | 80 | 0.480 | 0.528a | |||
Butterflies | S | 149 | 0.894 | 0.588a | |||
Butterflies | C | 42 | 67 | – | 0.402 | 0.840 | [144] |
Plants | C | 10 | 29 | – | 0.174 | 0.200 | [108] |
Birds | C | 11 | 29 | – | 0.174 | 0.220 | [105] |
Birds | C | 17 | – | 91 | 0.506b | 0.357c | [106] |
Birds | C | 20 | 3.58 | 0.022 | 0.400 | [145] | |
Birds | C | 35 | −2.4 | – | −0.014 | 0.700 | [146] |
a Based on the cited elevation or latitude expected shifts.
b The authors use 0.556 °C/100 km.
c The authors use 0.068 °C per year in France in Spring for conversion, and get 1.156 °C.
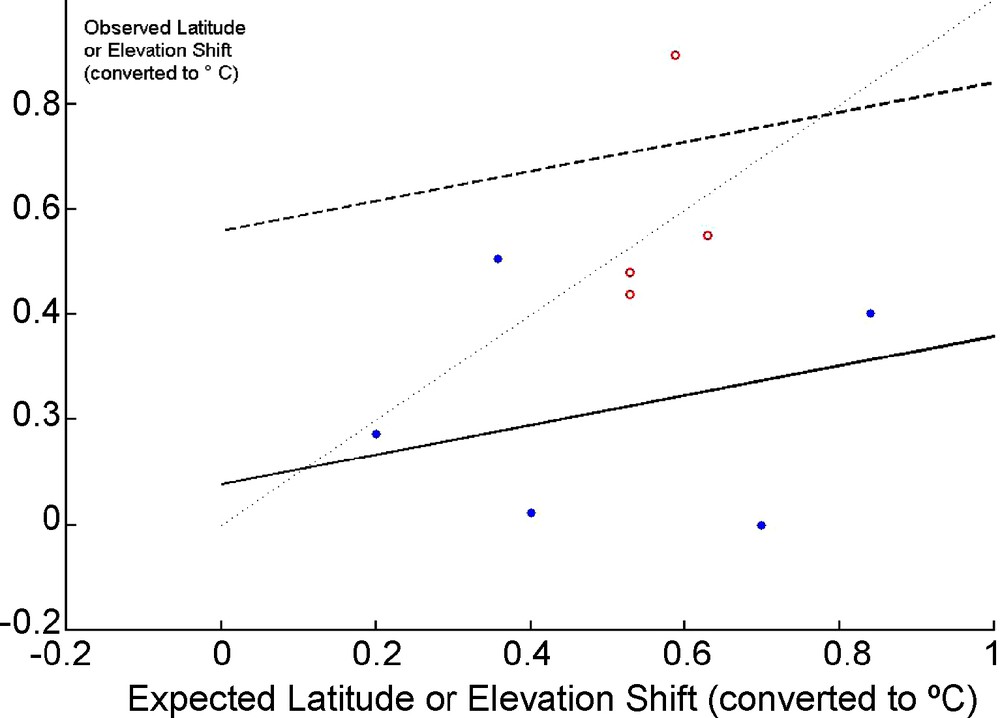
Observed versus Expected Elevation or Latitude Shifts (converted to equivalent° C°) for the data in Table 1. Open circles and dotted line: butterflies, filled circles and plain line: birds. Thin dotted line: Y = X. The lines for butterflies and birds are based on an ANCOVA (two regression lines with a common slope and different intercept).
Lenoir et al. [108] show a faster elevation change for those alpine plant species with a shorter life-cycle, a finding that would be interesting to test among Vertebrates.
Finally, for what concerns elevation shifts, some Vertebrate species, exactly as alpine plants [109] could become more and more isolated on mountain tops, in Alpine tundra habitat islands, as already happened since the last glacial period [110]. Thermal stress indeed explains local extinctions and rapid elevation shifts in a mountain Lagomorph, the Pika Ochotona princeps [111]. More generally, for plant and butterflies, species with restricted distributions were shown to be particularly sensitive to climate change [112].
Besides this wide evidence of the already sizeable influence of climate on vertebrate populations, several papers emphasizes complex interactions, such as between incidental exploitation and climate change for seabirds [113], changes in land use and climate for all Vertebrates [63,114,115].
6 Discussion
6.1 Overview
The situation of Vertebrates in face of anthropogenic impacts is characterized by two types of dissymmetry.
6.1.1 Dynamics of populations
First, concerning the dynamics of populations, while sharp population declines can happen rapidly (such as the kill of more than 50% of the Elephants Loxodonta africana in Zakouma National Park during the recent troubles in Chad, [37]), growing back to earlier population size takes much more time or may just never happen because of the low MPGR rate of Vertebrates, in particular long-lived ones. In technical terms, human activities increase the perturbation rate of such populations, up to a level to which they are not adapted, and induce a slow erosion of population sizes. Full evolutionary compensation remains the exception [51] and will be just impossible for long-lived species [86], although one may expect some selection for a faster demography and higher MPGR.
6.1.2 Increase in global extinction rates
Second, the resulting increase in global extinction rates by several ten-fold [116,117] cannot be compensated by an increase in speciation rate. Although the fragmentation of habitats, by isolating local populations, may in the long run favor the evolution of local adaptation, and in turn speciation [118] such effects require thousands or even million years [119] and will be unavoidably superseded by the near immediate acceleration in local extinctions, small isolated subpopulations often being non viable. The current decrease, fragmentation and extinction of Vertebrates will indeed modify and impair their evolution to come for several million years.
Although there is an overall increase in extinction rates of all life forms [116], the selectivity of the extinction towards long-lived species is strong and unavoidable. This selectivity may not be a specific characteristic of mass extinctions (contra [120]), but just an automatic correlate of any increase in extinction rate. The survival of many small animals and the extinction of Dinosaurs at the K-T transition [121] fit this scheme.
The current general decrease of many Vertebrate populations, in particular specialists, together with the spread of habitat loss and fragmentation, and the explosion of local extinctions may be but the first signs of an accelerated crisis, just as the thinning of glaciers is a poorly visible sign that precedes major retreats. A recent review of conservation statuses indeed confirms our analysis of the mechanisms impacting Vertebrate populations and shows a continuing deterioration worldwide [122]. The only pause might come after the extinctions of the most sensitive species, Megafauna and islands species in particular.
6.2 Consequences
The consequences of the impact of human activities on Vertebrates are diverse and still not fully evaluated, in particular because they are less easily amenable to experimental approaches than those linked with smaller forms of life. They tend to be underrated as, apart the case of fishery resources as most studies on ecosystem services concentrate on key resources such as water or core functioning such as soil microbial biodiversity [123] (see, however, [124]). In this context, given the poor resilience of Vertebrate populations, the option value for future generations of any choice is obviously very high.
Following the Millenium Ecosystem Assessment [1], ecosystem services are usually classified in three main types [125]: provisioning services, regulating services and cultural services (supporting services being supposed to result from these three types).
A large part of Vertebrate biodiversity is linked to cultural services and among them to recreation and aesthetic values: thus, one cannot follow Carpenter et al. [125] who indicate an absence of trends for such services. The patrimonial value of large Vertebrates is universally recognized, often considered as distinct from services resulting from “ordinary biodiversity” [126], and as shown in this review, clearly threatened to an unprecedented level.
The impact of the Vertebrate crisis on provisioning services is obvious for fisheries [7] including freshwater ones [9,127]. It also concerns genetic resources. Even if one should not expect much new domestication of Vertebrates, an extended use of Vertebrates as a resource, such as in wildlife ranching, is a key possibility from developing countries, although the results are currently ambiguous [128]. Hunting and Ecotourism fortunately induce a cultural and economic incentive for specific habitat conservation [129].
Finally, the disruption in ecosystems brought by local or global vertebrate extinctions [130] will affect a number of “regulating services” [125], and deserves deeper understanding through the development of trophic ecology and ecology of interactions, including their fundamental and theoretical aspects [131]. Besides the regulation of insect pests by insectivorous birds [132], a striking example is zoochory, that takes a particular importance with the expected vegetation belt shift towards the poles. The current gradient (0.2 °C per decade ∼3.3 km per year) is already well beyond that prevailing at the end of the late glacial period. For trees with a long generation time, the vegetation–climate balance may be conserved only with propagules being moved several km, with a key role of Corvids [133].
6.3 What to do?
Some of the research needs were emphasized earlier. I will only briefly mention the need for improved knowledge, in particular through improved monitoring [134], modeling [97] and comparative [54] approaches.
In the current Vertebrate crisis, one should clearly reaffirm the need for improved species protection. It is notable that a large part of the species conservation effort is done by NGO and conservation associations (for an example of successful reintroduction, [135]) which should be acknowledged for their energy and efficiency. For what concerns habitat conservation, Megafauna survival is a matter of scale. Few countries would accept as South Africa does, to protect a piece of land such as the Kruger National Park (350 km × 60 km, about 1/10th of Great Britain surface). Given the huge economical needs around, any successful attempt at eradicating the tsetse fly (which currently hampers the development of livestock breeding) would unavoidably imply a reduction in the size of African National Parks. A form of compensation is probably a solution in many cases to escape the “tragedy of the commons” [136]: some western countries decided to give money to Ecuador [137] in exchange of the abandonment of gas exploitation in a wide area of the Napo basin, which shelters one of the highest Vertebrate biodiversity in the world with 550 bird species per square kilometer, as many as in the whole of Europe. Implicitly, the compensation approach gives an economic value to biodiversity, even in the absence of direct identified services. A number of other approaches (ecological corridors for reconnecting habitat patches, prey-predator management [138], mitigation measures of various kinds [139]) open the way to an enlarged ecological engineering that would feed on the ecological side the ecology–socioeconomy integration currently under way [140].
Given their relatively secondary role in ecological mechanisms and ecosystem services, the erosion of Vertebrate biodiversity will not be the major incentive for changes in socioeconomic systems. Given the level of threats to which Vertebrates are currently submitted and their specific sensitivity, unless they are accepted as sentinels of the Man impact on the biosphere [141,142], the crisis of Vertebrates will not slow down.
Acknowledgments
I thank P.A. Crochet for having brought to my attention a few references, and M. Schaub for critical reading and helpful suggestions.