1 Introduction
The Hymenophyllaceae, or filmy ferns or “filmies”, are the most speciose (more than 600 species) and diverse family of basal leptosporangiate ferns in terms of morphology and ecology. This is especially true for the trichomanoids, one of this family's two major clades comprising ca. 300 [1,2] terrestrial, climbing, saxicolous and epiphytic species [3,4] (Fig. 1). Filmy ferns are distributed pantropically (with a few exceptions in temperate areas) in the wettest places, and are generally confined to rainforests. They are characterized by a single-cell thick lamina (hence the English vernacular name), which lacks cuticle, differentiated epidermis and stomata. Since no barrier exists to regulate loss of water, these ferns depend upon high levels of environmental moisture and are strict hygrophytes (i.e., a high level of atmospheric hygrometry is required for their growth). Filmy ferns are thus a pertinent and original representative group to investigate adaptive strategies related to hygrophily in ferns and in vascular plants in general.
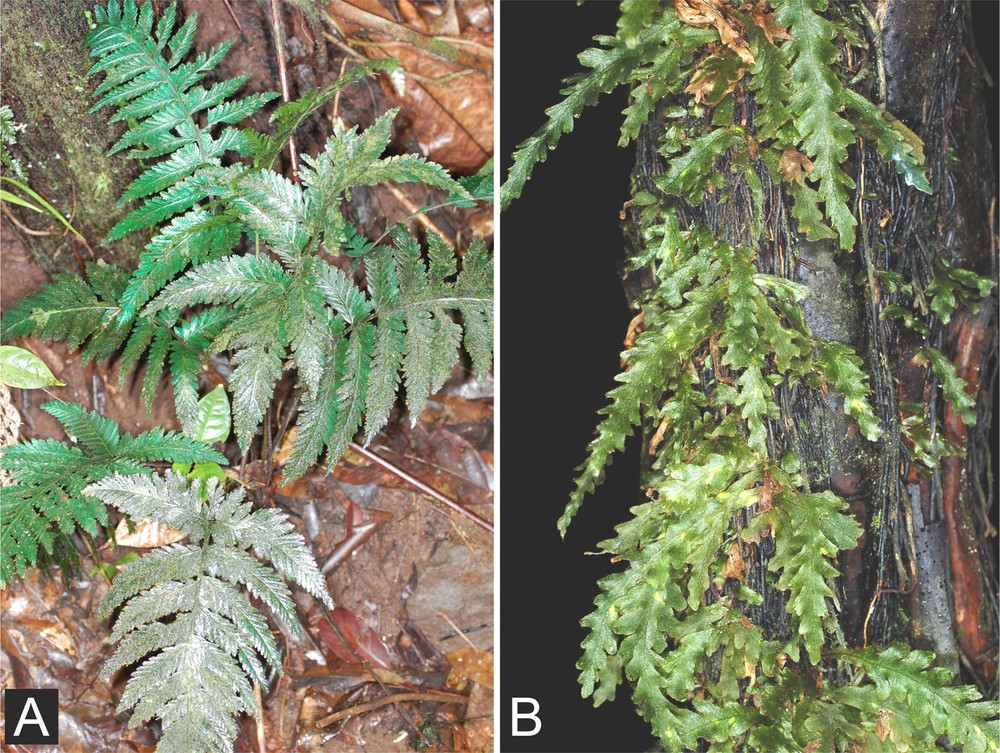
Two trichomanoid species illustrating contrasting ecologies and growth-forms. A. Trichomanes elegans, a large terrestrial species with clustered erect fronds on a short thick monocaulous rhizome. Fronds can exceed 50 cm in length (Basse Terre, La Guadeloupe). B. Trichomanes polypodioides, an epiphytic species of medium size (fronds rarely exceed 15 cm in length) colonizing a tree fern trunk with its branching creeping rhizome (Basse Terre, La Guadeloupe).
Several studies have previously addressed the ecology of the Hymenophyllaceae, and especially of the trichomanoids, in an evolutionary context. For epiphytic trichomanoids, a regressive evolution in morphology was proposed [4,5]. This regressive evolution illustrates tendencies toward a “bryophyte-like” strategy so named because numerous epiphytic trichomanoid species resemble bryophytes. In addition, they often live sympatrically with epiphytic leafy liverworts and mosses on tree trunks and branches. However, these studies were performed using limited taxonomic samplings. In a more recent study, regressive evolution for the stele was also proposed, but this data-set was incomplete and the scenarios inferred were thus strongly extrapolated [6]. Consequently, although preliminary assumptions have been reviewed previously [7], a complete analysis of all available data (i.e., ecological diversity, evolution of morphology and anatomy, including molecular dating to estimate the age of diversifications) is still lacking. Furthermore, the adaptive strategies previously proposed have never been statistically validated using a phylogenetic comparative method (as defined by Felsenstein [8], and formalized by Harvey and Pagel [9] and Martins [10]). Phylogenetic comparisons are useful and required to evidence statistically valid correlations among the traits in a taxonomic group. The objects studied here (i.e., taxa) are indeed not independent, since they are evolutionarily linked, and standard predictive tests are thus not appropriate. In other words, a correlation revealed by a standard test could reflect a common inheritance rather than a true biological (or ecological) relationship. Comparative methods, on the other hand, allow statistical tests to be applied to taxonomical objects with a phylogenetic correction.
The aim of this study is therefore to analyze the evolution of trichomanoid anatomy, relying on an expanded molecular phylogeny representing morphological, ecological and geographical variability in the lineage, as well as on adequate statistical tests. To this end, we first completed the morphological and especially the anatomical data-set by acquiring new qualitative and quantitative data. We then statistically tested potential relationships among selected characters and growth-forms, with the aim of proposing hypotheses on potential adaptive anatomical strategies. This descriptive and analytical study focusing on anatomy and growth-forms thus completes an essential intermediate step that will enable further evolutionary analyses addressing trichomanoid habitats and precise ecological preferences.
2 Material and methods
2.1 Taxonomic sampling
We focus here on the trichomanoid lineage, which exhibits the highest levels of diversity in terms of morphology and ecology in the Hymenophyllaceae. We selected 50 trichomanoid species representative of the morphological, ecological and presumed anatomical variability of the lineage and its global geographical distribution. This sampling included representatives for each genus and subgenus of the lineage according to the most recently published classification and phylogeny [2,6] and was determined in part by accessibility to material available for destructive anatomical investigation (see below). Selected species are reported in Table 1 along with their ecology and geographical distribution. Vouchers of specimens selected for anatomical investigation are available on request.
List of selected taxa with geographical distribution, ecology, qualitative and qualitative characters of interest for growth-form and anatomy (see text).
Taxon | Subgenus | Distribution | Ecology | Stem branching (= coloniality) | Stele type | Cortex | Blade length (cm) | Stem thickness (μm) | Stele diameter (μm) |
Abrodictyum caudatum (Brack.) Ebihara & K. Iwats. | Abrodictyum | P | EC | N | M | Ho | 23.00 | 1349.15 | 549.94 |
Abrodictyum elongatum (A.Cunn.) Ebihara & K. Iwats. | Pachychaetum | P | T | N | M | Ho | 25.00 | 1696.06 | 389.46 |
Abrodictyum flavofuscum (Bosch) Ebihara & K. Iwats. | Abrodictyum | P | EC | N | M | Ho | 25.00 | 2055.41 | 1066.94 |
Abrodictyum meifolium (Bory ex Willd.) Ebihara & K. Iwats. | Pachychaetum | IO | T | N | M | Ho | 25.00 | 1504.78 | 416.72 |
Abrodictyum obscurum (Blume) Ebihara & K. Iwats. | Pachychaetum | A | T | N | M | Ho | 20.00 | 1548.36 | 407.09 |
Abrodictyum pluma (Hook.) Ebihara & K. Iwats. | Abrodictyum | A | T | N | M | Ho | 18.00 | 931.85 | 267.84 |
Abrodictyum rigidum (Sw.) Ebihara & Dubuisson | Pachychaetum | NT | T | N | M | Ho | 23.00 | 2483.55 | 669.88 |
Abrodictyum strictum (Menzies ex Hook. & Grev.) Ebihara & K. Iwats. | Abrodictyum | P | T | N | M | Ho | 19.00 | 1530.19 | 330.01 |
Abrodictyum tamarisciforme (Jacq.) Ebihara & Dubuisson | Pachychaetum | IO | IE | N | M | Ho | 30.00 | 2352.97 | 754.55 |
Callistopteris apiifolia (C. Presl) Copel. | – | A | T | N | M | Ho | 40.00 | 3252.50 | 790.36 |
Cephalomanes javanicum (Blume) C. Presl | – | A | T | N | M | Ho | 20.00 | 1158.04 | 257.67 |
Crepidomanes bipunctatum (Poir.) Copel. | Crepidomanes | IO + A | CE | Y | SC | Ho | 4.00 | 704.76 | 83.93 |
Crepidomanes cf. bonapartei (C. Chr.) J.P.Roux | Crepidomanes | IO | CE | Y | SC to Rg | He | 2.00 | 382.87 | 77.79 |
Crepidomanes fallax (H. Christ) Ebihara & Dubuisson | Crepidomanes | AF + IO | CE | Y | SC to Rg | He | 10.00 | 462.22 | 53.98 |
Crepidomanes latealatum (Bosch) Copel. | Crepidomanes | A | CE | Y | SC | He | 4.50 | 636.89 | 111.70 |
Crepidomanes minutum (Blume) K. Iwats. | Crepidomanes | PT | CE | Y | Rg* | Pl | 3.00 | 273.63 | 20.53 |
Crepidomanes aphlebioides (H. Christ) I.M. Turner | Nesopteris | A | EC | Y | M | He | 60.00 | 2886.78 | 1554.18 |
Crepidomanes intermedium (Bosch) Ebihara & K. Iwats. | Nesopteris | A | T | N | M | He | 37.00 | 1504.16 | 411.45 |
Crepidomanes thysanostomum (Makino) Ebihara & K. Iwats. | Nesopteris | A | T | N | M | He | 25.00 | 919.43 | 161.81 |
Didymoglossum cuspidatum (Willd.) Ebihara & Dubuisson | Microgonium | IO | CE | Y | Rg** | Pl | 1.75 | 309.96 | 59.70 |
Didymoglossum ekmanii (Wess. Boer) Ebihara & Dubuisson | Microgonium | NT | CE | Y | Rg** | Pl | 2.25 | 333.43 | 82.72 |
Didymoglossum exiguum (Bedd.) Copel. | Didymoglossum | A | CE | Y | Rg** | Pl | 0.75 | 217.21 | 18.74 |
Didymoglossum gourlianum (Grev. Ex J. Sm.) Pic. Serm. | Didymoglossum | NT | CE | Y | Rg** | Pl | 8.00 | 642.45 | 77.38 |
Didymoglossum hildebrandtii (Kuhn) Ebihara & Dubuisson | Didymoglossum | IO | CE | Y | C | Pl | 2.50 | 559.73 | 133.24 |
Didymoglossum krausii (Hook. & Grev.) C. Presl | Didymoglossum | NT | CE | Y | Rg** | Pl | 4.00 | 218.84 | 27.17 |
Didymoglossum membraceum (L.) Vareschi | Didymoglossum | NT | CE | Y | C-Rg | Pl | 4.00 | 506.37 | 84.00 |
Polyphlebium borbonicum (Bosch) Ebihara & Dubuisson | – | AF + IO | CE | Y | SC | He | 10.00 | 328.22 | 87.73 |
Polyphlebium capillaceum (L.) Ebihara & Dubuisson | – | NT | CE | Y | SC | He | 14.00 | 378.16 | 94.77 |
Polyphlebium endlicherianum (C. Presl) Ebihara & K. Iwats. | – | P | CE | Y | Rg* | Pl | 7.50 | 329.95 | 35.24 |
Polyphlebium hymenophylloides (Bosch) Ebihara & Dubuisson | – | NT | CE | Y | SC to Rg | Pl | 7.80 | 329.07 | 62.71 |
Polyphlebium venosum (R. Br.) Copel. | – | P | CE | Y | Rg | He | 7.00 | 281.92 | 35.76 |
Trichomanes alatum Sw. | Trichomanes | NT | IE | N | M | Pl | 20.00 | 1638.10 | 330.25 |
Trichomanes arbuscula Desv. | Trichomanes | NT | T | N | M | Ho | 9.00 | 928.06 | 246.22 |
Trichomanes crispum L. | Trichomanes | NT | IE | N | M | Ho | 18.00 | 2197.16 | 640.54 |
Trichomanes holopterum Kunze | Trichomanes | NT | T | N | M | Ho | 6.00 | 1582.01 | 516.36 |
Trichomanes osmundoides Poir. | Feea | NT | T | N | M | Ho | 16.00 | 1344.84 | 492.08 |
Trichomanes pinnatum Hedw. | Trichomanes | NT | T | N | M | Ho | 45.00 | 2739.30 | 1583.58 |
Trichomanes polypodioides L. | Trichomanes | NT | CE | Y | M | Ho | 12.00 | 504.46 | 159.62 |
Trichomanes robustum E. Fourn. | Trichomanes | NT | T | N | M | Pl | 45.00 | 997.17 | 402.38 |
Trichomanes scandens L. | Trichomanes | NT | EC | Y | M | Ho | 35.00 | 1461.01 | 646.92 |
Trichomanes trigonum Desv. | Trichomanes | NT | T | N | M | Ho | 30.00 | 1485.37 | 551.46 |
Trichomanes elegans Rich. | Davalliopsis | NT | T | N | M | Ho | 50.00 | 3480.62 | 1204.08 |
Trichomanes ankersii C. Parker ex Hook. | Lacostea | NT | L | Y | M | Ho | 8.50 | 839.99 | 422.54 |
Trichomanes pedicellatum Desv. | Lacostea | NT | L | Y | M | Ho | 8.50 | 1205.36 | 655.05 |
Vandenboschia davallioides (Gaudich.) Copel. | Vandenboschia | Hawaï | EC | Y | Re | Ho | 40.00 | 2598.14 | 1361.98 |
Vandenboschia gigantea (Bory ex Willd.) Ebihara & Dubuisson | Vandenboschia | IO | HE | Y | Re | He | 50.00 | 2153.30 | 880.01 |
Vandenboschia maxima (Blume) Copel. | Vandenboschia | A | T | N | M | He | 50.00 | 1489.50 | 368.07 |
Vandenboschia radicans (Sw.) Copel. | Vandenboschia | NT | EC | Y | Re | Ho | 40.00 | 1600.41 | 737.83 |
Vandenboschia rupestris (Raddi) Ebihara & K. Iwats. | Lacosteopsis | NT | EC | Y | Re | He | 15.00 | 848.22 | 337.46 |
Vandenboschia speciosa (Willd.) G. Kunkel | Vandenboschia | E | EC | Y | Re | He | 20.00 | 1378.21 | 546.97 |
2.2 Stem anatomy investigation
Anatomical sections were performed for all species in the sampling. Some have been previously documented for anatomy [3,6,11–16], but were selected in order to check and correct errors or misinterpretations, if necessary, and to acquire new quantitative data. In parallel, we sectioned numerous other species not included in our sampling, and characterized their anatomy to better assess the homogeneity within the trichomanoid clades.
Mature rhizomes of adult specimens were sectioned using a standard procedure. Samples were stored in 70% alcohol and were rehydrated for 10 minutes in distilled water before cutting. When the material was difficult to cut, samples were further softened by soaking overnight in water. Freehand transverse sections were obtained and immersed in 50% (for the largest sections) or 10 to 20% (for the smallest sections) sodium hypochlorite to eliminate cellular organelles. After washing with distilled water, mordanting fixation was performed in 1% acetic acid for 1 hour. Sections were then immersed in a mixture of carmine alun (6% p/v) and iodine green (0.01% v/v) (Mirande's reagent [17]) for 4 to 12 hours. This reagent was used to distinguish between cellulosic (stained pink) and lignified tissues (e.g., lignified xylem, sclerenchyma and suberified tissues; stained blue-green). The sections were then washed in distilled water for five minutes and dehydrated with a series of ethanol-water washes of 75%, 95% and 100% ethanol for 15 minutes each. After three washes in toluene, sections were mounted in Therebinthina canadensis balsam and covered with cover slips. Whole samples were examined using a Zeiss stereomicroscope (http://www.zeiss.com), and images were captured using a Zeiss AxioCam camera.
2.3 Quantitative measures
Ideally, numerous intra- and interindividual measurements are required for each species in order to obtain statistically representative data that take into account natural variability. Filmy ferns are problematic because many species inhabit endangered habitats and are therefore rare. This hinders the collection of numerous specimens from several locations, and consequently limits their availability for destructive investigations. Herbarium collections are useful in this context because they often provide numerous specimens of various origins, which can be used in anatomical studies. Consequently, most of our selected material came from herbarium specimens, and especially from the collections of the Muséum national d’histoire naturelle, Paris. Another impact on anatomical investigation concerns the growth-form. As described hereafter, colonial species display long branched rhizomes, providing several parts for sectioning which will not be prejudicial to the specimens. In the case of monocaulous species, stem sections are destructive and specimens are lost (this is quite problematic with rare and precious herbarium specimens). Furthermore, we can expect higher variability in a specimen with long-creeping rhizomes (several centimetres long) than in a specimen with short rhizomes (not exceeding 1–2 cm). It was therefore not possible to apply the same standard procedure for all species. In this study, we applied the following procedure, which takes into account the rarity and fragility of the material. For each species studied, whenever possible, at least three specimens collected from different locations were selected and ten sections were made per specimen, each at a different position on the stem. In the case of rare, short-monocaulous species, ten sections were generally made on the single short rhizome of a single specimen.
Measurements were made automatically on digital images using Axiovision Release 4.8.1. software (provided by Zeiss). These measurements were made on all sections performed for the stem thickness and the stele diameter. The mean of all measured data was calculated for each species and each trait. Due to heterogeneity in the data acquisition procedure, intraspecific variability was not taken into account. Furthermore, this variability was not integrated in the selected phylogenetic comparative methods (see hereafter). We thus assume that the data (means) used here are at least representative of the taxon and useful for inter-specific comparative studies, but do not reflect true and natural variability. To test potential correlations between plant size and stem thickness, we also recorded the blade length as the global mean of measures taken for the three largest leaves of all specimens (or for the single specimen), or extracted them from a previous study [4]. Correlations among selected characters were graphically evaluated using a standard regression procedure to evidence potential clustering. This approach enabled us to categorize quantitative characters and to formulate hypothetical correlations, which were then phylogenetically tested.
2.4 Phylogenetic approaches and inferred evolution of selected characters
We refer here to the most recently published phylogeny [6], to which we made two modifications. First, we used a representative sub-sampling, due to the limited availability of material for anatomical investigation; and second, we included a previously un-sampled species, Crepidomanes cf. bonapartei (C. Chr.) J.P. Roux, collected on the island of La Réunion (voucher J.-Y. Dubuisson HR2002-2, Genbank accession number AB608733). Molecular data acquisition protocol, vouchers and sequence (rbcL) accession numbers for all other taxa were published previously [6]. We reconstructed the phylogenetic relationships among the trichomanoids based on rbcL sequences, including the newly sequenced species, and by using a Bayesian inference as described previously [6]. We used five hymenophylloid species as out-group, selected among the basal clades of the lineage [18]: Hymenophyllum pulcherrimum Colenso, H. fuciforme Sw., H. pallidum (Blume) Ebihara & K. Iwats, H. dilatatum (G. Forst.) Sw. and H. nephrophyllum Ebihara & K. Iwats. We compiled the 50% majority rule of all trees retained by the Bayesian analysis, and used this tree in subsequent analyses after pruning the out-groups to avoid methodological bias. Indeed, all hymenophylloids display the same gross morphology and are epiphytic [2,19,20]. In addition, the genus Hymenophyllum is in a clade considered to be quite derived and its diversification is estimated to have occurred in the upper Cretaceous, whereas the trichomanoids are thought to have diversified mainly in the Jurassic [21]. By restricting our study to trichomanoids, we were ensured of having potential plesiomorphic (ancestral) and apomorphic (derived) states with which to infer the evolution of selected characters.
We reconstructed the evolutions of the stele and cortex types proposed hereafter, and of growth-forms. We propose two different growth-form types. Colonial growth is observed in species displaying long-creeping and highly branched rhizomes enabling individuals to colonize a wide surface of the substrate. Individual growth is observed in species displaying a monocaulous short-to-long creeping rhizome or a creeping rhizome with little or no branching. This latter growth-form does not enable individuals to colonize a wide surface. We also inferred the evolution of quantitative characters (i.e., stem thickness and stele diameter), whenever we could categorize them, as subsequent methods required discrete data. The categorization of these data is discussed in “Results”.
The evolution of selected characters was inferred on the consensus phylogeny (including branch lengths) using the maximum likelihood (ML) method [22,23] as implemented in the Mesquite package ver. 2.73 [24]. We tested two models implemented in Mesquite. The first (MK1 for “Markov K-state 1 parameter model”) assumes equivalent probabilities for forward (apomorphic) and backward (reversal) changes for a single character. The second (AsymmMK for “Asymmetrical Markov K-state 2 parameter model”) attributes distinct probabilities for reversal and apomorphic changes. This difference in change rates appears more realistic, especially in the case of regressive evolution when regression and loss of characters are often irreversible (the irreversibility constraint is particular to the AsymmMK model when the backward rate is constrained to be very low to negligible). However, the AsymmMK model can only be selected for binary characters. For both models, rates of changes are directly estimated based on the data. We tested correlations between characters on the phylogeny (according to the phylogenetic comparative methods [23,25,26]) by using the Pagel and Meade's method [27] on discrete data implemented in their BayesTraits package (available from www.evolution.rdg.ac.uk). For each pair of characters tested, the program calculates the likelihood of the distribution of character states expected on the trees under the assumption that both characters are dependent (or evolutionarily correlated) and the likelihood of the expected distribution under the assumption that both characters are independent (or not evolutionarily correlated). We used the ML method implemented in the BayesTraits package. The analyses were performed on a random sampling of 100 trees among the trees produced by the Bayesian analysis used to reconstruct the phylogeny. The resulting likelihoods were compared using the likelihood ratio test statistic (LR) [28], where LR = 2 × (log-likelihood (dependent model)–log-likelihood (independent model)) is distributed as a χ2 with four degrees of freedom. A significant χ2 value proposes that the evolutionary correlation between the two characters is statistically significant. The LR used here corresponds to the mean of the LR provided for each of the 100 sampled trees.
3 Results
3.1 Anatomical diversity
We report and describe below the distinct stele and cortex types observed in our sampling, which are also illustrated on Fig. 2.

Representative stem anatomy types observed among trichomanoids. A. Trichomanes trigonum exhibiting a massive stele and a homogeneous cortex. The arrow shows the limit of the cortex, scale bar = 500 μm. B. Crepidomanes thysanostomum, detail of massive stele with exarch differentiation in a young stem, scale bar = 50 μm. C. Vandenboschia speciosa, reduced stele with heterogeneous cortex, the arrow shows the limit between the inner cortex (supposed true cortex) and the outer cortex (supposed hypertrophied hypodermis), scale bar = 200 μm. D. Trichomanes scandens, massive stele with heterogeneous cortex, the white arrow shows the limit between the inner cortex (supposed true cortex) and the outer cortex (presumed hypertrophied hypodermis), scale bar = 100 μm. E. Didymoglossum gourlianum, collateral stele with petiole-like cortex, the arrow shows the ventrally interrupted phloem, scale bar = 50 μm. F. Crepidomanes cf. bonapartei, sub-collateral stele with heterogeneous cortex, here also corresponding to a regressed type (see text), scale bar = 100 μm. G. Polyphlebium capillaceum, sub-collateral stele with petiole-like cortex, scale bar = 100 μm. H. Vandenboschia maxima, reduced stele with homogeneous cortex, scale bar = 200 μm. I. Trichomanes polypodioides, massive stele with apparent petiole-like cortex, scale bar = 50 μm. Co: cortex; En: endoderm; Ep: epidermis; Hy: hypodermis; ICo: inner cortex; iP: internal parenchyma; Mx: metaxylem; Ph: phloem; Px: protoxylem; Tr: Trichome; X: xylem.
3.1.1 Massive protostele
A massive central xylem-core is surrounded by a ring of phloem (Fig. 2A, D and I). The xylem is more or less parenchymatous and its differentiation is clearly peripheral (or exarch) or undetermined when mature. However, the observation of young stems with a massive stele, which exhibit incompletely lignified tissues, showed that the lignification begins at the periphery, confirming the exarch status for this type of stele (Fig. 2B).
3.1.2 Reduced protostele
A large central xylem-core is surrounded by a ring of phloem but the xylemian parenchyma is centrally concentrated (Fig. 2C and H). Differentiation is mesarch with protoxylem mostly located at the periphery of central or internal parenchyma.
3.1.3 Sub-collateral protostele
Ventral xylem is absent in this structure (Fig. 2F and G). Thus, the protoxylem appears ventrally located and seems exarch. The ring of phloem is complete.
3.1.4 Collateral protostele
This structure corresponds to a sub-collateral type stele but lacks ventral phloem (the ring of phloem is thus ventrally interrupted) (Fig. 2E).
3.1.5 Regressed protostele
The xylem is reduced to very few (1 to 3–4) tracheids or is absent, corresponding primarily to a sub-collateral stele with a complete ring of phloem (Fig. 2F) or a collateral structure with a ventrally interrupted ring of phloem.
3.1.6 Cortex
Three main types of cortex are distinguishable: homogeneous, heterogeneous and petiole-like.
3.1.6.1 Homogeneous cortex
There is a unique parenchymatous cortex surrounding the stele and separating it from the epidermis (Fig. 2A and H). In mature stems, the cortex is more or less suberified and the suberification is centripetal, beginning at the periphery just below the epidermis.
3.1.6.2 Heterogeneous cortex
The cortex is divided into two parts of more or less equal thickness (Fig. 2C, D and F). The inner one resembles a standard suberified homogeneous cortex, with the upper limit of the suberification defining the limit between both parts. The external part, often referred to as the outer cortex, could thus be considered as a hypertrophied hypodermis, which is rarely suberified.
3.1.6.3 Petiole-like cortex
In some species, the stem cortex is identical to the cortex observed in petioles, which displays a strongly suberified part at the periphery just below a single-layer epidermis (Fig. 2E, G and I). This could also be considered a priori as homogeneous because a hypertrophied hypodermis is absent. But such species belong to groups exhibiting typical heterogeneous cortex with inner cortex suberified at the periphery and a more or less thick hypodermis (see Fig. 2F). For example, in the genus Didymoglossum, all studied species display a petiole-like cortex (see Table 1) except D. hildebrandtii, which has a heterogeneous cortex with a single-layer hypodermis under the epidermis. This suggests the hypothesis that the petiole-like cortex could have derived secondarily from a heterogeneous cortex by regression of the hypodermis.
3.2 Anatomy diversity and taxonomy
Table 2 summarizes the anatomy observed in recognized genera and subgenera (all species observations are reported in Table 1). Anatomy appears to be relatively homogeneous at these taxonomical levels. There are nevertheless some exceptions. In Trichomanes subgenus Trichomanes, all species display a massive stele and a homogeneous cortex, except the climbing T. scandens, which exhibits a heterogeneous cortex (Fig. 2D) and the colonial epiphytic T. polypodioides, which exhibits an apparently petiole-like cortex (see discussion later) (Fig. 2I). In the hemi-epiphytic or climbing epiphytic genus Vandenboschia, all species display a typical reduced stele and a heterogeneous cortex, except the terrestrial V. maxima, which exhibits a homogeneous cortex (Fig. 2H).
Distribution of anatomical characters among trichomanoids, in relation to taxonomy and ecology. Stele type and cortex are detailed in text.
Genus | Subgenus | Ecology | Stele type | Cortex |
Callistopteris | Terrestrials | Massive | Homogeneous | |
Cephalomanes | Terrestrials | Massive | Homogeneous | |
Abrodictyum | Mostly terrestrials, some individual epiphytes and climbing epiphytes | Massive | Homogeneous | |
Trichomanes | Trichomanes | Mostly terrestrials, some individual epiphytes, some colonial epiphytesa and one climbing hemi-epiphyteb | Massive | Homogeneous and one heterogeneous caseb |
Feea | Terrestrials | Massive | Homogeneous | |
Davalliopsis | Terrestrials | Massive | Homogeneous | |
Lacostea | Lianescent climbers | Massive | Heterogeneous | |
Polyphlebium | Colonial epiphytes | Sub-collateral to regressed | Heterogeneous to petiole-like | |
Didymoglossum | Colonial epiphytes | Collateral to regressed | Mostly petiole-like or heterogeneous with thin hypodermis | |
Crepidomanes | Crepidomanes | Colonial epiphytes | Sub-collateral to regressed | Heterogeneous to petiole-like |
Nesopteris | Terrestrials and one climbing hemi-epiphytec | Massive | Heterogeneous | |
Vandenboschia | Climbing (hemi-)epiphytes and one terrestriald | Reduced | Heterogeneous and one homogeneous cased |
a Trichomanes polypodioides and related species not selected here.
b Trichomanes scandens.
c Crepidomanes aphlebioides.
d Vandenboschia maxima.
3.3 Quantitative data
Quantitative data are reported in Table 1. With the sampling used here, blade length ranged from 0.75 cm to 60 cm (mean = 20.02 cm, SD = 15.70 cm), stem thickness from 217.21 μm to 3480.62 μm (mean = 1250.80 μm, SD = 852.83 μm) and stele diameter from 18.74 μm to 1583.58 μm (mean = 425.17 μm, SD = 398.33 μm).
Stem thickness appeared to correlate significantly with blade length (R = 0.79, ddl = 48, p < 0.001; Fig. 3A). When we distinguished among stele types, the correlation was maintained only for species with a massive stele (R = 0.67, ddl = 25, p < 0.001) and was not significant for the other species (Fig. 3A). Two clouds of points were nevertheless distinguishable: the first one clustered collateral and sub-collateral type steles, with a single exception having a massive stele (T. polypodioides); whereas the second clustered massive and reduced types. This allowed us to graphically define two new classes of stele types combining collateral with sub-collateral types, and massive with reduced types. Based on these results, we also graphically defined two classes for the stem thickness with a limit of 750 μm.
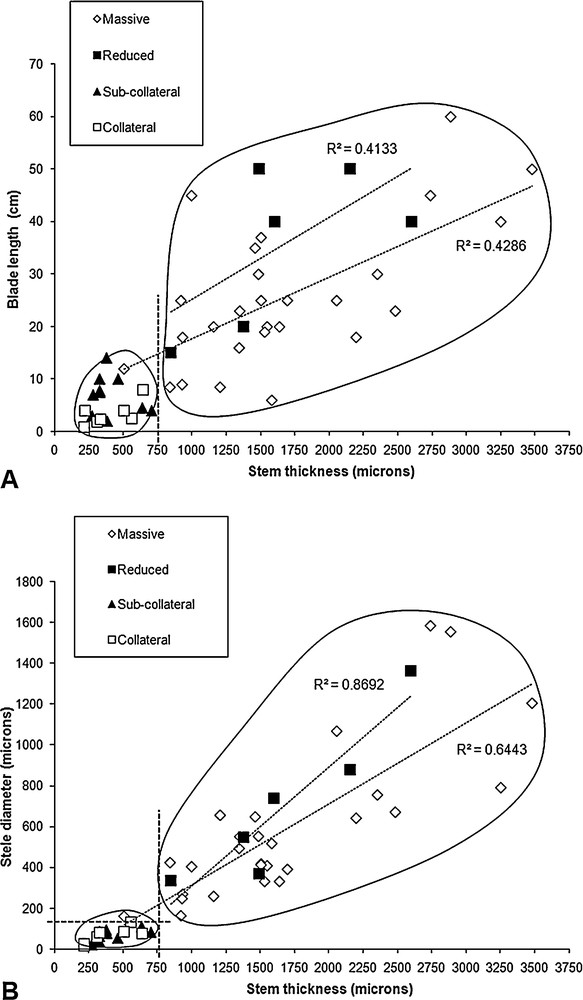
Graphical correlations between selected characters of the trichomanoids, depending on anatomy type. A. Correlation between blade length and stem thickness, according to stele type. B. Correlation between stele diameter and stem thickness, depending on stele type. Clustering and regressions are detailed and discussed in text.
Stem thickness appeared to correlate significantly with stele diameter (R = 0.79, ddl = 48, p < 0.001; Fig. 3B), but when we distinguished among stele types, the correlation was maintained only for species with massive and reduced types (respectively R = 0.80, ddl = 25, p < 0.001; R = 0.93, ddl = 4, p < 0.001). Based on these results, we confirmed the two classes for the stem thickness with a limit of 750 μm. We also defined two classes of stele diameter with an empirical limit of 150 μm, just below T. polypodioides and Crepidomanes thysanostomum, which exhibited the thinnest steles in their anatomy type (massive stele), and just above D. hildebrandtii, which exhibited the thickest stele in its anatomy type (collateral stele). After anatomy type was separated into two classes, massive-reduced and sub-collateral-collateral, the graphical analysis suggested two putative evolutionary correlations between anatomy type and quantitative data. These were: sub-collateral and collateral stele with thin stem (smaller than 750 μm); and sub-collateral and collateral stele with small stele (smaller than 150 μm). No categorizations were possible for blade length, which prevented further tests of phylogenetic correlation with the data used in this study.
3.4 Inferred evolution of anatomy and growth-forms and comparative phylogenetic analyses
The phylogeny inferred from the Bayesian approach was similar to the one obtained from a previous phylogenetic analysis using a broader taxonomic sampling and maximum parsimony (MP) [6]. However, the positions of the Callistopteris and Cephalomanes genera in our tree differed from those of the tree obtained using a Bayesian approach in the previous study. These two genera form a sister clade to all other trichomanoids in our Bayesian analyses and in previous MP analyses [6], whereas they form a sister clade to Abrodictyum + Trichomanes in the Bayesian analysis performed with a broader sampling [6]. However, relationships among these taxa were not statistically supported for any of these analyses. Therefore, we relied on the topology most frequently obtained (i.e., that inferred in this study and shown on Fig. 4). The ancestral state probabilities for anatomy and growth-form for selected nodes are reported in the Appendix.

Comparative evolution of characters in trichomanoids inferred using maximum likelihood. A. Comparative evolution of stele type and stem thickness (MK1 model), small stele corresponds to diameter less than 750 μm (see text). B. Comparative evolution of cortex type and growth-form (colonial or individual growth) (MK1 model). On both figures, the coloration of internal nodes is proportional to change probabilities (see Appendix). The statistical test result is reported with the LR value and the probability suggesting a significant correlation between compared characters (see text). On nodes, abbreviations correspond to representative clades as also reported in the Appendix: Ca: genus Callistopteris; Ce: genus Cephalomanes; Ab: genus Abrodictyum; Tr: genus Trichomanes; D: Trichomanes subgenus Davalliopsis; L: Trichomanes subgenus Lacostea; Po: genus Polyphlebium; Di: genus Didymoglossum; Cr: genus Crepidomanes; C: Crepidomanes subgenus Crepidomanes; N: Crepidomanes subgenus Nesopteris; Va: genus Vandenboschia; HE: “(Hemi)-Epiphytic” clade (see text).
The inferred evolution of stele type is shown in Fig. 4A (left, with probabilities in the Appendix). A massive stele type appears to be the ancestral state in the trichomanoids. Massive stele types were also inferred for: a clade which we named HE, for (hemi)-epiphytic, because it mostly includes climbing hemi-epiphytes and epiphytes; for the clade grouping the Crepidomanes, Didymoglossum and Vandenboschia genera; and for the common ancestor of the clade grouping the Crepidomanes and Vandenboschia genera. Probabilities for these reconstructions were low (P < 0.62). The alternative scenario proposed a sub-collateral state for the same nodes, also with low probabilities (P < 0.38).
When treating both stem thickness and stele diameter as binary characters (according to the classes defined here-above), we found similar evolutionary scenarios for both characters, regardless of the model selected (MK1 or AsymmMK). Consequently, only the evolution of stem thickness (with MK1 model) is reported in Fig. 4A (right). For stele thickness, the ancestral state of the trichomanoids was inferred as large (larger than 750 μm) and the inferred derived small stem (smaller than 750 μm) would have appeared at least four times: in genus Polyphlebium, in genus Didymoglossum, in Crepidomanes subgenus Crepidomanes and in T. polypodioides. These inferences are supported by high probabilities (P ≥ 0.92, see Appendix). The stele diameter exhibited the same evolution with the exception of T. polypodioides, which retained a large stele (larger than 150 μm in diameter).
The inferred evolution of cortex is shown in Fig. 4B (left, with probabilities in the Appendix). A homogeneous cortex appears to be ancestral in the trichomanoids. If so, then the heterogeneous cortex would have evolved at least three times: in T. scandens, in T. polypodioides (or once in a clade grouping these species, if we propose this scenario to resolve the tritomy), and once in the common ancestor of the HE clade (with one reversal in Vandenboschia maxima). Again, these results are supported by high probabilities (P ≥ 0.93, see Appendix).
The inferred evolution of growth-form is shown on Fig. 4B (right). Since individual growth was inferred as ancestral in trichomanoids, coloniality (or colonial growth) would have appeared four times: in the HE clade (with one reversal in V. maxima and one reversal in Cr. intermedium-Cr. thysanostomum), in the Trichomanes subgenus Lacostea, in T. polypodioides, and in T. scandens. These results are supported by high probabilities (P ≥ 0.87).
Our comparative analyses did not reveal any significant phylogenetic correlations either between stele type and cortex type or between stele type and growth-forms, regardless of the coding of stele type (multistate or binary, not detailed here). By treating stele type as a binary character, significant correlations were revealed between sub-collateral-collateral type and small stem (as reported on Fig. 4A, p < 0.001) and between sub-collateral-collateral type and small stele (LR = 20.325, p < 0.001). Thus, the evolution of stem thickness strongly correlated phylogenetically with stele diameter. The heterogeneous cortex correlated phylogenetically with colonial growth (as reported on Fig. 4B, p < 0.001).
4 Discussion
4.1 Anatomical diversity in trichomanoids
Our observations reinforce our previous findings of relative anatomical homogeneity at the generic and sub-generic levels (see Table 2) as already proposed and extrapolated from incomplete data [2,6]. Nevertheless, they reveal some exceptions, especially concerning the cortex (e.g., V. maxima with a homogeneous cortex and T. scandens with a heterogeneous cortex). Our observations also allow us to correct the following misinterpretations. V. maxima was previously described to have a heterogeneous cortex [6] but our new investigation clearly shows that it is homogeneous (Fig. 2H), suggesting that an error was made in the previous study. In addition, the Crepidomanes subgenus Nesopteris was previously described to have a homogeneous cortex (except for Cr. aphlebioides) [2,6], whereas our new investigation clearly reveals a heterogeneous type for the entire clade. Previous observations were made on old, poorly preserved herbarium specimens displaying a thick, dense suberified stem that did not facilitate section and interpretation of structures and tissues. The use of more recently collected and better-preserved material permitted us to observe a un-suberified outer part in the cortex (or a developed hypodermis as we proposed here). We suspect that the outer part could have been eliminated in previous studies during the sectioning procedure or that the cortex (including hypodermis) could be fully suberified in old stems, which would make the inner and outer parts difficult to distinguish. Traditionally, terrestrial Nesopteris taxa were considered closely related to Abrodictyum because of their individual growth, short-creeping to erect stem and their clustered large erect fronds [3]. Although these characters appear plesiomorphic in trichomanoids and are therefore not valuable for phylogenetic clustering, no morphological characters have previously supported the alternative molecular-based inclusion [2] of Nesopteris into a clade mostly consisting of colonial epiphytic and climbing taxa with long-creeping stems and remote fronds (i.e., the HE clade). Our anatomical corrections therefore provide additional support for the inclusion of Nesopteris (especially the terrestrial species) in the HE clade and in the genus Crepidomanes.
4.2 Anatomical evolution in trichomanoids
A massive stele combined with a homogenous cortex was inferred to be ancestral or plesiomorphic in trichomanoids. This is in agreement with a previous study on anatomical evolution in the lineage performed on incomplete data and using a maximum parsimony (MP) procedure [6]. For stele type, our ML analysis proposed the same scenario inferred by the previous MP analysis selecting a DELTRAN optimization (which favours changes in terminal branches and thus parallelisms) [6]. In the MP analysis, by treating characters as ordered and assuming that a reduced stele was a priori intermediate between massive and sub-collateral/collateral types, a reduced stele was inferred for the common ancestor of the HE clade, from which would have independently evolved a sub-collateral type in genus Polyphlebium and in Crepidomanes subgenus Crepidomanes, and a collateral type in genus Didymoglossum, with reversal to massive from reduced type in V. maxima (with corrected data) [6]. This scenario is nevertheless not retrieved with our ML analysis, which does not provide the possibility to order or polarize the character states a priori. We could thus propose a massive ancestral state that would have independently evolved to reduced, sub-collateral and collateral stele types, in accordance with our ML scenario and the parsimony analysis using DELTRAN optimization. We nevertheless do not reject the alternative scenario suggesting that the reduced type is an intermediate between massive and sub-collateral/collateral stele. In favour of this last scenario, it is biologically realistic to propose that sub-collateral and collateral types, characterized by ventral protoxylem (as evidenced in the largest specimens when the protoxylem is clearly distinguishable), may come from the reduced type, characterized by central protoxylem, by reduction of the ventral part of the xylem. The fact that we did not infer this scenario with unordered parsimony and with ML could be due to bias in the phylogeny caused by the use of extant species only. It is thus possible that several lineages or taxa with reduced steles in the HE clade would have diversified before the appearance of the sub-collateral and the collateral clades. Most or some of these old lineages with reduced steles would be now extinct (and thus considered ‘ghost lineages’) and the reduced type would remain only in the extant genus Vandenboschia. The success of the sub-collateral and collateral clades, which currently appear more diversified than the reduced one, could explain why the ML analysis does not infer a reduced state as ancestral in HE. The less common state observed in a clade has indeed the lowest probability to be inferred on the basal branches. The alternative scenario proposing a reduced type as ancestral in HE clade should be tested by adding data, especially from fossils. However, the fossil record for filmies is scarce and poor [21,29], and is unfortunately useless for anatomical studies.
Whatever stele state is inferred for the common ancestor of the HE clade, our analyses suggest, in trichomanoids, at least three major diversifications related to anatomy. The appearance of sub-collateral and collateral steles, which correlated strongly with a decrease in stem thickness and stele diameter, appears also strongly related to the diversification of the Didymoglossum and Polyphlebium genera and of Crepidomanes subgenus Crepidomanes. These decreases in size and anatomy suggest a general regressive evolution of the anatomy in these three clades, corroborating recent hypotheses already proposed on morphology. This is also in disagreement with the first proposition on the subject published by Prantl who proposed that the ancestral stele in the Hymenophyllaceae was of the collateral type [30]. Boodle, 25 years later, suggested an alternative scenario, considering that both (sub)-collateral and massive types would have independently derived from the reduced one [11]. These older hypotheses were likely influenced by the fact that the species these authors studied were most frequently epiphytic and exhibited particular anatomies rather than the standard (or ancestral) one most commonly displayed by terrestrial species (see Table 2).
Moreover, T. polypodioides and all species belonging to genus Polyphlebium show a regressed root system (i.e., the stems bear few and filiform roots) and all species belonging to genus Didymoglossum and Crepidomanes subgenus Crepidomanes are rootless and develop root-like shoots [5]. In contrast, all other trichomanoids bear a developed root system with numerous and robust, large, and thick roots [2,5,6]. These observations reveal that the anatomical regression of the stem was accompanied by a regression in the adventious root system in at least four groups. Consequently, we could hypothesize that regressive evolution of the root system and anatomy could be applied, in the same groups, to other morphological characters (e.g., the global size of the sporophyte, which is mainly defined by the frond length). This would be consistent with dwarfism tendencies, as already proposed [4–7]. In other words, regression in stem anatomy could be accompanied by a reduction in blade size and species with the thinnest stems and the most regressed anatomies would also be the smallest ones. The present data did not reveal any significant graphical correlation between blade lengths and stem thickness for all the taxa showing anatomical regression (see Fig. 3A, left cluster). A correlation between stem thickness and blade length was only evidenced in species with an ancestral massive stele, which thus exhibited no anatomical regression. The absence of a correlation in species displaying anatomical regression could be due to the fact that the stems were reduced to their lower limits of thickness. In addition, we neither inferred the evolution of blade length nor tested the phylogenetic correlation with stem thickness or stele diameter because no blade size categorization was possible, at least with the present sampling and data. A previous study on an expanded taxonomic sampling nevertheless suggests three size classes for the trichomanoids [4]: large plants (with fronds longer than 12 cm), small to dwarf plants (with fronds shorter than 6 cm) and medium-size plants (with fronds of intermediate length). The genus Didymoglossum and Crepidomanes subgenus Crepidomanes indeed respectively comprise more than 88% and more than 84% of small or dwarf species (with the remaining ones being medium sized), while the genus Polyphlebium includes less than 46% of small or dwarf species. If dwarfism defined here by frond size is likely to be preponderant in clades exhibiting anatomical regression, then a strict correlation between dwarfism and anatomy regression either cannot be proposed or it must be restricted to the genus Didymoglossum and Crepidomanes subgenus Crepidomanes. Dwarfism is observed in other fern families, and especially in traditional Grammitidaceae and the genus Microgramma and relatives (all belonging to the Polypodiacaeae), which include many of the smallest living ferns. However, the smallest Polypodiaceae species display a typical dictyostele that differs only from that of their largest relatives by the size and the number of meristeles [16]. Dwarf species are also observed in the Vittariaceae, which exhibit protosteles, ectophloic solenosteles and dictyosteles [16], but no study has yet assessed the evolution of anatomy in this group nor tested whether these three types can be included in an evolutionary series. Our study is therefore the first to evidence vascular plant anatomical regression combined with significant changes within a single type (i.e., the protostele).
Regarding cortex evolution, the ML analysis allowed us to propose a scenario similar to that of the previous MP analysis, with a major difference concerning the reversal from heterogeneous to homogeneous cortex, which was suggested by MP in the clade (Cr. intermedium-Cr. thysanostomum) [6]. By correcting the cortex type in the subgenus Nesopteris and in V. maxima, we now infer only one reversal in the HE clade, in V. maxima. We previously assumed that a petiole-like cortex was a variant of the heterogeneous cortex, hence the binary coding for the cortex character. However, based on the distribution of petiole-like cortex in the genera Didymoglossum, Polyphlebium, and Crepidomanes subgenus Crepidomanes, we now propose that a petiole-like cortex would have derived directly from the heterogeneous one by secondary reduction of the hypodermis in these three clades. However, a petiole-like cortex is also displayed by T. polypodioides, which is included in a broad clade composed of taxa mostly exhibiting homogeneous cortex (genus Trichomanes). The supposed petiole-like cortex observed in this species would, in that single case, be derived from homogeneous type. Cortex was neglected in previous studies (except in that of Le Thomas [13]) because most anatomical investigations focused on stele features. In his review on the subject, Ogura described cortex as heterogeneous for the family, giving the impression that the heterogeneous type is the only type observable [16]. We demonstrate and confirm here that the cortex is highly variable, as already evidenced [6,13], and that the homogeneous type represents the plesiomorphic state within trichomanoids.
4.3 Growth-forms and anatomy in trichomanoids
Individual growth is clearly inferred as ancestral in trichomanoids. Colonial growth would have appeared in the HE clade and, outside HE clade, in lianas (Trichomanes subgenus Lacostea) and in some climbing hemi-epiphytes (T. scandens). The coloniality is expected in climbers, which have the ability to provide more than one shoot climbing on the substrates, but not obligatory because the climbing epiphytic species Abrodictyum caudatum and A. flavofuscum are not colonial. Molecular dating estimated the HE clade to have originated between the upper Jurassic and the lower Cretaceous [21]. Our analysis thus suggests that the first colonial species (the ancestor of the HE clade) would have diversified in the understory of the first angiosperm-dominated rainforests in the Cretaceous, as for most modern fern lineages [31]. It is possible to observe in extant V. gigantea how this secondary climbing hemi-epiphyte first colonizes a wide surface of the ground of the understory, then climbs on trunks where it produces fertile fronds [4]. The elevation of these fronds a few meters above the ground is expected to maximize spore dispersal. In forest understory, the coloniality is therefore advantageous because it increases the probability, for individuals, to find a vertical support. Although habitat evolution has not been precisely studied here, we could suggest that climbing hemi-epiphytic trichomanoids would also have diversified in the first angiosperm-dominated forests and that this habitat would be related to coloniality acquisition in the group. Future studies should aim to test this hypothesis by exploring and testing correlations with habitats and precise ecological preferences.
Our analysis reveals a strong statistical relationship between colonial growth and heterogeneous cortex (see Fig. 4), suggesting that cortical heterogeneity could provide some advantage to coloniality or that cortical heterogeneity would have been selected in a colonial context. However, such an advantage could also depend on the habitat. Heterogeneous cortex is only observed, in the extant Hymenophyllaceae, in colonial climbing and epiphytic species. Conversely, terrestrial trichomanoids, including those with creeping rhizomes but not colonial ones (e.g., T. robustum and A. pluma), always display a homogeneous cortex. In other families with terrestrial species exhibiting long-creeping, branched rhizomes (such as in the bracken fern Pteridium aquilinum, the Dennstaedtiaceae, or the Gleicheniaceae), the cortex is also homogeneous. Climbing species are characterized by long, thick, and robust stems that can climb several meters vertically on a support. This habitat requires both stem biomechanical rigidity for climbing ability and flexibility for resistance against twisting [32]. The peripheral suberification of the inner part of a heterogeneous cortex would provide rigidity, to add to that provided by the thick protostele displayed by climbing trichomanoids. The outer part (supposed a hypertrophied hypodermis), which is directly in contact with the substrate, would provide the required flexibility. The biomechanical properties of such anatomy remain to be assessed using accurate comparative biomechanical studies. If we assume, however, that cortex heterogeneity would have been biomechanically selected in a climbing context, instead of a colonial context, its appearance in the common ancestor of the HE clade would reinforce our current hypothesis that climbing hemi-epiphytism was acquired in the same node. Finally, the majority of colonial and non-climbing epiphytes of the HE clade, belonging to the Didymoglossum and Polyphlebium genera and Crepidomanes subgenus Crepidomanes, exhibit thin lax rhizomes with petiole-like cortex, which we suppose derived from the heterogeneous type by reduction of the outer part or the hypodermis. We could suggest for these epiphytes that the rigidity and flexibility properties may be less required to colonize the substrate. The peripheral but not dense suberification of the cortex would provide the minimal required rigidity for stem cohesion and colonial growth as well as act as a barrier to limit water loss from the stems in a strongly desiccating epiphytic context. The reduced stele anatomy would enable sufficient stem flexibility, so that the latter part of the hypodermis would have been counter-selected. Here again, the biomechanical properties of such anatomy remain to be assessed.
4.4 Hypotheses for further works
The earliest trichomanoids are here inferred as robust plants displaying individual growth, a standard developed protostele and a homogeneous cortex, which are related to a thick stem with a developed root system (as also observed for all extant terrestrial taxa of the group). Colonial growth would have been selected in a clade dating from at least the lower Cretaceous, likely in the understory of the first angiosperm-dominated forests. Although our present analyses do not yet statistically propose an ancestral habitat for the first colonial filmies, the hypothesis that their climbing hemi-epiphytism appeared in relation to coloniality in the HE clade warrants further study, as does the heterogeneous cortex which may have been selected in relation to a climbing habit. Furthermore, anatomical regression, illustrated by sub-collateral and collateral type acquisition and related to reductions in stem and stele size, was observed in three groups embedded in the HE clade and involves only colonial epiphytes. This is in accordance with previously published assumptions on regressive morphological evolution in the epiphytic Hymenophyllaceae, which also suggest a “bryophyte-like” strategy [7]. This strategy does not seem to be unique, however, because epiphytism outside the HE clade also concerns species exhibiting a massive stele, a thick stem and robust roots. This is the case, in the sampling used here, for A. tamarisciforme, A. flavofuscum, A. caudatum, T. crispum, T. alatum, and T. polypodioides (apart from the stem thickness and roots for this last species). Further comparative and phylogenetic analyses integrating accurate ecological preferences are expected and should therefore test hypotheses of evolutionary relationships among: a) coloniality and heterogeneous cortex to climbing habit; and b) morphological and anatomical regression to colonial epiphytism, at least in the HE clade.
Disclosure of interest
The authors declare that they have no conflicts of interest concerning this article.
Acknowledgments
This work, and especially the field trips for specimen acquisition, was supported by UMR 7207 “Centre de recherche sur la paléobiodiversité et les paléoenvironnements”, IFR 101 “Institut d’écologie, biodiversité, évolution, environnement” and the PPF MNHN “État et structure phylogénétique de la biodiversité actuelle et fossile”. The molecular work was partly supported by JSPS fellow for A. Ebihara. We thank G. Rouhan, F. Rakotondrainibe, J.-N. Labat for the use of P herbarium specimens, C. Chaussidon, G. Rouhan, F. Rakotondrainibe, C. Reeb, A. Le Thomas, and H. Schneider for discussions on filmy fern anatomy, biology and ecology, T. Dufour, N. Salel, E. Watroba for precious help for anatomy investigations, and Kurt Liittschwager for his helpful advice and corrections of the English.
Appendix A
Probabilities of inferred ancestral states for anatomy and growth forms for representative trichomanoid clades. The values correspond to the probability of each state on selected clades according to a MK1 model (single value) or respectively to a MK1 model and an AsymmMK model (two values; for cortex, coloniality, stem thickness and stele diameter).
Stele Type (Fig. 3A) | Cortex (Fig. 3B) | Coloniality (Fig. 3B) | ||||||
Clade | Massive | Reduced | Sub-collateral | Collateral | Homogeneous | Heterogeneous | Non colonial | Colonial |
(Ca-Ce) | 1 | 0 | 0 | 0 | 1-1 | 0 | 0.99-0.99 | 0.01-0.01 |
Ab | 1 | 0 | 0 | 0 | 1-1 | 0 | 1-1 | 0 |
(A. caudatum-A. flavofuscum) | 1 | 0 | 0 | 0 | 1-1 | 0 | 1-1 | 0 |
(Tr-HE) | 1 | 0 | 0 | 0 | 0.98-0.99 | 0.02-0.01 | 0.96-0.97 | 0.04-0.03 |
Tr | 1 | 0 | 0 | 0 | 0.99-1 | 0.01-0 | 0.99-0.99 | 0.01-0.01 |
(T. scandens-T. polypodioides-T. alatum) | 1 | 0 | 0 | 0 | 0.62-0.80 | 0.38-0.20 | 0.61-0.71 | 0.39-0.29 |
D-L | 1 | 0 | 0 | 0 | 0.96-0.97 | 0.04-0.03 | 0.92-0.93 | 0.08-0.07 |
L | 1 | 0 | 0 | 0 | 0.01-0.02 | 0.99-0.98 | 0.02-0.02 | 0.98-0.98 |
HE | 0.61 | 0.03 | 0.34 | 0.02 | 0.04-0.07 | 0.96-0.93 | 0-0.13 | 1-0.87 |
Po | 0 | 1 | 0 | 0 | 0 | 1-1 | 0 | 1-1 |
(Cr-Va-Di) | 0.59 | 0.04 | 0.33 | 0.04 | 0.01-0.02 | 0.99-0.98 | 0.04-0.07 | 0.96-0.93 |
Di | 0.02 | 0 | 0.02 | 0.96 | 0 | 1-1 | 0.02-0.02 | 0.98-0.98 |
(Cr-Va) | 0.57 | 0.08 | 0.33 | 0.02 | 0 | 1-1 | 0.02-0.04 | 0.98-0.96 |
Cr | 0.58 | 0.04 | 0.37 | 0.01 | 0 | 1-1 | 0.03-0.05 | 0.97-0.95 |
N | 0.99 | 0 | 0.01 | 0 | 0 | 1-1 | 0.24-0.28 | 0.76-0.72 |
(Cr. Thysanostomum-Cr. intermedium) | 1 | 0 | 0 | 0 | 0 | 1-1 | 1-0.97 | 0-0.03 |
C | 0.02 | 0 | 0.98 | 0 | 0 | 1-1 | 0.01-0.01 | 0.99-0.99 |
Va | 0.01 | 0.98 | 0.01 | 0 | 0 | 1-1 | 0-0.01 | 1-0.99 |
Stem thickness (Fig. 3A) | Stele diameter | |||||||
Clade | Thick | Thin | Large | Small | ||||
(Ca-Ce) | 1-1 | 0-0 | 1-1 | 0-0 | ||||
Ab | 1-1 | 0-0 | 1-1 | 0-0 | ||||
(A. caudatum-A. flavofuscum) | 1-1 | 0-0 | 1-1 | 0-0 | ||||
(Tr-HE) | 1-1 | 0-0 | 1-1 | 0-0 | ||||
Tr | 1-1 | 0-0 | 1-1 | 0-0 | ||||
(T. scandens-T. polypodioides-T. alatum) | 1-1 | 0-0 | 1-1 | 0-0 | ||||
D-L | 1-1 | 0-0 | 1-1 | 0-0 | ||||
L | 1-1 | 0-0 | 1-1 | 0-0 | ||||
HE | 0.73-0.88 | 0.27-0.12 | 0.73-0.81 | 0.27-0.19 | ||||
Po | 0.01-0.01 | 0.99-0.99 | 0.01-0.01 | 0.99-0.99 | ||||
(Cr-Va-Di) | 0.73-0.88 | 0.27-0.12 | 0.73-0.81 | 0.27-0.19 | ||||
Di | 0.08-0.11 | 0.92-0.89 | 0.06-0.07 | 0.94-0.93 | ||||
(Cr-Va) | 0.79-0.92 | 0.21-0.08 | 0.79-0.86 | 0.21-0.14 | ||||
Cr | 0.76-0.89 | 0.24-0.11 | 0.77-0.84 | 0.23-0.16 | ||||
N | 0.98-0.99 | 0.02-0.01 | 0.99-0.99 | 0.01-0.01 | ||||
(Cr. Thysanostomum-Cr. intermedium) | 1-1 | 0-0 | 1-1 | 0-0 | ||||
C | 0.06-0.08 | 0.94-0.92 | 0.06-0.05 | 0.94-0.95 | ||||
Va | 0.99-1 | 0.01-0 | 0.99-0.99 | 0.01-0.01 |