1 Introduction
The hotspots1 approach makes it possible to focus conservation efforts on regions or areas containing exceptional concentrations of endemic species or experiencing unprecedented loss of habitat. The largely recognized interest of this approach is to sustain the largest possible number of species at the lowest cost [2,3]. However, two issues not considered in the evaluation of hotspots [4] need to be specifically addressed when considering river networks: linear inter-habitat coupling between streams, rivers and estuaries flowing across various terrestrial environments [5], and freshwater fishes as one of the most severely threatened biota worldwide [6]. Considering river networks as linear hotspots–hotlines across ecological regions–would help to address these issues.
First, the idea of hotline is consistent with the spatial connectedness of river networks and their role as natural corridors for the movement of nutrients and organisms [7]. River networks contribute to biodiversity in many landscapes on Earth as suggested by a representation of biomes in drainage basins of the largest river systems in the northernmost third of the world (Table 1). Second, such hotlines across ecological regions are characterized by their temporal flow variability which, as a specific perturbation regime for rivers, determines the structure and function of river networks, as well as many adaptations of aquatic and riparian species to dynamic habitat mosaics [8–10]. This “natural flow-regime paradigm” leads to the idea that flow management guidelines in distinctive regions may protect biodiversity as well as the goods and services provided by linear ecological systems such as rivers [11,12].
Biomes represented in the catchments of the 139 largest river systems of the northern third of the world (in parentheses: number of river systems where the biome dominates). Biomes are listed in order of increasing degree of river system exploitation (after [7]).
Biome | Number of river systems |
Tundra and barren arctic | 42 (14) |
Subtropical and temperate rain forests | 10 (1) |
Temperate needle-leaf forests | 85 (69) |
Temperate broad-leaf forests, and subpolar deciduous thickets | |
Northern provincesa | 5 (3) |
Southern provinces | 37 (28) |
Mixed mountain and highland systems | 29 (16) |
Temperate grasslands | 11 (3) |
Evergreen sclerophyllous forests | 11 (4) |
Cold-winter (continental) deserts | 8 (1) |
Lake systems | 5 (0) |
Warm deserts | 1 (0) |
In turn, freshwater biodiversity indicates how adequately we are providing “environmental flows–the quantity, timing and quality of water flows required to sustain freshwater and estuarine ecosystems and the human livelihoods and well-being that depend upon these ecosystems” [13]. It also bears witness to how well we manage river systems and the landscapes they drain [14]. Clearly, biodiversity preservation in river networks, including their connected riparian areas [15], is linked with the development of human societies, perhaps to a stronger extent than for other ecosystems since freshwater is a vital resource everywhere in the world.
Indeed, river networks are unique ecological systems in many respects. They have played a crucial role in the history of human societies through the provision of essential goods and services, but also through the consequences of flooding and water borne illnesses. Rivers interpenetrate every terrestrial ecosystem on Earth and influence many coastal marine environments [16]. Their channels vary enormously as a consequence of high and low flows which themselves are extremely variable in their structure [9,17,18]. Historically, this led human societies to construct dams and embankments along river courses, thus deeply modifying aquatic and riparian habitats, and affecting the dynamics of biodiversity at all levels of complexity from population to ecosystem processes.
Due to their linear structure, river networks require specific strategies for their preservation, as developed in the first part of the present review. The following parts of the review illustrate the importance of linearity to address four issues of biodiversity preservation in river networks, namely: risks of extinction, barriers to migration, hybridization in headwater streams, and biotic homogenization of rivers. The two final parts deal with implications of linearity for the future of river networks in a changing climate, and for human security along rivers.
2 River preservation needs specific strategies
In continental environments, the design of protected areas has been generally driven by terrestrial rather than by freshwater habitat features [19]. This emphasis may be partly coherent with the influence terrestrial ecosystems have on the integrity of adjacent freshwater ecosystems [20]. However, the protection of terrestrial habitats cannot always insure an adequate protection of rivers, as shown by a recent study of continental waters in Michigan [21]. In this state, protection based on terrestrial environments insufficiently protects aquatic and riparian habitats, particularly along small upstream channels and large downstream rivers: these zones are only protected through measures such as those of the National Wild and Scenic Rivers program or the Michigan Natural Rivers program which are river specific.
Indeed, the protection of river biodiversity demands specific strategies such as flow regime restoration and integrated management of drainage basins [19]. First, habitat and species conservation must go hand in hand with the protection of fundamental processes such as subterranean water recharge. Second, the functioning of reservoirs and exotic species invasions may annihilate the benefits of protecting the surrounding terrestrial environment [19,22], or river sections may be impacted by the degradation of terrestrial areas kilometres upstream [19,20]. In addition, river sections frequently delineate boundaries for reserve areas, and thus are protected only along one bank.
Clearly, the traditional idea of protected area hardly applies to river networks which are essentially linear and largely influenced by zones far away from the sections to be protected [19] (Fig. 1). Notions of freshwater focal area, critical management zone, and catchment management have been suggested to integrate terrestrial, freshwater and marine systems. However, it is not possible to transform entire drainage areas, most of them inhabited, into natural reserves. The concept of integrated river basin management (IRBM) seems more appropriate as it coordinates “conservation, management and development of water, land and related resources across sectors within a given river basin, in order to maximize the economic and social benefits derived from water resources in an equitable manner while preserving and, where necessary, restoring freshwater ecosystems” [23]. Such a concept may allow the development of a coherent network for the conservation of freshwater fish species as suggested, for example, in France [24].
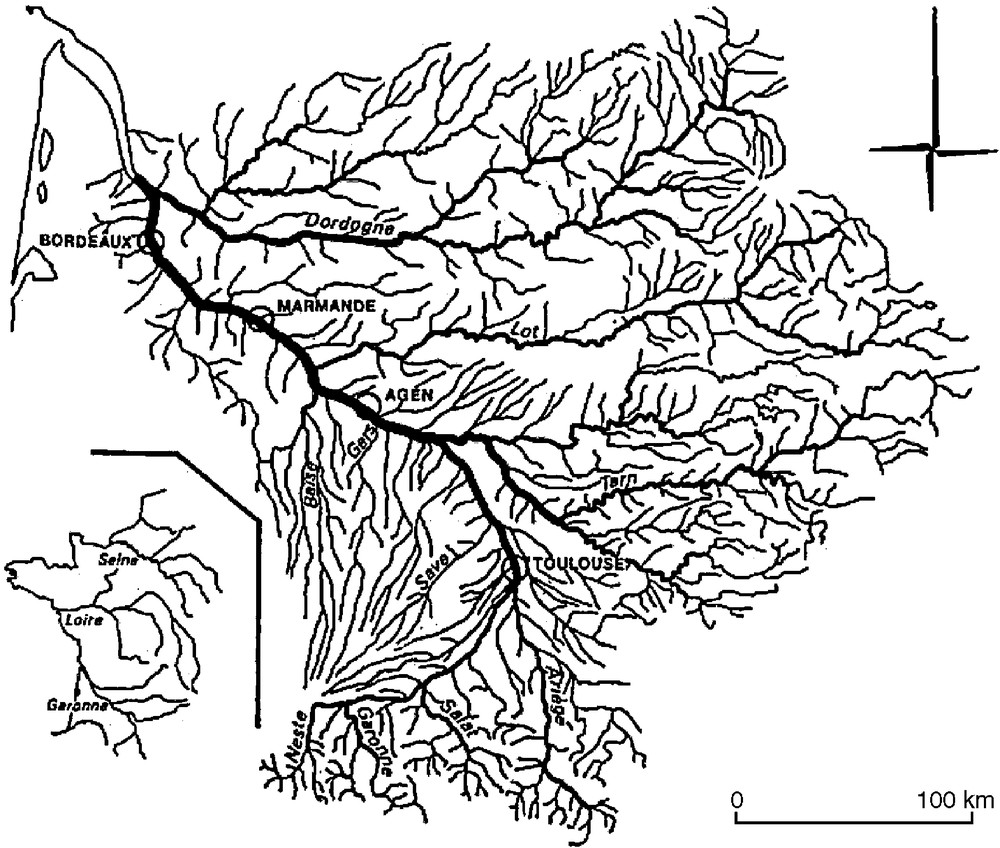
The Garonne and Dordogne Rivers in South-West France illustrate an example of a linear system organized in a complex and connected network, open to the Atlantic Ocean through a large estuary. They represent hotlines for sharing water between ecological and societal systems.
In this perspective, mapping all freshwater habitats on Earth through an ecoregion approach may facilitate identifying outstanding and threatened systems. Such a map is now available [25]: it includes 426 units, distinguished on the basis of the distribution of 13,400 world freshwater fish species and incorporates major ecological and evolutionary patterns. It reveals that more than 6900 of these species are endemic, i.e. present in a single ecoregion–a characteristic feature of river fish species, expected to be confirmed when the distributions of groups such as invertebrates, reptiles, and amphibians will be included.
From an evolutionary perspective, spatial and temporal habitat dynamics have largely driven life in flowing waters [26]. For million years, aquatic flora and fauna have had to adapt to ever changing life conditions in linear river networks, especially those where fragmentation, isolation, migration and recolonisation caused by extreme floods and droughts exerted strong selective pressures on populations. Such conditions are particularly important for the origins of biodiversity. Approximately 126,000 animal species have been recorded in freshwater, i.e. 9.5% of the total number of animal species recognised globally for an area of about 0.01% of the total surface of the globe. In addition, the 2614 recorded aquatic species of macrophytes amount to about 1% of the total number of known vascular plants, and although algae and cyanobacteria are reasonably documented, microorganisms such as bacteria, viruses, Protozoa and Fungi are notoriously understudied [27]. Linkages of rivers with other surface and groundwater systems from sources to estuaries have shaped the evolution of life in river networks–with appearances and disappearances of species in a movement of constant production of diversity, at all levels of complexity, up to and including the coupled physical landscape [28].
However, a large proportion of these species are threatened with extinction, as regularly reported by the International Union for Conservation of Nature (IUCN) Red Lists [29]. For example, trends assessed more than ten years ago in North America, one of the best documented regions in the world, are still valid: projected mean future extinction rate for freshwater fauna is estimated to be about five times larger than the rate for terrestrial fauna and three times that for coastal marine mammals; the recent extinction rate at the regional scale for freshwater fish is estimated as 1000 times higher than that for freshwater fossil fish species [30]. Despite incomplete data for many other regions, current trends for molluscs, amphibians, turtles and fish suggest that freshwater environments are stricken by a disastrous biodiversity crisis [31,32].
3 Heading to extinction?
Observed trends suggest that since 1970 freshwater species are declining more rapidly than marine or terrestrial species (Fig. 2). In this context, the question arises as to whether certain taxa of river species are more likely than others to move from “vulnerable” to “endangered” and “critically endangered” before extinction [33]. This section addresses the cases of rare and of large species, before considering the example of amphibians–an emblematic threatened freshwater taxon, among others.
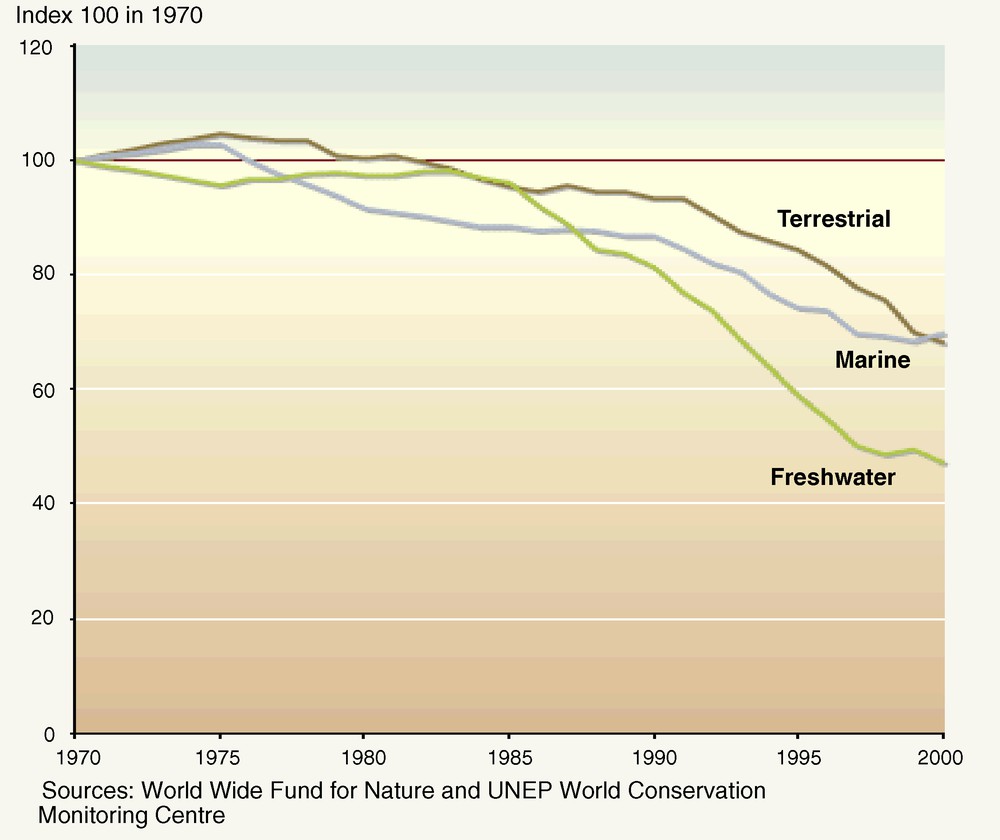
Trends in freshwater, marine, and terrestrial living planet indices 1970–2000 (after [149]).
3.1 Rare species
Rarity is a component of the biological diversity of freshwater ecosystems: about 13,400 fish species live in freshwaters, many as small isolated populations restricted to a few sites [25,34]. Such a diversification certainly has been facilitated by habitat heterogeneity and geographical isolation of lakes and streams. Isolated and rare small populations have persisted for long periods in freshwater environments, although rare species normally are considered as being at risk of extinction more than others. These populations may be less affected by competition and better protected against predators, parasites and pathogens, and their genomes may be locally adapted and purged of deleterious mutations. On the other hand, stream connectivity may facilitate rescue effects when these populations are declining.
Nevertheless, naturally rare species seem to be particularly vulnerable to human activities, as shown when narrowly distributed species are compared with more widely distributed related species [35,36]. Moreover, naturally rare freshwater species are likely to come under the anthropogenic Allee effect [37] just because they are recognised as rare by the public, and given more value. This vulnerability also characterises invertebrates: the decrease of melt water contribution to flows in alpine streams under climate change tends to decrease the abundance of rare endemic macro-invertebrate species adapted to low water temperatures and high suspended sediments [38]. Issues of biological conservation in freshwaters need to consider uncommon species [39], as well as range extents, habitat specificities, and local population sizes [40], particularly so for species of the “lower” taxonomic groups such as worms, rotifers, and others that, common or uncommon, remain poorly known.
3.2 Large species
Large freshwater fishes are particularly threatened by overfishing and habitat degradation everywhere in the world. In 2007, the National Geographic Society launched a stimulating “Megafishes Project” to document and to protect these giant species (http://megafishes.org/). The twenty largest of these species live in ten river basins and three lakes, and the status of many of them is unknown (Table 2).
The twenty largest fish freshwater species weighing more than 91 kilograms (200 pounds) or measuring more than 1.83 meters (6 feet) long (after [150]).
Mekong River Basin: Giant freshwater stingray* Himantura chaophraya (500 cm, 600 kg), Mekong giant catfish*** Pangasianodon gigas (300 cm, 300 kg), Giant barb Catlocarpio siamensis (300 cm, 300 kg), Giant pangasius (dog-eating catfish) Pangasius sanitwongsei (300 cm, 300 kg), Wallago (giant sheatfish) Wallago attu (240 cm) |
Mississippi River Basin: Alligator gar Atractosteus spatula (305 cm, 137 kg), Mississippi paddlefish* Polyodon spathula (221 cm including paddle), Pallid sturgeon** Scaphirhynchus albus (200 cm, 130 kg) |
Amazon River Basin: Arapaima (pirarucu; paiche) Arapaima gigas (450 cm, 200 kg), Piraíba (laulau; lechero) Brachyplatystoma filamentosum (360 cm, 200 kg) |
Amur River Basin: Soldatov's catfish Silurus soldatovi (400 cm), Taimen Hucho taimen (200 cm, 100 kg, also in Selenge River Basin and in Lake Baikal) |
Yangtze River Basin: Chinese paddlefish*** Psephurus gladius (700 cm, 500 kg) |
Europe and Asia: Wels catfish° Silurus glanis (500 cm, 306 kg) |
Brahmaputra River Basin: Putitor mahseer Tor putitora (275 cm) |
Tigris River Basin: Mangar Barbus esocinus (230 cm) |
Nile River Basin: Nile perch Lates niloticus (200 cm, 200 kg) |
Murray River Basin: Murray cod Maccullochella peelii peelii (200 cm, 113.5 kg) |
St Lawrence and Great Lakes: Lake sturgeon Acipenser fulvescens (274 cm, 125 kg) |
Lake Tanganyika: Tanganyika lates (giant perch)** Lates angustifrons (200 cm, 100 kg) |
Dams, industrial effluents, and commercial navigation threaten large-bodied species, e.g., in the Yangtze where the Chinese paddlefish Psephurus gladius may be seen as a “living dead”. Some large species can still be preserved from extinction through sound management such as has been accomplished with the lake sturgeon Acipenser fulvescens formerly decimated by pollutants, lost of habitat and angling in the St Lawrence River and the Great Lakes [41].
Fish are not the only threatened large species living in rivers. The probable extinction of the Yangtze River dolphin, Lipotes vexillifer or baiji [42] attracted attention to the future of all river dolphins and porpoises. These emblematic species live in the Ganges, Indus, Yangtze, Mekong and Amazon–among the mightiest rivers on Earth, the Asian ones flowing through areas densely populated by humans. In these rivers, dolphins are heading toward extinction through pollution, accidental capture by fishing nets, disturbance by and collisions with boats, and the construction of dams. Their position at the top of aquatic food chains and their low intrinsic population growth rates make them particularly vulnerable to human activities (Table 3). In addition, river dolphins are rare and large species, two basic conditions of vulnerability that they share with other emblematic threatened freshwater species, including crocodilian species [43].
Dolphins and porpoises living in freshwaters (after [151]).
Name | Range | Estimates | Main threats | IUCN red list status |
Ganges river dolphin (blind dolphin or susu) Platanista gangetica gangetica |
Isolated subpopulations in small pockets of the main Ganges and Brahmaputra rivers and a few tributaries in India and Nepal, as well as in the Meghna river system in Bangladesh. A small subpopulation of tens of individuals in the Karnali River in Nepal | From 4000–5000 individuals in 1982 to fewer than 2000 in 1997. Probably 1200–1800 individuals left at the present time | Habitat destruction and fragmentation due to water infrastructure for irrigation, hydropower, and flood prevention. Industrial and agricultural pollution. Entanglement in fishing gear | Endangered |
Indus river dolphin (bhulan) Platanista gangetica minor |
Now restricted to five subpopulations in an approximately 1,300-km stretch of the river Indus in Pakistan, separated by irrigation barrages | Increased from around 1200 in 2001 to 1600 – 1750 individuals in 2006 | Agricultural practice, habitat fragmentation, water extraction, entrapment in canals, illegal gill nets | Endangered |
Irrawaddy dolphin Orcaella brevirostris |
Freshwater subpopulations in the Mekong River, the Mahakam River (Kalimantan, Indonesian Borneo), and the upper reaches of the Irrawaddy River (Myanmar) north of Mandalay |
71 individuals for the Mekong subpopulation (2007), 70 for the Mahakam subpopulation, and 58–72 for the Irrawaddy subpopulation (most recent surveys estimates) | Gill nets and other destructive fishing methods, projected construction of dams on the lower Mekong, habitat degradation through sedimentation, pollution from coal and gold mining, prey depletion due to illegal and unsustainable fishing methods | All three subpopulations Critically Endangered |
Yangtze Finless porpoise Neophocaena phocaenoides asiaerientalis |
Lower and middle reaches of the Yangtze River, as well as in Poyang and Dongting adjacent lake systems | Around 1000–1200 individuals in the river plus 600 in the two lakes (2006) | Habitat loss and degradation as a result of water infrastructures and pollution, prey depletion due to overfishing | Endangered |
Yangtze river dolphin (or baiji) Lipotes vexillifer |
Lived in the lower and middle reaches of the Yangtze River, Fuchun River, and Dongting and Poyang lakes in China | 6000 individuals in 1950, 200 in 1990, 7 in 1998, and not found in 2006 | Idem | Critically Endangered. Probably extinct |
Amazon river dolphin (pink river dolphin or boto) Inia geoffrensis |
Amazon and Orinoco river basins: in a variety of riverine habitat types, including rivers, small channels, confluences, and lakes | Probably tens of thousands. The most abundant of freshwater cetaceans | Fishing activities, hydropower development | Data deficient, considered vulnerable in Columbia, Peru and Brazil, and endangered in Ecuador |
Bolivian river dolphin (bufeo) Inia boliviensis |
Endemic to Bolivia, white water and clear water flood plains | Not known | Fishing activities, hydropower development, mercury pollution from small-scale gold mining activities | Not yet assessed, considered vulnerable in Bolivia |
Grey river dolphin (tucuxi) Sotalia fluviatilis fluviatilis |
Amazon river and main tributaries; Lower Orinoco | Not known | Fishing activities, hydropower development | Data deficient, considered vulnerable in Colombia and endangered in Ecuador |
3.3 Amphibians
In 2004, the IUCN Global Amphibian Assessment (http://www.globalamphibians.org) reported on the distribution, abundance, population trends, habitat associations, and threats for 5743 then described species of amphibians [44]: 32.5% of these species were globally threatened (vulnerable, endangered or critically endangered in the IUCN Red List). Although clearly higher than those for birds and mammals, this percentage is certainly an underestimate as the status of many rare amphibian species could not be gauged. More recent assessments confirm that nearly one-third of the world's amphibian species are threatened out of a total estimated 6785 amphibian species as of January 18, 2011 (http://www.amphibiaweb.org/).
Among the “rapidly declining” species mentioned by Stuart et al. [44], “enigmatic declining” species were surprisingly numerous. This included species declining for reasons that are not fully understood, with disease and climatic change being suspected most often. The percentage of enigmatic declining species increased steadily when going from the least to the worst extinction risk in the IUCN categories (from 9.7% in “near threatened” to 92.4% in “critically endangered”) reflecting the vulnerability of these species when considering extinction risk, particularly in streams at high altitudes in the tropics where occurrence of the fungal disease chytridiomycosis is higher. However, reduced amphibian survival occurs worldwide, associated with habitat destruction, climate and land use changes, pollution, ultraviolet radiation, species invasions, as well as chytrid outbreaks. Overexploitation is also a factor to consider in East Asia, the United States, and… France [45]. Interactions between all these factors are complex, requiring further documentation; for example, to understand what conditions amphibians require at their aquatic larval stage [46], how they are influenced by current and historic landscape characteristics [47], or how global warming alters patterns of cloud cover thereby reducing ultraviolet exposure in some places and increasing it in others [48]. Unprecedented conservation programs for amphibians are certainly indispensable as proposed under the Amphibian Survival Alliance, with in situ surveys, monitoring and habitat protection, coordinated with ex situ husbandry actions [49].
3.4 Conservation and restoration efforts
Every year, the status of various freshwater species move from vulnerable to endangered and to critically endangered in the IUCN Red List. Conservation efforts certainly slow this decline, particularly in reducing habitat loss and exotic species invasion [50]. Nevertheless, these efforts require addressing multiple factors causing population declines along river networks, as has been done for groups such as turtles (http://turtleconservationfund.org), amphibians (http://www.globalamphibians.org), and mussels [51]. They also require improving our knowledge of the status of freshwater species that are not well known, particularly invertebrates which play a crucial role in the complex food webs that characterise production and decomposition in flowing water ecosystems [52,53].
Restoration is another promising and developing activity for rivers in Europe and North America, for example under the European Centre for River Restoration (ECRR) and the National River Restoration Science Synthesis (NRRSS). Large sums are spent every year in land acquisition, bank or channel reshaping, flow regime enhancement, wetland construction, vegetation plantation, native species reintroduction, and related activities [54]. However, the outcomes of these efforts are generally not adequately assessed, monitored or communicated at levels which improve future practices. Among the criteria proposed for measuring success of ecological restoration [55], an important one is to complete pre- and postassessments and to make data publicly available. Too often, restoration addresses the hydromorphology of relatively short river sections, improving habitat diversity but not biodiversity at the scale of the river network [56]. Such a scale has been addressed, for example, in a recent proposal for fish and wildlife restoration of the Columbia River, with a focus on food webs as a basis for growth and vitality of fishes in both the freshwater and estuarine sections of the river basin [53]. In this river–the largest hydroelectric producer in the US–this focus would complement the traditional but insufficient approach of “hydrosystem, habitat, hatcheries and harvest”, and improve the capacity of the Columbia Basin to produce fish through food webs having both new and old elements caused by rapidly changing environmental conditions.
Conservation and restoration efforts also require accounting for the evolutionary history of species under consideration. In Europe, two major events profoundly affected fish distribution in river networks: the Messinian salinity crisis of the Mediterranean Sea (5 MA years ago) and the Last Glacial Maximum (18,000 years ago). These two events are at the origin of the mechanisms of speciation and extinction, which greatly influenced fish diversity in European river networks. The net result was the highest species richness occurring in the Central Peri-Mediterranean and, to a lesser degree, Ponto-Caspian Europe biogeographical regions [6]. However, consideration of linear connections within and between European biogeographical regions is also needed to explain expansions along river networks, as is well illustrated by the Ponto-Caspian mysid shrimp Hemimysis anomala. This species has recently invaded Northern and Western Europe along two pathways traced using information from mitochondrial DNA [57]. One pathway was through the Baltic Sea and further to the Rhine delta, probably from a population introduced to a Lithuanian reservoir of the lower Dniepr River in 1960. The other pathway was up the Danube River from its delta to the Main-Danube canal and then down to the Rhine River delta. The two lineages seem to have met and may be found intermixed at several NW European sites.
3.5 Conclusion
To the question: “are species in river networks heading to extinction?” the answer is that there will always be some species able to tolerate the new conditions. However, the native biodiversity will, in all likelihood, be reduced to very low level if conservation and restoration efforts are not better developed and implemented. Such efforts must be based on biological knowledge, and large and rare species may offer interesting case studies to understand the mechanisms by which river species are so vulnerable to extinction provoked by human actions. Restoration efforts also need to be communicated between scientists, decision makers, and the public. Here again, the linear structure of river networks should not be forgotten. Conserving or restoring a river section is useless without paying attention to the quality of sources and headwater channels as well as to the estuaries and large channels downstream.
4 Barriers to migration
River networks are routes for migration used by diadromous (migratory between saltwater and freshwater) fish species. These species can cover long distances from sea to reproduce in rivers and return (anadromous) or, inversely, from rivers to reproduce in sea and return (catadromous). All have been adversely affected by human activities: various barriers to migration (e.g., dams), as well as habitat degradation, over exploitation, and pollution (see [58]).
Time series analyses of the 24 diadromous fish species living within the North Atlantic basin indicate that the relative abundances of 13 species have declined to less than 98% of their historical levels, whereas the relative abundances of the 11 other species have declined to less than 90% [59]. The length of time series data varied according to the species, some beginning in 1880 (Alosa alosa), others in 1950 (Anguilla anguilla). Damming was a main driver of the declines and, as shown by several examples, dam removal has been the most useful recovery action. Overfishing and pollution were two other important factors of decline.
Historically, robust populations formed the basis of important fisheries in the 19th century. Then, some populations became extirpated–at low pace through the mid-20th century, more rapidly thereafter. Cumulated impacts resulted in species declines and scarcity, followed by collapses in fishery demands and profit relative to other uses of river networks. Collectively, these changes reduced motivation for management and restoration measures that would favour fish [59]. This downward spiral led to societal disinterest and not only resulted in loss of fish species, it also led to the loss of services provided by the disappearing species. If it is decided to eventually restore the fish populations, maintaining healthy populations of diadromous fish within healthy ecosystems not only will be complex from a societal perspective, it will take decades of effort and numerous tradeoffs.
The example of the European eel illustrates the multiple approaches needed to restore populations of a species where the numbers have fallen severely–by as much as 99% since the 1970s according to some estimates [60]. Contaminants are certainly a cause of decrease in European eel populations [61]. With dams, fishing, recently introduced parasites, and possible changes in ocean currents, they may collectively contribute to prevent the eels to complete their biological cycle. Transporting and stocking young eels in the upper parts of river basins has been attempted, for example recently in Germany where it is hoped that the transported eels will not loose their navigational imprinting. Since 2007, an “eel management plan” requires each country of the European Union to limit eel mortality so that the number of eels migrating to the ocean will be at least 40% of the theoretical number estimated in the absence of human impacts. However, such a plan would need to be adjusted to the variable quality of eels leaving countries extending from Iceland to the Mediterranean region, and to their variable ability to migrate to and reproduce in the Sargasso Sea. Breeding eels in captivity also has been suggested as a way to reduce fishing pressure on wild populations, a technique also applied to other species with mixed results.
Other plans aim at reversing downward trends and restoring migratory fish species to their historical range of 1900–a range that may not be suitable in the context of existing conditions (land uses). Here, predictive models are needed to assess the potential suitability of river basins for restoration projects under different global warming scenarios. Lassalle et al. [62] have developed distribution models for 21 migratory fish species out of 28 from Europe, North Africa and the Middle East (Table 4), in order to explore the potential consequences of climate change and its implications for current conservation plans. The authors suggest that future management action should be preceded by an assessment of the suitability of the conservation unit (basins, area, country) under global warming. Such an assessment should be based on the most optimistic and the most pessimistic emission scenarios of IPCC, at the temporal scales often used in projection studies: 2020, 2050 and 2100. It remains to explain the results of such scenarios: for example, under one of the most pessimistic emission scenarios (3 °C warmer than the 1900–1910 level), the Rhine basin would be still suitable in 2100 for Alosa alosa populations, but not the nearby Meuse and the Weser catchments [62,63].
Twenty-eight diadromous fish regularly encountered in Europe, North Africa and the Middle East (after [62]).
Latin name | Common name | Type | N | IUCN |
Caspiomyzon wagneri | Caspian lamprey | A | 10 | |
Lampetra fluviatilis | River lamprey | A | 89 | LR/nt |
Petromyzo marinus | Marine lamprey | A | 107 | LR/lc |
Anguilla anguilla | European eel | C | 175 | CR |
Acipenser gueldenstaedtii | Russian sturgeon | A | 24 | EN |
Acipenser naccarii | Adriatic sturgeon | A | 8 | VU |
Acipenser stellatus | Stellate sturgeon | A | 30 | EN |
Acipenser sturio | European sturgeon | A | 72 | CR |
Huso huso | Beluga | A | 26 | EN |
Alos algeriensis | A | 3 | DD | |
Alosa alosa | Allis shad | A | 63 | DD |
Alosa caspia | Caspian shad | A | 4 | |
Alosa fallax | Twaite shad | A | 104 | DD |
Alosa immaculate | Black Sea shad | A | 14 | DD |
Alosa kessleri | Black spined herring | A | 2 | DD |
Alosa tanaica | A | 14 | ||
Alosa volgensis | Volga herring | A | 2 | DD |
Vimba vimba | Vimba | A | 43 | LR/lc |
Osmerus eperlanus | Smelt | A | 45 | DD |
Coregonus oxyrinchus | Houting | A | 3 | DD |
Coregonus sp. | A | 25 | DD | |
Salmo caspius | Caspian trout | A | 13 | |
Salmo labrax | Black Sea salmon | A | 17 | |
Salmo salar | Atlantic salmon | A | 96 | LR/lc |
Salmo trutta | Brown trout | A | 137 | LR/lc |
Salvelinus alpinus | Arctic char | A | 30 | LR/lc |
Liza ramada | Thin-lipped mullet | C | 127 | |
Platichthys flesus | European flounder | C | 138 |
A critical first step toward maintaining healthy populations of diadromous fish species is clearly to restore river networks and their connectivity with coastal marine ecosystems, as illustrated, for example, by the Columbia River [64]. According to Nilsson et al. [65], half of the world's large river systems (172 out of 292) are affected by dams which act as barriers to fish migration, particularly in Europe where nonfragmented large river networks are rare. In the United States, 600 dams (out of 3500 deteriorated or no longer useful) have been removed for safety and economic concerns, resulting in rapid recovery of invertebrate, fish, and riparian vegetation [66]. However, such removals are always complicated and expensive, and a source of societal tension. This is particularly so when rivers cross several countries, such as the Danube in Europe, the rivers flowing across the Middle East or the Lancang-Mekong in Asia [67]. This is also the case in China where about 110 rivers and lakes cross or form borders with 19 countries [68].
5 Headwater streams and the effect of fish introductions
Headwater streams are essential in maintaining biodiversity and ecosystem processes of entire river networks: they offer refuges from temperature and flow extremes, protect resident species against competitors and predators, provide spawning sites and rearing areas, and constitute a rich source of food [69]. Hundreds to thousands of invertebrate species generally live in springs and first to second order streams of a river network. However, human activity threatens particularly small headwater streams through agriculture, logging, mining and urbanization, contributing to the loss of ecological integrity in ecosystems downstream [70].
Hybridization is a general issue in river networks, particularly so concerning genetic diversity of European salmonids in headwater streams. For several decades, exotic cultivated salmonids have been intentionally introduced in headwater streams to increase the size of in situ populations [71]. They have mixed to wild populations, reducing the fitness of wild fish through genetic introgression, loss of gene diversity, and sometimes extirpating local populations [72]. Thus, in the Mediterranean region, brown trout (Salmo trutta) have been subjected to extensive introgression as a consequence of stocking genetically divergent cultivated individuals from the Atlantic region [73,74]. The resultant genetic erosion threatens the diversity of wild trout stocks in Pyrenean drainage basins open to the Mediterranean Sea [75]. Hence, the concept is to establish genetic refuges to maintain and restore the autochthonous gene pools of trout populations in various headwater streams by banning the release of farmed individuals [76]. To be efficient, these refuges must be established in cooperation with local residents and administrators who are aware of the problem of introgression caused by the introduction of alien individuals.
Besides losses of gene diversity within populations, introducing and stocking nonnative fish–often top predators–change the structure of in situ communities [77,78]. The consequences may be important at the level of ecosystem processes, for example increasing top-down effects in trophic networks, modifying nutrient cycles, reducing the exchanges with surrounding terrestrial ecosystems [79]. These consequences have been well documented for some streams in New Zealand where stocking brown trout has restricted many native populations of the Galaxiidae family to sections not colonised by trout, for example upstream of waterfalls [80]. Brown trout are far more effective predators than native Galaxiids: they consume near 100% of the invertebrate production of the streams versus less than 20% for Galaxiids. As a consequence, invertebrates are less abundant in brown trout streams, and consume a lower proportion of the aquatic primary production. This results in benthic primary production by diatoms and algae six times greater in brown trout than in Galaxiid streams. Changes in nitrogen cycling accompany these modifications in trophic relationships [81]. Similarly, arctic char (Salvelinus alpinus) introduced in a headwater stream in Montana can compete with native species for foraging microhabitats [82] and, in addition, reduce the emergence of aquatic insects eaten by riparian terrestrial invertebrates and birds [83,84], consequently decreasing the flux of carbon from stream to riparian ecosystems [85]. Nonnative fish introductions have also been implicated with the decline of amphibians within protected areas [86].
There is a need to better understand the effects of stocking predators on ecosystem dynamics in headwater streams [79]. Such an understanding would help conservation biologists facing the invasion versus isolation trade-off: opposing barriers to upstream movements of nonnative populations may protect native populations upstream, but isolate them, and thus increase the risk of local extinction [87]. Decisions need to be articulated and discussed, particularly for native trout populations which are threatened worldwide and increasingly relegated to headwater streams refuges. In this regard, Faush et al. [87] suggest focusing on four main questions: Are populations of conservation value? Are they vulnerable to invasion and displacement by nonnative salmonids? Would they be threatened with local extinction if isolated with barriers? How should management be prioritized among multiple populations? Araguas et al. [76] propose similar guidelines to improve management based on genetic refuges, and focus on habitat improvement and recovery, as well as on social attitudes favouring reduced exploitation to levels that can be sustained by natural reproduction.
On the whole, we know very little about ecological impacts associated with fish introductions, and some authors [88] recommend postponing intentional introduction of nonnative species in river networks, as long as we do not understand their effects on both the abundance and distribution of native species. For the Columbia River Basin, the Independent Scientific Advisory Board recommends to elevate the issue of nonnative species effects to a priority equivalent to that of habitat loss and degradation, climate change, and human population growth and development [89]. Among the research needs, the Advisory Board points to the vulnerability of the landscape to nonnative species spread, the impacts of nonnative on native salmonids and other native species (predation, transmission of diseases and parasites, competition), the potential synergistic interactions of climate change, land use, and nonnative species spread.
6 Biotic homogenization
Biotic homogenization is the process by which the genetic, taxonomic or functional similarities increase over time. Introduction of cosmopolitan species have homogenized the world freshwater fish faunas for centuries. Aquaculture and angling practices, ballast water transfers, aquarium releases, and illegal stocking are some drivers of this phenomenon which often results in the extirpation of native species. As a result, regionally distinct communities are progressively replaced by cosmopolitan ones [90], a process that is accelerating. An example is that of Mediterranean climate regions distributed across distant parts of the world. Originally, they had only a few fish species in common and were characterized by high levels of endemism. These regions are now considerably more similar as a result of species invasions, extinctions and environmental alterations [91]. Further, in a continental example, Rahel [92] documented the homogenization of fish faunas across the United States, indicating that: (1) on average, pairs of states had 15.4 more species in common in 2000 than before European settlement of North America; and (2) 89 pairs of states shared an average of 25.2 species whereas they had formerly no species in common.
Another important driver is dam operations, particularly when used for water storage. Depending on the operation regime, dams tend to damp the flows regimes of rivers, reducing high flows and elevating the levels of low flows. Dam operation also changes the timing and duration of extreme flows. This results in less complex channels, with less diverse habitats available for fish and other aquatic organisms. In addition, levees along rivers disconnect main channels from their floodplains thereby reducing channel migration, development of riparian systems and functional connections with neighbouring terrestrial ecosystems – key properties along every river network in the world. Several authors [93,94] suggest that the cumulative effect of river regulation established in the United States during the 20th century has erased natural flow variations induced by regional climates. Indeed, flow regime differences from one region to another in the United States during the first half of the 20th century are significantly reduced in rivers regulated by dams, and not in free flowing rivers (Box 1).
The importance of natural heterogeneity has been clearly demonstrated for sockeye salmon (Oncorhynchus nerka) in river networks of Bristol Bay, Alaska [105]. Temperature, depth, plant cover and meteorological events vary enormously in these large river networks, among habitats and among years. As a consequence, salmon populations fluctuate differently and independently from one river subnetwork to the other, prospering in some habitats one year, and in other habitats another year. This lessens the interannual variability of the entire stock of sockeye salmon at the scale of Bristol Bay and, at the mouth of river networks, stabilizes commercial fisheries exploiting the hundreds of populations that are differentially adapted to specific tributaries and lakes upstream. In addition, age class heterogeneity in sockeye salmon further reduces the probability of all individuals of a same cohort encountering unfavourable environmental conditions during their run upstream.
7 River networks in a changing climate
Climate change is expected to greatly modify water and biogeochemical cycles along rivers. Theoretically, higher atmospheric CO2 concentrations induce plant stomata to remain closed more frequently and less water vapour to be lost by plant evapotranspiration. As a consequence, photosynthesis occurs with less water uptake from the soil, potentially increasing runoff. This process may be enough to explain why more freshwater has been delivered by rivers to oceans for about a century [106]. With more freshwater, higher total annual loads of dissolved nitrogen and phosphorus are transported from continental areas to sea, particularly in rivers flowing across regions where permafrost is susceptible to thaw. Some scenarios predict nitrogen and phosphorus levels transported to the Kara Sea and adjacent Arctic Ocean by the rivers Ob and Yenissei to increase by 30 to 50% by 2100 [107].
Climate change is also expected to affect downstream hydrology of river basins fed by mountain snow and glaciers. The consequences will affect more than one-sixth of the Earth's population living in regions including parts of northern China, north western India, areas south of the Hindu Kush, subbasins downstream of the southern Andes, north central United States of America, and some coastal areas of western North America and Europe [108]. Glacier retreat now occurs in most mountainous regions, particularly in the Himalaya-Hindu Kush region, the third largest glacial area on earth, and also in the South American Andes. Both regions are heading for a water supply crisis which will require the development of new water management techniques and storage capacity, as well as significant changes to agriculture, industry and lifestyle [108].
In this respect, the Tibetan plateau and adjacent mountain ranges–often called the third pole of climate change–are a water tower for the Indus, Ganges, Brahmaputra, Yangtze and Yellow Asian rivers – home to more than 1.4 billion people. The contribution of melt water to annual runoff varies between basins: extremely important for the Indus, important for the Brahmaputra, modest for the Ganges, Yangtze and Yellow rivers [109]. As a consequence, climate change is predicted to severely affect water availability and food security in the Indus and Brahmaputra basins where 60 million people largely depend on irrigated agriculture and melt water. The impact may be less severe or even seasonally positive in other Asian river basins. However, the impact of climate change on water resources and agriculture is difficult to assess in a large country such as China [110]. For example, runoff in both the Yellow and the Yangtze rivers is influenced by the retreat of glaciers in western China, but runoff is characterized by drying trends and intense withdrawal by humans in the Yellow River, and by frequent floods caused by monsoon rains in the Yangtze River. Clearly such differences need to be included in scenarios of future water resources as well as in adaptation strategies such as improved agricultural practice, reforestation programmes, flood control, drought alleviation and re-establishing healthier river ecosystems [110].
On a global scale, combined changes in climate and water consumption are expected to drive up to 75% of local fish species toward extinction in some rivers by 2070. This process will have harmful consequences particularly in poor countries which often rely on local fish for food [111]. On a regional scale, conditions predicted for twenty-first century climate change would variously affect the distribution range of the 28 diadromous fish species living in Europe, North Africa and the Middle East. More specifically, there would be little or no effect for five species, distribution range expansion for three, and distribution range contraction, i.e. lost of suitable basins, for fourteen [63]. Managed relocation may be a useful conservation strategy to increase the probability of future persistence of individuals unable to move quickly enough to adapt their distribution range [112,113]. However, unintended extirpation of local species may occur, as well as invasions, in the highly connected river networks thereby leading some authors to recommend against managed relocation [114,115]. At a minimum, ecological and life-history traits of candidate species for managed translocation should be considered first, and translocations should occur within the species’ historical range, optimally within the same major river network [113].
Another conservation strategy under climatic change is to use rivers and their adjacent riparian areas as linear corridors in a landscape [116]. These corridors strengthen landscape connectivity, facilitating species movement for aquatic but also for terrestrial species such as birds [117], linking aquatic and terrestrial food webs [83,85,118], and allowing semi-aquatic species such as amphibians to complete their life cycle [119]. In addition, river networks and corridors are natural routes for displacement of aquatic and terrestrial species when habitats become unsuitable under new climatic conditions. However, these natural routes are also used by exotic species to colonize freshwater habitats, particularly those already modified or degraded [120–122], with deleterious consequences. Well known examples are crayfish plague in Europe, salmonid declines in Southern Hemisphere lakes and streams [112], or alteration of flow regimes due to Tamarix invasions in Australia and North America [123]. Therefore, developing connections of fragmented habitats through river corridors must be based on in-depth knowledge of the behaviour, preferences and life history of the aquatic and riparian species involved, and the dynamics of their habitats. Moreover, it requires consultation and consistency between urban, agricultural and ecological policies, and progress in understanding the dynamics of socioecological systems [124].
8 Towards human well being along rivers
On October 4, 2010, the toxic spill from a Hungarian aluminium oxide factory contaminated thousands of hectares with 600,000 to 1 million m3 of red toxic sludge, entering the Torna and Marcal tributaries of the Danube River [125]. Traces were detected in the waters of the Danube delta, 1800 km downstream, illustrating the hotline character of this prestigious European river system.
Everywhere in the world, river networks transport thousands of synthetic and natural contaminants at low to very low concentrations. Such micropollutants may be heavy metals, organic pollutants, but also hormones, drugs, and various persistent degradation products. They represent an increasing toxicological issue in industrialized as well as in many other areas [126–128]: their distribution, bioavailability, and effects on humans and aquatic systems are difficult to assess, and methods to minimize their introduction to rivers need improvements. At the same time, designing less harmful industrial processes and products is an imperative for human health along rivers. Addressing such issues is a real scientific challenge, requiring cooperation between environmental chemists, engineers, toxicologists, ecologists, and the involvement of all interested stakeholders [128].
Water related infectious diseases raise another great concern along rivers, needing dauntless international programs, particularly in developing countries [129]. The latest WHO/UNICEF report [130] reminds us that over 2.6 billion people were living without access to improved sanitation facilities, and nearly 900 million people were not receiving their drinking-water from improved water sources in 2008. Diarrhoea appears as the second leading contributor to global burden of disease, after lower respiratory infections but ahead of heart disease and HIV/AIDS. Diarrhoea kills 1.5 million children annually, as a consequence of unsafe water, inadequate sanitation and insufficient hygiene. The problems have been known for a long time, and many organisational reasons explain such a situation. A possible answer is “targeting resources for better results” as suggested by the title of a recent UN-GLASS report [131].
Among diseases directly or indirectly linked to rivers, malaria remains a public health problem in over 100 countries worldwide, inhabited by some 40% of the world population. World Health Organization recommended these countries to supply all persons at risk insecticide-treated mosquito nets, establish a program of indoor residual spraying, provide laboratory based diagnosis for all suspected cases, and provide all confirmed cases effective treatment. Such efforts have reduced the estimated number of deaths from nearly 1 million in 2000 to 781,000 in 2009 [132]. However, the progress in malaria control is fragile, and control programmes need to be rigorously maintained once introduced. Other parasitic diseases are also of concern along rivers, such as schistosomiasis (bilharzia), lymphatic filariasis, onchocerciasis (river blindness)… Progress in these areas is essential to meet the targets of the UN Millennium Development Goal [133].
In addition, several examples suggest that biodiversity loss can increase infectious transmissions, as shown for West Nile encephalitis in the United States–an illness due to a virus transmitted by mosquito and having several species of passerine birds as hosts [134,135], and for schistosomiasis–a parasitic illness caused by a trematode that alternately infects aquatic snails and humans [136]. Although the generality and mechanisms involved need further study, preserving biodiversity seems to be a way to reduce the prevalence of infectious diseases [137], preventing insects and molluscs vectors of human parasitic infections to proliferate, and transmission conditions to develop [32].
9 Conclusion
In their natural state, rivers are linearly connected ecosystems. They are organised as complex, fluctuating networks, open to seas and oceans. In addition, their structure and function depend on the quality of their surrounding terrestrial environment in their respective drainage basins. The connectedness of river ecosystems is responsive to global climatic variations and hydrological hazards, to the point that river runoff has been suggested as a monitoring tool for responses of terrestrial and marine ecosystems to climate change [138]. At whatever scale–river section, drainage basin or the planet Earth–the upstream-downstream connection makes rivers hotlines for biodiversity.
At the river section scale, the sustainability of running water ecosystems requires naturally fluctuating flows. Recurring floods are important reset events for running water ecosystems, with regard to sediment and nutrient redistribution, as well as for aquatic and riparian biodiversity [100]. In the absence of such resets, for example when dam operations restrict major floods, exotic species may establish and proliferate [139]. However, we still need to improve our ability to set limits to acceptable fluctuations. Extreme hydrological events–millennium floods and prolonged droughts–may promote insecure conditions for humans as well as for biodiversity along river networks. We also need better knowledge to restore river sections on the basis of historical references. Firstly, we cannot think of returning to rivers obstructed with debris wood and log jams as before the development of navigation. Secondly, we hardly realize how important the anthropogenic transformation of river networks may have been in space and time: for example, only in 2008 were the implications for stream restoration caused by tens of thousands of water mills operating in Europe and the United States during the 17th–19th centuries recognized [140]. Beyond historical references, it must be understood that realistic restoration goals are not necessarily “analogue” futures of what have existed in the past [102]; they require managing “hybrid” or “novel” systems created by humans in an ever-changing world [53].
Conservation measures at the drainage basin scale are also needed to maintain the biodiversity of river networks. Conservation measures at that scale take into account the ghost of land use past [141], and meet ecological and societal needs for freshwater, as well as the ways they interact [139]. Clearly, integrated management of water resources at the scale of drainage basins is required to regularise possible future water shortages, undesirable environmental impacts, and inequitable sharing of water resources [142,143]. It is also a prerequisite to a sustainable use of water resources in the context of scarcity. Enormous additional water demand is expected during the next decades with an increasing human population, requiring better management of soils and water at the drainage basin scale [144], as well as better understanding of how climatic variability affects surface and subsurface hydrology and how local societies may adapt to water scarcity [145].
At the global scale, responding to food requirements of an expanding human population will increase the proportion of water vapour in the hydrological cycle [146]. For an unchanged water production, water required to produce food will have to double to 9660 km3 per year in 2050 [144], meaning that an additional 5200 km3 per year needs to be found, and used mainly by irrigation, at the risk of increasing ecosystem degradation and incidence of water borne illnesses. Clearly, a better knowledge of impacts of climatic variability is needed, which means efforts to collect, analyse and disseminate hydrological information around the world [145]. Dam construction may improve water security, but dams induce changes in flow regimes and habitat losses, reducing biodiversity–a costly consequence in the long term as river biodiversity contributes to nutrient cycling and fisheries sustainability, and reduces incidence of water linked illnesses [147].
The services delivered by rivers are threatened everywhere in the world, as a consequence of inadequate management of river networks and the basins they drain [148]. At the same time, these rivers are constant threats for human security through floods and water borne illnesses, and we know that this is related to the reduction of biodiversity along rivers at genetic, population and ecosystem levels [147]. Considering every river as a hotline for biodiversity, in developed, as well as in developing countries, is an integral part of global water security.
Disclosure of interest
The author declares that he has no conflicts of interest.
Acknowledgments
I thank Robert J. Naiman (University of Washington, Seattle, USA) for highly constructive and comprehensive suggestions on earlier drafts, and Eric Chauvet (CNRS, Toulouse, France), Jean-Dominique Lebreton (CNRS, Montpellier, France), and Eric Tabacchi (CNRS, Toulouse, France) for useful discussions and comments.
1 Defined as areas characterised by “exceptional concentrations of species with high levels of endemism, and experiencing unusually rapid rates of depletion” [1].