1 Introduction
The fact that organisms not just live passively in a given environment, but shape and change the environment around them, is essential to the understanding of biological evolution throughout the Earth's history. For example, the Precambrian increase in atmospheric O2 concentration, mainly as a result of oxygenic photosynthesis of cyanobacteria, changed the Earth's biogeochemistry fundamentally [1,2] and made the stunning evolution of oxygen-dependent life possible (e.g. [3]). The physiology of organisms, the biogeochemical processes they influence, and the evolving diversity of organisms interact in many ways and are intricately linked. The functional relationship between biogeochemical processes and biodiversity, as one specific aspect of these interactions, gained considerable interest in light of the rapidly decreasing biodiversity worldwide [4,5] and its possible negative effects on ecosystem properties and functioning [6,7].
The efforts towards a better understanding of how ecosystem functioning and biodiversity are related focussed mostly on the impact of species richness on some key processes such as primary productivity [8–10] and decomposition [11–13]. While some early experimental studies provided evidence that increasing species richness of herbaceous plants is positively correlated with net primary productivity [8,14], following discussions and assessments highlighted the complexity of this relationship requiring careful interpretations of such correlative evidence from artificially composed communities [15–17]. Depending on which of the different mechanisms such as selection effects, facilitation or resource partitioning is at play, the interpretation of any correlation between plant species richness and productivity can be fundamentally different [15,17,18]. Moreover, the relation between species richness and productivity is temporally dynamic with both plant species richness and productivity changing with ongoing community development [19].
Obviously, mechanisms of biodiversity effects on ecosystem functioning cannot be understood by counting species, and the particular characteristics of organisms and their distribution in time and space need to be considered. For example, assembling herbaceous plants into just three functional types, i.e. legumes, forbs and grasses, allowed to account for a significant amount of variation in most biodiversity-ecosystem functioning experiments regardless of how many species were used (e.g. [10,18]). The fact that the presence of nitrogen (N) fixing legumes was particularly important also provided an appealing mechanism for the observed positive biodiversity effects, that is increased N-availability at the community level, followed by higher productivity [18,20,21]. However, the presence of N-fixing plants is not a prerequisite for positive biodiversity effects on plant productivity [22,23]. Besides grouping species into broadly and arbitrarily defined functional types, the well-established concept of functional traits [24–26] allows a finer grained characterization of functional diversity on the basis of objective and continuous variables [26,27]. While these traits and associated functions are coherently assembled for higher plants [28,29], such generally accepted lists of traits only start to emerge for other groups of organisms (e.g. [30]). The lack of a unified framework of functional traits outside the plant kingdom is particularly critical for hyperdiverse communities organized in complex food webs such as soil organisms [31–34], for which a functional approach to diversity seems as one of few promising concepts to understand the role of biodiversity for ecosystem functioning.
With this article, we intend to explore the current status of functional approaches to the diversity of terrestrial microbial decomposers and their plant-derived substrates (plant leaf litter), and how functional diversity is linked to decomposition of organic matter as a key ecosystem process influencing the carbon and nutrient cycles. Unlike plant primary productivity that can be understood reasonably well by looking at plants as just one group of organisms within one trophic level, decomposition depends on many different groups of organisms across different trophic levels [32,33]. These different groups, such as earthworms, saprotrophic fungi, or fungal feeding micro-arthropods, their functional roles and their importance in driving key soil processes are well recognized and intensively treated in the literature (e.g. [31,32,34–37]). However, functional diversity within groups of organisms and its impacts on decomposition is much less studied. Our assessment will be largely limited to terrestrial ecosystems despite the many similarities with aquatic decomposer systems [13].
2 Decomposition and the functional diversity of plant litter
Recycling of carbon (C) and nutrients during decomposition of dead organic matter is of key importance for ecosystem functioning. It determines the balance between the mineralization and sequestration of C, and soil nutrient availability to a great extent [38,39]. Up to 90% of the global terrestrial plant production can enter the pool of dead organic matter [40] with subsequent mineralization during decomposition. Rates of decomposition vary strongly depending on environmental conditions – with temperature and moisture as the most limiting factors to decomposer organisms – and also on the chemical and physical quality of the organic matter [41–44]. Litter quality traits that exhibit strong control over decomposition typically include litter N concentration, lignin:N ratio and C:N ratio [44–46], but may also be unrelated to N in nontemperate ecosystems such as in the Amazonian rainforest [47,48].
Related to climate and soil dependent plant traits and habitat-specific dominance of plant functional types, leaf litter quality varies widely among plant species across broad geographical scales [49,50]. However, there is also substantial variation among co-occurring plant species at the local scale of the plant community under identical environmental conditions (see Fig. 1 with data from [51]). As a consequence, distinct litter types decompose together in mixtures rather than in isolation from each other. Litter mixture decomposition has been intensively studied in recent years and the majority of these studies showed that litter mixture decomposition could not be predicted from the decomposition of single litter types included in these mixtures, with predominantly synergistic mixture effects (see reviews by [12,13,52]). These nonadditive mixture effects typically show an idiosyncratic or no relationship with the number of litter species included in the mixtures (e.g. [11]; data shown in Fig. 2). The seemingly unpredictable litter mixture effects on decomposition seriously hinders the development of a general mechanistic framework of litter diversity effects on decomposition that may allow the assessment of the impact of global change induced alterations of plant biodiversity on organic matter turnover and C sequestration.
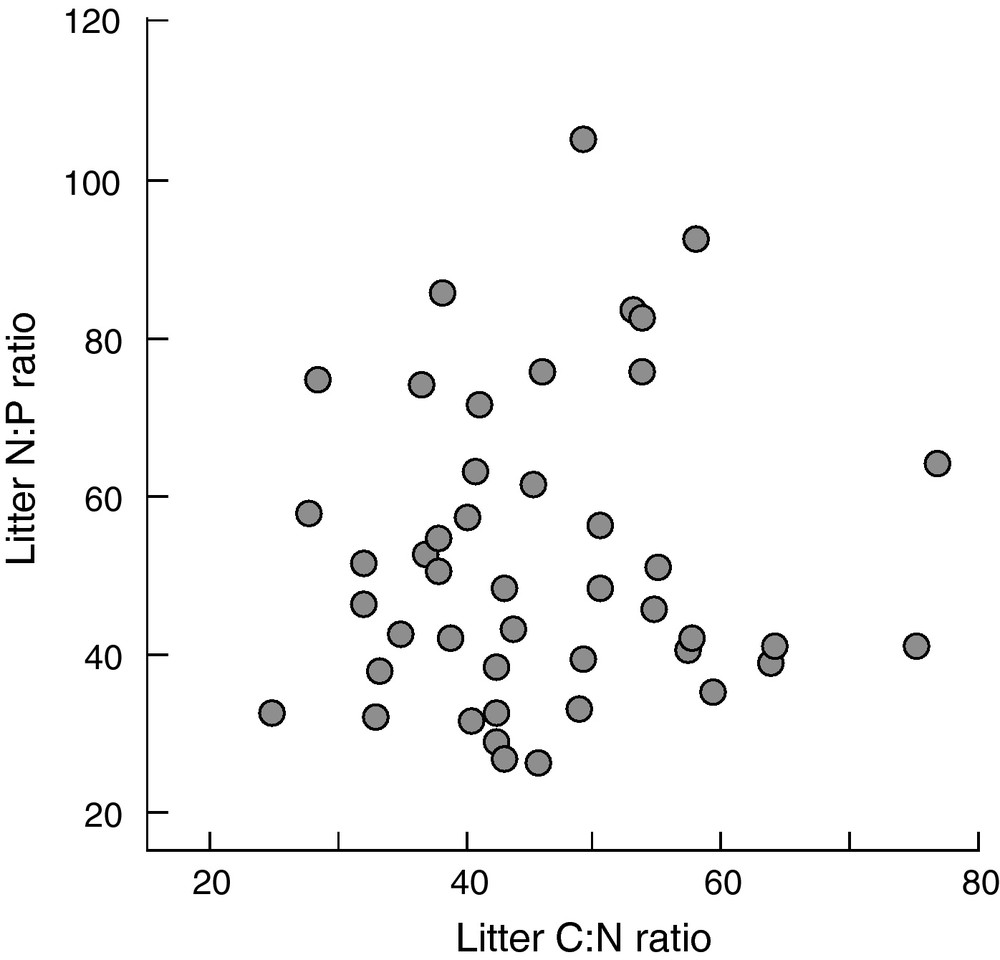
Leaf litter nitrogen: phosphorus ratio as a function of leaf litter carbon: nitrogen ratio from 45 Amazonian rainforest tree species co-occurring at the same site within a 0.98 ha forest area at Paracou, French Guiana. Each symbol represents the mean of a single species. Data from [51].

Litter mixture effect on litter mass loss measured after 300 days of decomposition in the field using litterbags as a function of litter species richness. The litter mixture effect is calculated as the observed litter mass loss (obs) of a mixture relative to the expected litter mass loss (exp) calculated from the corresponding single litter species treatments. Each symbol represents the mean (n = 4) of a specific mixture constituted from different species within functional groups (four sets: herbs from fertile cropping fields, grassland herbs, grasses and trees) or across functional groups (three additional sets). The filled circles connected with a line represent data points for grassland herbs as one of the total seven sets. Across all sets of species, the data show predominantly positive nonadditive mixture effects (i.e. litter mass loss is greater than expected) and no relation with litter species number. Within the set of grassland herbs, mixture effects on litter mass loss show an idiosyncratic relationship with litter species number. Data modified and redrawn from [11].
A few years ago, Epps et al. [53] developed a theoretical framework that allows making abstraction of litter species identity and arbitrary diversity measures such as taxonomic richness, by considering functional traits represented by the different litter types. These functional traits were defined on the basis of commonly used chemical parameters of litter quality characterization and were used to define a multivariate chemical diversity index as a weighted mean of compositional dissimilarity of litter mixtures [53]. Using published data, Epps et al. [53] showed that (1) there is considerable functional diversity within levels of litter species numbers, (2) the relationship between functional diversity and species richness varies among different plant communities, and (3) relationships between functional diversity and species richness can be negative, positive or idiosyncratic for the same set of litter types, but with three different series of additive mixtures (see Fig. 4 in [53]). According to the reasonable assumption that resource heterogeneity of litter mixtures drives the activity of decomposers and ultimately decomposition, they concluded that litter diversity–decomposition studies based on species richness as diversity measure almost necessarily result in idiosyncratic relationships, and that the proposed chemical diversity index based on Rao's quadratic entropy better reflects the functionally relevant diversity of litter mixtures [53].
In light of the rapidly developing body of literature on the pertinence and relevance of different measures of functional diversity [27,54–56], the considerations by Epps et al. [53] were not particularly novel in general terms, but these authors theoretically applied the concept of functional diversity for the first time to litter mixtures. The application of functional diversity to nonliving “remains of a plant community” may be somewhat unconventional. However, the functional traits for the characterization of plant leaf litter are largely the same as those used for green plant foliage [57] and are traditionally used as predictors for decomposition [50]. It is thus surprising that the convincing ideas and suggestions by Epps et al. [53] have only rarely been tested experimentally.
The first study applying an explicit approach of functional diversity for litter mixture decomposition used litter from four alpine herbaceous species [58,59]. These authors determined nine mostly C-related chemical litter traits, and calculated the Shannon diversity index for all litter treatments including all possible mixtures of the four litter types. The Shannon diversity index accounts for both the presence and abundance of all measured litter compounds, providing a chemical diversity index for all single species and multi-species litter treatments. Soil respiration and net N mineralization measured in microcosms with natural soil amended with the different litter treatments showed no correlation with litter species number [58]. In contrast, soil respiration increased and net N mineralization decreased with increasing chemical diversity, providing clear evidence that functional diversity based on chemical traits rather than species numbers affects decomposition in this study system [58]. Working in a different ecosystem of a nutrient-poor neotropical lowland rainforest, Hättenschwiler and Bracht Jørgensen [60] measured decomposition of four litter species that varied distinctly in their C:N and N:P ratios in a field experiment. Creating all possible combinations of these litter types, they tested the hypothesis that litter mixtures decompose faster with an increasingly heterogeneous C: nutrient stoichiometry because decomposers would exploit stoichiometrically diverse litter mixtures more efficiently. Stoichiometric diversity was expressed with a “dissimilarity index” using the functional attribute diversity (FAD2) introduced by Walker et al. [61]. In short, the FAD2 places litter mixtures composed of stoichiometrically different litter types at higher scores than litter mixtures composed of stoichiometrically similar litter types. In line with their hypothesis, decomposition proceeded faster with increasing stoichiometric dissimilarity of litter mixtures, but was not affected by litter species richness (Fig. 3). However, the positive relationship between decomposition and stoichiometric dissimilarity of litter mixtures was only found in the presence of soil macrofauna but not in their absence. Moreover, litter C quality (mostly the abundance of labile C compounds) had an overall stronger control over decomposition than stoichiometric dissimilarity. In a different study, Barantal et al. [62] tested the hypothesis that functional diversity correlates with litter mixture decomposition using an extensive set of 28 different litter mixtures created from a pool of litter collected from 16 Amazonian tree species. On the basis of eight chemical litter traits including N, P, and different C compounds, they used three distinct indices to describe functional diversity of litter mixtures in order to account for different aspects of functional diversity. Functional richness, functional divergence, and functional evenness were expressed with the FD index [63], Rao's quadratic entropy [53,64], and the Shannon index [e.g. 58], respectively. The frequent and at times strong nonadditive litter mixture effects on decomposition observed by Barantal et al. [62], however, were not correlated to either of the different functional diversity indices. Rather they found that the presence of particular species was determining nonadditive responses of litter mixture decomposition.

Litter mass loss (in % of total initial dry mass [d.m.]) after 204 days of decomposition in the tropical rainforest of Paracou, French Guiana as a function of litter species number (left panel) or as a function of stoichiometric dissimilarity (right panel). Soil macrofauna had access to the field microcosms. In the left panel, each symbol represents the mean (n = 4) of four single species treatments, six 2-species mixtures, four 3-species mixtures and one 4-species mixture. In the right panel, each symbol represents the mean (n = 4) of the 11 different mixtures along the dissimilarity gradient. Data modified and redrawn from [60].
The very few studies that experimentally explored how functional diversity of litter mixtures is related to the decomposition of these mixtures provided conflicting results. Some of these contrasts may be related to different study systems with different environmental constraints and/or to the use of different chemical litter traits for the calculation of functional diversity indices. Because this latter point is critical, Barantal et al. [62] calculated functional diversity from several possible combinations of the eight measured traits with essentially no difference among the correlations between mixture effects and functional litter diversity. However, it is still possible that some key traits have not been measured, and that consequently, the functional diversity indices may not reflect the critical differences among distinct litter mixtures well enough. The suggestion to characterize litter quality by means of reflectance spectroscopy such as diffuse reflectance infrared Fourier transform spectroscopy (DRIFTS) or near infrared spectroscopy (NIRS) [53] which provide an integrated quality measure, thus seems worthy of exploration in more detail. The evaluation whether functional litter diversity may or may not predict mixture effects on decomposition is currently data limited and would benefit greatly from a wider application in new experiments to come or in already existing datasets.
3 Functional diversity of microbial decomposers
Soil bacteria and soil fungi are highly diverse groups of organisms with recent estimates based on high throughput DNA sequencing techniques ranging roughly between 2000 and 20,000 Operational Taxonomic Units (OTUs) per gram of soil for bacteria [65,66] and between 1000 and 2000 OTUs per gram of soil for fungi [67,68]. The great diversity of microorganisms is paradoxically also the major obstacle in the understanding of the functional role of this diversity. It seems impossible to track and functionally describe each microbial taxon of a given community, and linking taxon identity to function is a major challenge in microbial ecology [69]. Consequently, microbial communities are currently treated as a “black box” in ecosystem models. Considering microbial diversity as unimportant may be problematic for the assessment of how ecosystem processes are impacted by biodiversity change (e.g. [70]). Indeed, soil microorganisms are by far the major drivers of soil carbon and energy flow and nutrient transformations and mineralization. Whether or not these key ecosystem processes depend on microbial diversity and to what extent microbial diversity is sensitive to environmental change is presently not well understood.
A theoretical model predicted a positive effect of microbial diversity on decomposition resulting from more intense microbial exploitation of organic matter through functional niche complementarity [71]. This theoretical prediction is supported by the classical culture-based laboratory work that established the specialization of different fungal species for different carbon forms suggesting resource partitioning (e.g. [72,73]). Further, successional dynamics of microbial communities with ongoing litter decomposition demonstrated that microbial communities shift with the progressive change in resource availability [38,74,75]. A major limitation of this early work was the artificial conditions of laboratory cultures excluding the majority of species that would naturally occur but that are not culturable [76]. Recent studies using soil from a temperate forest [77] or decomposed litter from a boreal forest [78] very elegantly circumvented the major methodological shortcomings of earlier studies. Adding either different C sources [77] or different N compounds [78] together with a nucleotide-analogue tag to the naturally established microbial communities, followed by quantitative polymerase chain reaction (qPCR) and sequencing of fungal communities, these authors were able to determine the structure and richness of active fungal communities under close to natural conditions. Both studies clearly showed resource preferences among fungal taxa resulting in shifts in community structure and richness as a function of different resources. The data from these studies are perhaps the currently most convincing experimental evidence for resource partitioning among soil microbial taxa under natural conditions. They further imply functional diversity of microbial communities and suggest that decreasing fungal diversity reduces the efficiency of the use of heterogeneous resources and thus the rate of decomposition.
For obvious reasons, direct manipulative tests of the effects of changing microbial diversity on ecosystem processes are difficult to perform and rely on either artificially reduced community diversity (e.g. dilution, fumigation) or on the synthetic composition of artificial communities from a few isolated and culturable taxa (e.g. [79–86]). These approaches are biased towards “resistant” or culturable taxa, which are unlikely to represent well natural microbial communities, as a major difficulty for data interpretation in a natural context as was already mentioned above. Despite this limitation, we will have a closer look at some of these studies and what they tell us about how process rates may depend on microbial diversity. We focus on two of these studies that allow the evaluation of mechanisms of diversity effects. This will permit to take the discussion a step further beyond taxonomic or genetic diversity towards functional diversity. The study by Tiunov and Scheu [85] nicely showed some of the operating mechanisms for diversity effects and the importance of functional aspects. They inoculated sterilized forest soil or powdered cellulose with five common species of saprotrophic fungi in all possible combinations from monocultures to all five species. Two species were fast growing cellulolytic species, one was also cellulolytic but relatively slowly growing, and two species were from the zygomycetes or the so-called “sugar fungi” that are not able to degrade cellulose. On both substrates, decomposition increased with increasing numbers of fungal species (Fig. 4). Selection effects, i.e. the increasing probability of the presence of at least one of the two fast-growing species in more species-rich communities, and complementarity effects, both contributed to higher process rates of more species-rich communities [85]. The use of two substrates, the complex multi-resource forest soil, and the simple single-resource cellulose allowed evaluating the “complementarity component” of the diversity effect in more detail. Counter expectation, the complementarity effect on forest soil was weaker than on cellulose resulting in an overall weaker positive relationship between community respiration and species richness on forest soil compared to cellulose (Fig. 4). This result is interesting in that it shows that facilitation among fungal species apparently contributed more to the overall complementarity effect than resource partitioning, a mechanism that should not operate on cellulose as the single uniform substrate. In particular, the presence of cellulolytic species facilitated the growth and activity of sugar fungi probably through the production of intermediate products during enzymatic degradation of cellulose. Tiunov and Scheu [85] further hypothesized that the sugar fungi may increase the enzymatic activity of the cellulolytic species through the uptake of inhibitory sugars. The facilitative interactions between these two “functional groups” of fungi, however, were not sufficient for the observed complementarity in the treatments with cellulose as the sole C substrate. The combination of any of the three cellulolytic species alone increased community respiration additively as if the three species grew on distinct substrates with complete resource partitioning. This surprising result is in line with a similar study that also used cellulose as the sole C source, but manipulated bacterial species richness to test diversity effects on cellulose decomposition [84]. In this study, microcosms were composed of bacterial communities of one, two, four, and eight species, randomly chosen from a pool of 10 cellulolytic bacteria, and maintained under constant environmental conditions. Despite the deliberate selection of “functionally redundant” species growing on the same resource, species richness facilitated species coexistence, increased the number of individuals, and increased cellulose decomposition in treatments with more than two species [84]. These results suggest again facilitation among a priori functionally similar species as the main mechanism of diversity effects. Such facilitation may arise due to small differences in the production of the required cocktail of cellulose degrading enzymes among species, or differential use of intermediate products, enzymes, secondary metabolites and metabolic waste products.
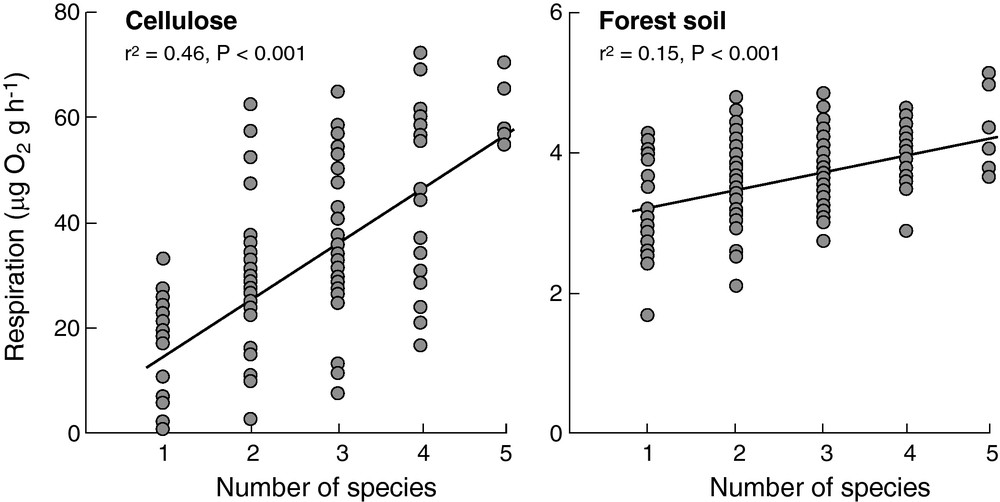
Community respiration of fungi as a function of fungal species richness on cellulose as the sole substrate (left panel) or on forest soil as a complex substrate (right panel). Data modified and redrawn from [85].
These two studies showed that microbial species from the same functional group of cellulose degraders growing on cellulose as the uniform substrate still exhibit “enough” functional dissimilarity that their combined presence in higher diverse communities results in higher process rates and in the case of the study by Wohl et al. [84] also in higher individual densities. Collectively, it seems that there exist ample possibilities for synergistic interactions among microbes through facilitation and resource partitioning even without environmental heterogeneity or strongly divergent resources. However, process rates are ultimately limited and the two studies that used wider species richness gradients beyond 10 species showed that process rates may saturate at relatively low [83] or higher levels [86] of species richness. A functional classification of microbial decomposers based on functional traits as continuous variables similar to the well-established trait framework for plants would greatly advance the possibilities to test and better understand the relationship between decomposition and microbial diversity. A functional approach to microbial diversity may also improve the predictability of the so far only generally and quite loosely described link between microbial community composition and ecosystem process rates (see [70] for review). In fact, changes in the composition and structure of microbial communities can be a rather poor indicator for associated changes in microbial-driven processes [87,88]. In particular, communities that are more similar regarding their taxonomic composition may not necessarily be more similar regarding their functional capabilities [87].
Some of the big questions surrounding functional approaches to microbial diversity are how to define functional diversity of microbes, and what and how to measure functional traits of microbes. While functional traits of macroscopic organisms usually correspond to physical or chemical characteristics measurable at the organism level and used as proxies of individual performance [26], this approach is not possible for microorganisms. Functional microbial traits instead will rather involve measures of the capacity of microorganisms to perform a particular function. However, the type of function and the organisational scale (individuals vs. groups of organisms) to consider, remain challenging. In the following, we will list some possible ways to deal with these questions that may allow working towards the ambitious goal of a functional classification of microbes. A useful, but rather coarse-grained approach is the definition of functional groups such as the contrasting groups of sugar fungi and cellulolytic fungi discussed above [85]. For example, in a recent paper, Miki et al. [89] defined microbial functional groups based on the competitiveness on specific substrates, and used these functional groups to incorporate the role of microbial diversity in a plant–soil microbe feedback model. Similarly, McGuire et al. [78] characterized the functional diversity of fungal communities using the response of individual taxa to specific substrates. Whereas these two previous studies characterized microbial functional diversity at the level of individual culturable strains/isolates, Moorhead and Sinsabaugh [90] proposed to define the functional role of microbial communities on the basis of broad chemical traits of plant litter. They suggested to distinguish just three guilds of microbial decomposers that use broadly different pools of organic matter: a guild of ‘opportunist microorganisms’ with high affinities for soluble substrates and high growth rates, a guild of ‘decomposer specialists’ with a high affinity for holocellulose and intermediate growth rates, and finally, a guild of ‘miners’ that is specialized for degrading lignin and grows slowly. This is a strongly simplified view of microbial functional diversity that, however, would permit the incorporation of at least some level of microbial diversity into decomposition models.
Some more detail in the assessment of functional diversity of microbial communities is possible with the use of the “community level physiological profiles (CLPP)” [91]. These authors proposed to use the catabolic potential of microbial communities for their functional characterization. The catabolic potential describes the range, the diversity, and the intensity of resource use of microbial communities when offered a wide range of different substrates. The catabolic potential characterizes the “functional ability” of entire microbial communities. Such functional characterization of the whole community was also recently used to assess rates of denitrification as a function of various carbon sources [92]. These authors used 16 different species of denitrifying bacteria growing alone or in combination on various carbon sources. Interestingly, they demonstrated that denitrification of a given assembled community could be calculated from the individual species performance. Hence, the performance of individual species constituting a specific denitrifier community allows predicting the “community niche”. However, while CLPPs or community niche approaches are useful to characterize and compare the functioning of entire communities under for example distinct environmental conditions, they do not really allow describing the functional diversity within communities. Moreover, CLPPs also failed to correlate with enzyme activity during decomposition [93] or with carbon mineralization (Fromin et al., unpublished) in other studies, suggesting that some key activities or processes are not always well predicted by CLPPs.
Molecular tools are probably among the most promising approaches to establish a connection between genetic identity and function of microbes, and the ecosystem processes they drive. Conventional molecular techniques targeting genes implied in specific functions have been used extensively for the characterization of microbial functional groups, but only rarely as a measure of functional diversity of microbial communities [94]. A particular difficulty in the context of how microbial diversity relates to decomposition is the fact that decomposition is a highly complex process involving many different enzymes and thus a multitude of genes coding for the expression of these enzymes. The recent developments in microarray and metagenomics technology offered new opportunities to assess the functional structure of microbial communities. For instance, the GeoChip [95] that contains > 24,000 probes and covers 150 gene families involved in biogeochemical C, N and P cycling is highly promising for studying the relationship between functional gene structure of the microbial community and ecosystem processes such as decomposition. For example, Zhang et al. [96] showed that the diversity of functional genes expressed under changing land cover tended to correlate with increasing amounts of soil organic carbon during decomposition. Finally, metatranscriptomic and pyrosequencing-based approaches also present highly promising tools for the assessment of microbial functional diversity, but these applications are still in its infancy [97,98].
Assessing functional diversity for the taxonomically very rich microbial decomposer communities is obviously very challenging. There are a number of promising new techniques and tools being developed that if applied in concert might allow this field of research to advance rapidly. At present, however, there is no single approach that would permit to describe the relevant functional microbial diversity for decomposition. Decomposition as such might be a too broad process to be described with a simple functional diversity measure of microorganisms. Instead, it might be more promising to focus on simpler processes driven by microbes, such as cellulose degradation or nitrification.
4 Conclusions
Our brief overview showed that plant litter and microbial diversity matters for decomposition of plant litter as a key ecosystem process influencing carbon and nutrient cycles. Considering numbers of taxonomic units appear overall as little helpful for the understanding and quantification of how decomposition depends on biodiversity. A functional classification of biodiversity based on functionally relevant traits of organisms seems a more promising approach. These functional traits are generally well-defined and easy to measure for plant litter, but currently much more difficult to establish for microorganisms. Despite easy access to plant litter traits, studies evaluating litter mixture decomposition that used explicit functional diversity approaches are very few. Some of these studies clearly showed that trait-based functional diversity predict litter diversity effects on decomposition better than litter species richness. It would be highly interesting to reanalyze the multitude of litter diversity experiments using a unified functional diversity approach, possibly with the application of alternative techniques such as DRIFTS or NIRS to characterize litter quality in an integrative way. Perhaps the biggest challenge for functional approaches to microbial diversity is how to define functional diversity of microbes, and what and how to measure functional traits of microbes. There are a number of promising new techniques and tools being developed such as the GeoChip that might allow significant progress in the near future.
Interactions across the trophic levels of plant-derived substrates and microbial decomposers were only implicitly treated in our analysis and would merit a closer examination. Such interactions may modify the impact of functional diversity within trophic levels on process rates [13,99,100]. Even if this is adding further complexity to the assessment of how biodiversity drives ecosystem processes, the incorporation of food web complexity is ultimately indispensable for a mechanistic understanding of biodiversity effects on ecosystem functioning [13,99,100].
Disclosure of interest
The authors declare that they have no conflicts of interest concerning this article.
Acknowledgements
Discussions and collaboration on different projects with Jean-François David, Ira Tanya Handa, Heidy Schimann, Sylvain Coq, Nicolas Fanin, Matty Berg, Stefan Scheu, Olaf Butenschoen, Brendan McKie, Mark Gessner, Eric Chauvet, Jasper van Ruijven, Rien Aerts and numerous other colleagues and students all stimulated the thoughts presented in this paper. Funding was provided from a CNRS ATIP (SDV) grant, a CNRS “PIR Amazonie II” grant, and the “BioCycle” project funded by a CNRS EDD grant through the European Science Foundation's (ESF) EuroDIVERSITY programme, and endorsed by DIVERSITAS.