1 Introduction
Pluripotency of stem cells is important for cell replacement therapy. Embryonic stem cells (ESCs) exhibit pluripotency [1,2], but because of practical issues, they are problematic for human cell replacement therapy. This led laboratories to induce pluripotency in normal adult somatic cells such as skin fibroblasts (iPSCs) [3,4]. However, the induction of pluripotency may evoke unknown genetic changes in the cells and cause tumorigenicity [4]. Therefore, we investigated whether there are natural adult stem cells that express ESC genes and share pluripotency with ESCs. Indeed, we find that a subpopulation of stem cells from adult bone marrow naturally expresses an array of ESC genes including Oct-4, Rex-1, Sox-2, Klf-4 and LIN-28–genes that have been used to induce pluripotent stem cells from adult fibroblasts.
Pluripotency means that the stem cells have the potential to differentiate into a variety of different lineages. We examined, in this subset of bone marrow stem cells, the expression of lineage genes for each of the three embryonic germ layers. We previously reported that these bone marrow stem cells express an array of ectodermal neural genes of both neural progenitors and differentiated neurons and oligodendrocytes [5]. Here, we find that the same subset of adult stem cells expresses mesodermal cardiac lineage genes and endodermal pancreatic lineage genes as well as intestinal genes. Taken together, the results shown here indicate that the expression of ESC genes does not preclude the expression of terminal differentiated cell genes of each of the three germ layers and that these cells may represent ESC-like cells in the adult.
2 Results
2.1 Mouse hematopoietic progenitors express embryonic stem cell genes
Adult bone marrow transplants have been performed for 50 years for stem cell replacement therapy, which gives rise to all hematopoietic lineages. We asked: do these bone marrow stem cells have greater pluripotency and moreover, do they express ESC genes? We reported earlier that there is a subset of CD34+ cells in ex vivo bone marrow that expresses ESC genes Oct-4 and Rex-1 and we developed a culture method to grow a pure population of this subset of stem cells [5]. Here we report that these same ex vivo stem cells and in vitro stem cell cultures also express ESC genes Sox-2, Klf-4 and LIN-28 (Fig. 1). Oct-4, Sox-2 and Klf-4 are ESC genes used by others to induce pluripotency in adult skin fibroblasts [3,4]. Here, in this subset of CD34+ adult bone marrow stem cells, these genes are expressed naturally without induction.
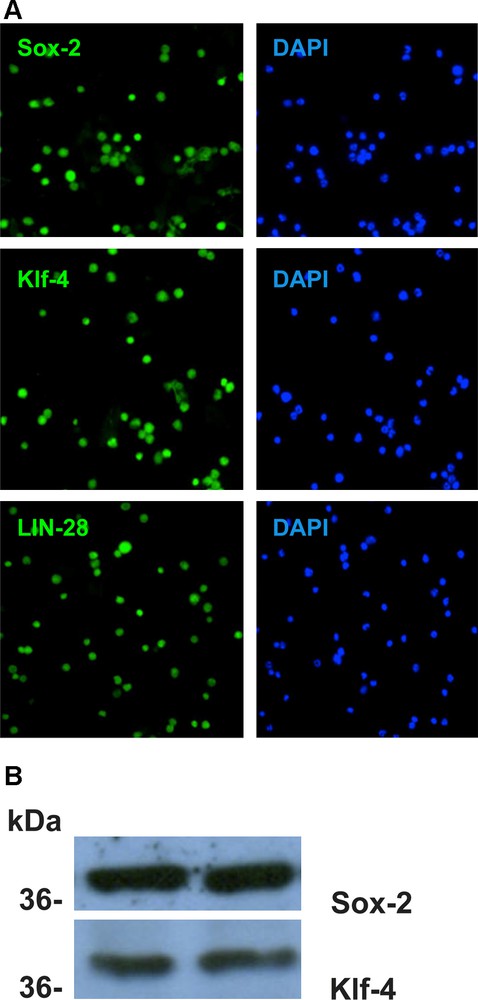
Embryonic stem cell genes Sox-2, Klf-4 and LIN-28 are expressed in all non-adherent CD34+ cells cultured from adult bone marrow as reported in M&M.
A. Immunocytochemistry. Cells were suspended in 4% paraformaldehyde fixative for 15 min. at 4 ̊C, washed 3 times in PBS and then deposited on microscope slides by cytospin and blocked with PBS containing 0.025% Tween-20 for 30 min. at RT. Cells were incubated for from 2 hours to overnight in primary antibody in Block buffer at RT, then washed with PBS 3 times quickly and three 5 min washes. The primary antibodies used were: anti-Sox-2 (Santa Cruz sc-20088), anti-Klf-4 (R&D Systems AF3158) and anti-LIN-28 (Santa Cruz sc-67266). The secondary antibodies FITC-anti-rabbit IgG (KPL202-1516) or FITC-anti-goat IgG (KPL 02-13-06) were added in Block buffer for 2 hrs. at RT and washed as above. The cells were mounted in Vectashield containing the nuclear dye DAPI, the coverslip sealed and cells were examined by flurescence microscopy. All cells with DAPI+ nuclei were labeled by each antibody.
B. Western blot analysis of the non-adherent CD34+ cells in culture with antibodies Sox-2 and Klf-4. Protein expression was determined on 4-12% polyacrylamide gradient gells (Invitrogen) by standard methodology (5,20). Duplicate lanes are shown for each protein. The MW standard positions (kDa) are shown on the left. The gels were run in triplicate. Both antibodies were strongly reactive.
The expression of ESC genes by this subset of CD34+ stem cells suggested pluripotency and therefore led us to look at lineage gene expression for each of the three embryonic germ layers: ectoderm, mesoderm and endoderm. We previously reported that these CD34+ cells express both the neural progenitor gene Pax-6 and differentiated genes of neurons, e.g. neurofilament and NeuN and of oligodendrocytes MOSP and CNPase [5]. Here we examined mesodermal and endodermal lineage genes.
2.2 Mouse hematopoietic progenitors express mesodermal lineage genes
These adult bone marrow stem cells express mesodermal hematopoietic stem cell genes (CD34+, Sca-1+, AA4.1+, cKit+, CD45+, GATA-2+, LMO-2+, Flk-2- and Lin-) but not the differentiated hematopoietic lineage genes macrophage F4/80, endothelial Factor 8, B-cell CD19 and T-cell CD4 and CD8 [5]. Therefore, another mesodermal lineage, the cardiac lineage, was examined. These bone marrow stem cells express both cardiac lineage transcription factor MEF-2 as well as differentiated cardiac genes troponin C and myosin light chain MLC-2 (Fig. 2). Thus, these CD34+ cells express mesodermal genes of both hematopoietic and cardiac progenitors.
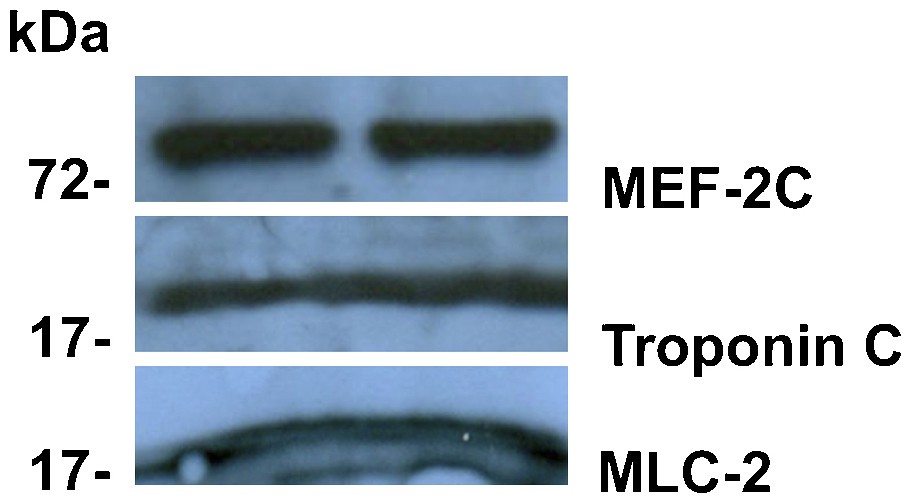
Mouse hematopoietic progenitors express mesodermal lineage cardiac genes. The non-adherent CD34+ cells in culture were analyzed by Western blot with antibodies to the cardiac lineage transcription factor MEF-2 C (Santa Cruz sc-13266) and the differentiated cardiac genes troponin C (Santa Cruz sc-7672) and myosin light chain MLC-2 (Santa Cruz sc-48414). The procedure used was identical to that used in Fig. 1. The MW standard positions (kDa) are shown on the left. The three proteins are expressed at a high level.
2.3 Mouse hematopoietic progenitors express endodermal lineage genes
We then moved to examining endodermal lineage gene expression. Co-expression of CD34 with antigens specific for the pancreatic lineage was first investigated ex vivo by immunocytochemistry of bone marrow cells The first pancreatic gene explored was insulin. Insulin was not detected ex vivo in CD34+ bone marrow stem cells. Consequently, genes expressed in earlier stages of endodermal/pancreatic development were investigated. We found that about one third of ex vivo CD34+ cells could be labeled with antibodies specific for three pancreatic endoderm lineage genes Pdx1, Ptf1a (Fig. 3) and NGN-3 (not shown). To our knowledge, this is the first report that bone marrow expresses genes of the endodermal/pancreatic lineage without induction. In addition, double-labeling experiments showed co-immunolabeling for these three proteins in the same cell (not shown). Furthermore, very few cells, if any, other than CD34+ cells were labeled with anti-pancreatic lineage antibodies.

Mouse hematopoietic progenitors express endodermal lineage genes.
A. Ex vivo expression of pancreatic lineage genes: in situ bone marrow cells were removed onto microscope slides and treated immediately with 4% paraformaldehyde at 4 ̊C. The slides were processed as in Fig. 1 with antibodies to pancreatic lineage genes Pdx-1 (Chemicon AB3243) and Ptf-1a (R&D Systems AF6119). The 2̊ antibody was TRITC-anti rabbit (KPL 03-15-06). A few nucleated cells are immunoreactive to these antibodies.
B. Expression of pancreatic lineage genes by the non-adherent CD34+ cells in culture. Cultured CD34+ cells were processed for immunocytochemistry as in Fig. 1. The 1̊ antibodies used were anti-Pdx-1 (Chemicon AB3243), anti-Ptf1a (R&D Systems AF6119), Anti-CD34 (Pharmingen 553731), anti-Pax-6 (Santa Cruz sc-11357), anti-Neurogenin-3 (Santa Cruz sc-13793), anti-Actin (Chemicon MAB1501R), anti-Sox-2 (Santa Cruz sc-20088), anti-Klf-4 (R&D Systems AF3158) and anti-LIN-28 (Santa Cruz sc-67266). The 2̊ antibodies were TRITC-anti rabbit (KPL 03-15-06) TRITC-anti-mouse (KPL 03-18-06) and FITC-anti-mouse (KPL 202-1806). All cells were labeled with the antibodies to the pancreatic lineage genes, as well as to antibodies toCD34, and actin.
C. Expression of pancreatic lineage genes in cultured non-adherent CD34+ cells by Western blot analysis. Western blots were carried out as in Fig. 1. The MW standard positions (kDa) are shown on the left. The antibodies to pancreatic lineage genes were strongly reactive.
D. Expression of pancreatic lineage gene mRNA s by the non-adherent CD34+ cells in culture. RT-PCR was carried out on cultures of non-adherent CD34+ cells. C3H-1 and C57Bl/6-1 cells had been in culture 6 weeks and C3H-2 and C57Bl/6-2 cells for 12 weeks at the time of PCR analysis. CD34+ cells (and positive control Min6 cells) were washed with PBS then suspended in lysis buffer (RNeasy kit, Quiagen) and RNA was purified on a column following the kit protocol. RNA was quantitated by nanodrop at an absorbance of 260 nm (one unit of OD at 260 nm = 40 gm of RNA/ml) and RNA purity estimated by the absorbance ratio between 260/280 nm. A sample of RNA was loaded on a 1.5% agarose gel in TBE containing BET. RNA then was stored at -80 ̊C. PCR forward and reverse primers were:
18S:5′AGTCCCTGCCCTTTGTACACA3′, 5′GATCCGAGGGCCTCACTAAAC3′;
Pdx-1:5′TTCGCGGGCACCTAAGC3′, 5′GGTTTACCCTGGAGCCATCA3′;
Nkx-2.2:5′AAAGCGCAGACCTACGAGCTG3′, 5′CGGCGTGAGACGGATGAG3′;
Pax-6:5′CCACCCATGCCCAGCTT3′, 5′AACTGACACTCCAGGTGAAATGAG3′;
CXCR4:5′GAAGTGGGGTCTGGAGACTAT3′, 5′TTGCCGACTATGCCAGTCAAG3′;
Sox-17:5′GATGCGGGATACGCCAGTG3′, 5′CCACCACCTCGCCTTTCAC3′;
Hnf-6:5′GGCAACGTGAGCGGTAGTTT3′, 5′TTGCTGGGAGTTGTGAATGCT3′;
Hnf1B:5′GACCTGACCGAGTTGCCTAAT3′, 5′CCGGCTCTTTCAGAATGGGT3′;
Ngn-3:5′AGTTGGCACTCAGCAAAAAGC3′, 5′GATTGCGCTCCCGATCAT3′;
Ptf1a:5′AATTGCCACCGATCACTCACA3′, 5′GGCCCGGAAGGACGAA3′;
Hnf4A:5′CCGGGCTGGCATGAAG3′, 5′GACCTCCGCGTGCTGATC3′; FoxA2:5′TAGCGGAGGCAAGAAGACC3′, 5′CTTAGGCCACCTCGCTTGT3′.
cDNA synthesis was performed using SuperScript III Reverse Transcriptase (Invitrogen). Real-time PCR was carried out in a Biometra Thermocycler: cDNA denaturation stage at 65 °C for 5 min. with random primers. PCR included 40 cycles of a first step 96 °C for 30 sec.; second step 55 °C for 30 sec.; last step 72 °C for 40 sec. Primer products were separated on a 1.0% agarose gel-containing BET. All genes, characteristic of each developmental stage, were expressed in the 6-week cultures (except Pax-6 in C57Bl/6) and most of them in 12-week cultures.
E. Semi-quantitative PCR of pancreatic lineage gene expression in cultures of CD34+ cells. C3H-1, C57Bl/6-1, C3H-2 and C57Bl/6-2 cell cultures were analyzed by semi-quantitative PCR. For semi-quantitative PCR analyses 18S ribosomal RNA was used as an internal control and cDNA primers listed above were used. PCR was carried out in a 96 well plate with IQSyBr Green Supermix (BioRad). PCR was carried out in a MyiQ thermocycler (BioRad). cDNA was denatured at 95 °C for 8.5 min. then PCR proceeded for 40 cycles: 1 min at 95 °C, 30 sec. at 58 °C and 1 min. at 70 °C. The presence of an amplification product was verified by the melting curve technique. Note that the highest level of expression is in C3H-1 except for Pax-6.
Also these CD34+ cells cultured in large numbers in vitro all showed the expression of genes from each stage of endodermal/pancreatic development from definitive endoderm to primitive gut to posterior foregut, pancreatic endoderm and endocrine precursors. Indeed, these cultured CD34+ stem cells all express genes for definitive endoderm Sox17 and CXCR4, primitive gut HNF1B and HNF4A, posterior foregut Pdx1 and HNF6 and pancreatic endoderm PTF1a, NGN3 and NKX2-2. These genes were expressed both at the mRNA level detected by RT-PCR and by semi-quantitative real-time PCR (Fig. 3) and at the protein level by immunocytochemistry for Pdx1, Ptf1a, Pax-6 and NGN-3 (Fig. 3) and Western blot (Fig. 3). These genes were expressed in CD34+ cultures from the three strains of mice examined: C3H, the C57Bl/6J transgenic mouse that expressed Green Fluorescent Protein, C57Bl/6-Tg(UBC-GFP)30Scha, and the C57Bl/6J transgenic mouse that expresses ß-galactosidase, B6.129S7-Gt(ROSA)26Sor/J.
The endoderm also gives rise to the primitive gut and then to the digestive tract including the intestine. A hallmark of the intestine is the presence of enterocytes localized in the mucosa, facing the lumen. This epithelium has a brush border, a specialized domain of the plasma membrane composed of microvilli. The axial-microfilament bundle of a microvillus contains a Ca2+-regulated actin binding protein, villin, the best characterized protein of the cytoskeleton of intestinal microvilli [6]. It has been shown that the amount of villin in mature enterocytes is very significantly higher than in immature intestinal (crypt) cells. We therefore investigated whether villin is also expressed in cultured CD34+ cells. Indeed, we found that villin is expressed at a very high level in these cells (Fig. 4).

Expression of villin in mouse non-adherent CD34+ cells in culture. Western blot analysis was carried out as in Fig. 1 with anti-villin antibody (Santa Cruz sc-7672) and showed a strong signal. The MW standard position (kDa) is shown on the left.
2.4 Human CD34+ cells express pancreatic lineage and embryonic stem cell genes
We previously examined human CD34+ cells collected from bone marrow and cultured by the same method as mouse cells but using human growth factors. These cells were found to display the same hematopoietic progenitor phenotype as the mouse CD34+ cells. Furthermore, they express the same neural genes as the mouse (unpublished results). Therefore, we investigated the expression of endodermal pancreatic lineage genes in the human CD34+ cells in culture. The human cells were found by immunocytochemistry to express Pdx-1 and Ptf1a (Fig. 5). Interestingly, the same human CD34+ cells also express embryonic stem cell genes Sox-2, Klf-4 and LIN-28 (Fig. 5).

Human hematopoietic progenitors express ESC and pancreatic lineage genes in cultures of CD34+ cells. Human bone marrow cells were cultured by the same method as mouse using human growth factors. The non-adherent CD34+ cells displayed the same hematopoietic progenitor phenotype as the mouse CD34+ cells. Immunocytochemistry was performed as in Fig. 1 with antibodies to human embryonic stem cell proteins Sox-2, Klf-4 and LIN-28 and pancreatic lineage proteins Pdx-1 and Ptf-1a (the same antibodies that were used for detection of mouse proteins). All cells in the cultures expressed the three ESC proteins and both pancreatic lineage proteins.
3 Discussion
The first question asked in this report was: are there embryonic-like stem cells in the adult and the answer is there apparently are such cells since we found that a subset of adult bone marrow stem cells expresses both the phenotype of hematopoietic progenitors and an array of ESC genes. The second question was: are these same cells pluripotent stem cells? Indeed, in addition to ESC genes these adult stem cells express genes of ectodermal, mesodermal and endodermal lineages suggesting that they may be pluripotent.
Although these adult stem cells express ESC genes and have the potential for pluripotency, they do not exhibit the unlimited proliferation of ESCs. The CD34+ cells have a limited lifespan since they stop dividing and plateau after 30–40 doublings like diploid somatic cells [5]. Accordingly, these CD34+ cells do not form teratomas when injected into a host [5] in sharp contrast to ESCs and iPSCs.
Unlike ESCs and iPSCs these adult stem cells spontaneously express, without induction, lineage genes from each of the three embryonic germ layers: ectoderm, mesoderm and endoderm. We previously reported ectodermal neural gene expression by these cells [5]. Here, mesodermal cardiac lineage genes MEL-2, an early cardiac stem cell transcription factor and differentiated myocyte troponin C and myosin light chain MLC-2 are expressed naturally by a subset of CD34+ cells–genes that have been reported by others to be induced in ESCs and iPSCs after in vitro manipulation [7–9].
Also this subset of CD34+ bone marrow stem cells spontaneously expresses endodermal lineage genes. Both early and late pancreatic lineage genes are expressed from definitive endoderm to primitive gut to posterior foregut and pancreatic endoderm. In addition they express a major structural gene of the intestinal epithelial lineage–another lineage derivative of endoderm.
The potential of bone marrow cells to express genes of the pancreatic lineage was previously suggested in a report indicating that human marrow-derived mesodermal progenitor cells could generate insulin-secreting islet-like clusters after inoculation into human fetal pancreas [10]. Also, human and murine bone marrow mesenchymal stem cells were shown to be induced to a pancreatic lineage after manipulation in vitro [10–13]. However, there are two main differences between these reports and our present findings. First, the cells previously studied by these groups are mesenchymal and do not express any of the hematopoietic progenitor markers of our CD34+ cells. Second and more importantly, in the previous studies the pancreatic lineage genes are not expressed in vivo by mesenchymal stem cells but are induced only by manipulation in culture as adherent cells. This is in sharp contrast with our present finding of a discrete population of CD34+ hematopoietic progenitors, which expresses pancreatic lineage genes ex vivo without any treatment or manipulation. As discussed above, this subset of CD34+ cells expresses markers typical of ESCs. In this context, it is of interest that ESCs also can be differentiated into insulin-producing tissue [14,15]. Furthermore, iPSCs from type-1 diabetes patients have been converted into insulin-producing cells [16] and iPSC-derived pancreatic beta-like cells can reverse the hyperglycemia in diabetic mouse models [17].
In this report, we have investigated properties of CD34+ cells originating from the bone marrow, both ex vivo and in culture. However, these CD34+ cells eventually leave the bone marrow and are found peripherally in the blood circulation. Although we have not investigated the properties of the circulating CD34+ cells, taking into account that these peripheral cells are non-adherent, one may speculate that these circulating CD34+ cells exhibit the same properties as those taken from the bone marrow that grow non-adherent in culture. The circulating CD34+ cells may thereby provide an autologous source for cell replacement by seeding organs, such as the pancreas, intestine or the heart. In this regard, it is of interest that some investigators have reported that blood enriched in CD34+ cells helps reduce hyperglycemia in diabetic patients [18]. Accordingly, one should consider the possibility that in vitro-expanded cultures of CD34+ cells might be utilized for cell replacement therapy by injection into the blood circulation or by direct inoculation into organs.
An earlier comprehensive analysis of gene expression by CD34+ cells [19] apparently did not report the ESC genes or embryonic germ layer lineage genes expressed in the subset of CD34+ cells investigated in the current study. It is known that CD34+ cells represent a mixed population of cells in bone marrow. It may be of interest that the genes reported here are present only in a subset of CD34+ cells and that subset was selected by our culture method.
Taken together, these readily available bone marrow CD34+ stem cells, that express embryonic stem cell genes as well as genes of each of the three embryonic germ layers (Fig. 6) and that exhibit the capacity to grow in very high numbers, are a potential source for cell replacement therapy in multiple tissues and organs.
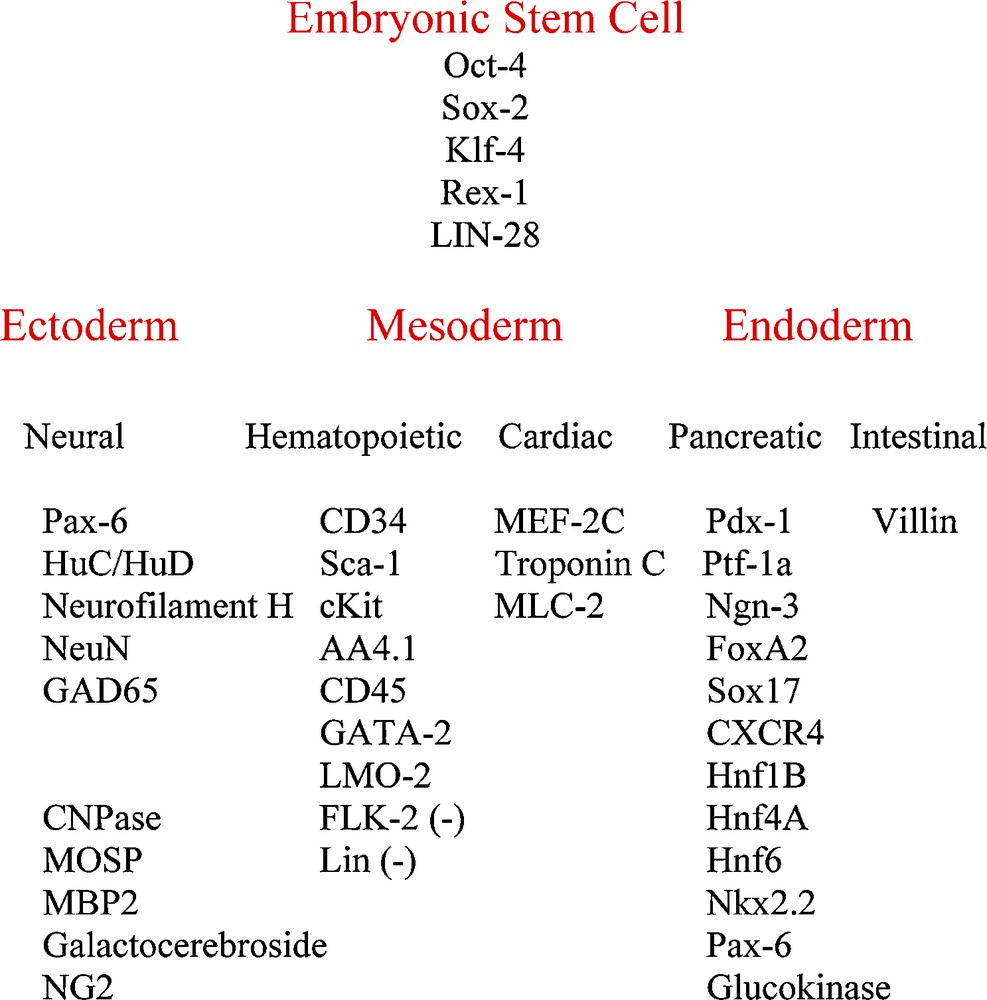
CD34+ stem cell gene expression. Oct-4, Rex-1 and the neural and hematopoietic genes were reported previously (5).
4 Materials and methods
4.1 Bone marrow CD34+ stem cell cultures
Non-adherent CD34+ hematopoietic stem cells were cultured from adult mouse bone marrow with medium containing mouse IL-3, IL-6, SCF and ß-mercaptoethanol as reported [5]. CD34+ cultures from the three strains of mice examined: C3H, the C57Bl/6J transgenic mouse that expressed Green Fluorescent Protein, C57Bl/6-Tg(UBC-GFP)30Scha, and the C57Bl/6J transgenic mouse that expresses ß-galactosidase, B6.129S7-Gt(ROSA)26Sor/J. The University of Maryland School of Medicine OAWA approved these animal studies. CD34+ stem cells were cultured, by the same method as mouse cells, from adult human bone marrow (Lonza Inc., Walkersville, MD) using human IL-3, IL-6 and SCF.
4.2 RT-PCR and semi-quantitative real-time PCR
CD34+ cell mRNA was analyzed by standard RT-PCR and semi-quantitative methods [5].
4.3 Immunocytochemistry
Cells were treated by standard immunochemistry methods and observed by fluorescence microscopy [5].
4.4 Western blot analysis of protein expression
Protein expression was determined on 4–12% polyacrylamide gradient gels (Invitrogen) by standard Western blot methodology [5,20,21].
Disclosure of interest
The authors declare that they have no conflicts of interest concerning this article.
Acknowledgements
B.P. was supported by Fondation Jérome-Lejeune. D.T. was supported by VA-REAP.