1 Introduction
Type 2 Diabetes is a multifactorial disease that results from the interaction of environmental factors and genetic predisposition leading to two major abnormalities: insulin resistance and defective pancreatic β-cell function. During the long lasting silent phase that precedes the onset of type-2 diabetes, known as prediabetes, hyperinsulinemia compensates for insulin resistance. Hyperglycemia then develops with a progressive β-cell failure but the mechanisms involved remain to be determined. From epidemiological studies, it appears that lifestyle related overweight and a familial history are major risk factors. Persistent imbalance between energy intake and expenditure, with the likely consequence of obesity, can lead to overt diabetes when associated to genetic predisposition.
Even if there is no animal model that reproduces the natural history of human type 2 diabetes, the gerbil Psammomys obesus, also known as the sand rat deserves special attention. This small rodent eats essentially low caloric succulent halophilic plants (Chenopodiaceae family) and remains normoglycemic in its biotope. However, when the low energy diet is switched to regular rodent laboratory chow, they rapidly gain weight, become obese and develop progressively the heterogeneous pattern of metabolic defects that occur in human metabolic syndrome and type-2 diabetes [1,2]. In addition, it must be emphasized that, after 6 months, about 25% of them display type 1 insulin-dependent diabetes with severe hyperglycemia and a drastic drop in plasma insulin levels as a result of inappropriate insulin production and depleted insulin stores [3–5], later shown to be associated with insulitis [6]. In this respect, IL-1β is a pro-inflammatory cytokine acting during the autoimmune process [7] of type 1 diabetes. Furthermore, upon prolonged exposure of purified human or rodent β cells to a combination of IL-1β and IFN-γ, about 50% of these cells undergo apoptosis after 6-9 days [8]. If type-1 diabetes is typically caused by an autoimmune assault inducing progressive β cell death, the importance of β-cell mass reduction in insulin secretion defect in type 2 diabetes is not established [9]. In contrast, there is now growing evidence that obesity is characterized by a chronic systemic low grade of inflammation and that biomarkers of inflammation predict the development of type 2 diabetes [10]. Therefore, we were interested to study the in vitro effects of 2 cytokines, IL-1β and IFN-γ in Psammomys obesus fed a high energy laboratory chow during 9 months. In our experimental conditions we did not observe the occurrence of insulin-dependent diabetes but in accordance with observations in humans consuming hypercaloric diets and probably due to the heterogeneous genetic background of our animals, we obtained 2 clearly distinct groups, obese and non-insulino dependent type-2 diabetic animals in which we tested and compared the effects of increasing concentrations of the 2 cytokines. We also compared the sensitivity to IL-1β and IFN-γ in Psammomys obesus and Wistar rats.
2 Materials and methods
2.1 Animals
In our study, we used wild sand rats trapped in collaboration with the Algiers Beni-Abbes research station in the South of Algeria and transferred to our laboratory's breeding farm in Algiers. A 15-day adaptation period was allowed and animals had free access to their natural food and water. Thereafter, two experimental groups were isolated. Control animals were maintained on their natural food, i.e. halophilic plants (Chenopodiaceae family) poorly caloric (0.4 kcal/g fresh weight), rich in water and salts; each of them ate about 50 g/day. A second group received 10 g/day of normal (3.25 kcal/g) laboratory chow for rodents for 9 months. Wistar rats (Rattus Norvegicus) were obtained from the Pasteur Institute in Algiers and fed the regular laboratory chow (Table 1).
Diet compositions.
(%) | Natural halophilic plants | Standard rodent chow |
Water | 80.8 | 9 |
Minerals | 6.9 | 6 |
Lipids | 0.4 | 13 |
Proteins | 3.53 | 22 |
Carbohydrates | 8.42 | 44 |
Other non specified materials |
— | 3 |
2.2 Biochemicals
Collagenase (Cl. histolyticum, 0.1 pz U/mg), Bovine Serum Albumin (fraction V), and D-glucose were purchased from respectively Serva, Sigma and Pacreas. Human Synthetic Interleukin-1β and Interferon-γ were obtained from Boehringer Mannheim.
2.3 Plasma assays
Blood was sampled from the retro-orbital sinus. Plasma aliquots obtained after centrifugation (10 min at 3000 rpm) were frozen (–25 °C) until plasma determinations. Glucose was measured by a colorimetric enzymatic method using the Boehringer kit. Plasma triglycerides and total cholesterol were determined by enzymatic colorimetric methods using respectively Ames and Boehringer kits. Insulin was measured in plasma and incubation media using the radio-immunological assay kit developed by CIS (ORIS INDUS).
2.4 Pancreatic islets experiments
Each animal was killed by decapitation, its pancreas was dissected out and islets isolated using the collagenase method previously described by Lacy et al. [11]. They were then hand-picked under a stereomicroscope and batches of 5 islets incubated in 2 mL Krebs-Ringer bicarbonate buffer supplemented with Bovine Serum Albumin (2 g/L) and glucose and interleukins at the appropriate concentrations.
2.5 Statistics
In text and figures data are expressed as means ± SD. The statistical difference between means was determined using Student's t test for paired values.
3 Results
3.1 Effects of the 9-month hypercaloric diet on plasma glucose and insulin levels in Psammomys obesus
On day 0, just before the onset of the hypercaloric diet, plasma insulin and glucose levels were similar in the three experimental groups (data not shown). A control group was maintained on the normal herbal diet. In the hypercaloric diet group, at the end of the 9-month diet, all animals were clearly overweighed; they were divided into 2 groups according to their fasting plasma glucose levels. Normoglycaemic sand rats were considered as obeses and clearly hyperglycaemic ones as diabetics. From Fig. 1 it appears that at the end of the hypercaloric diet in the obese group, plasma glucose was slightly but not significantly increased, reaching 55.2 ± 2.0 versus 48.4 ± 2.2 mg/100 mL at 0 time. This slight increase was paralleled by a drastic rise in plasma insulin from 27.5 ± 1.6 μUI/mL at the beginning of the diet to 113.4 ± 5.7 and 269.0 ± 18.6 μUI/mL after 3 and 9 months (Fig. 2). In contrast in diabetic animals, plasma glucose was already significantly increased by about 62% at time 3 months (p < 0.05), reaching 260.9 ± 35.7 mg/100 mL after 9 months (p < 0.001 versus 0 time value). Simultaneously, plasma insulin increased by respectively 590, 1035 and 840% after 3, 6 and 9 months.
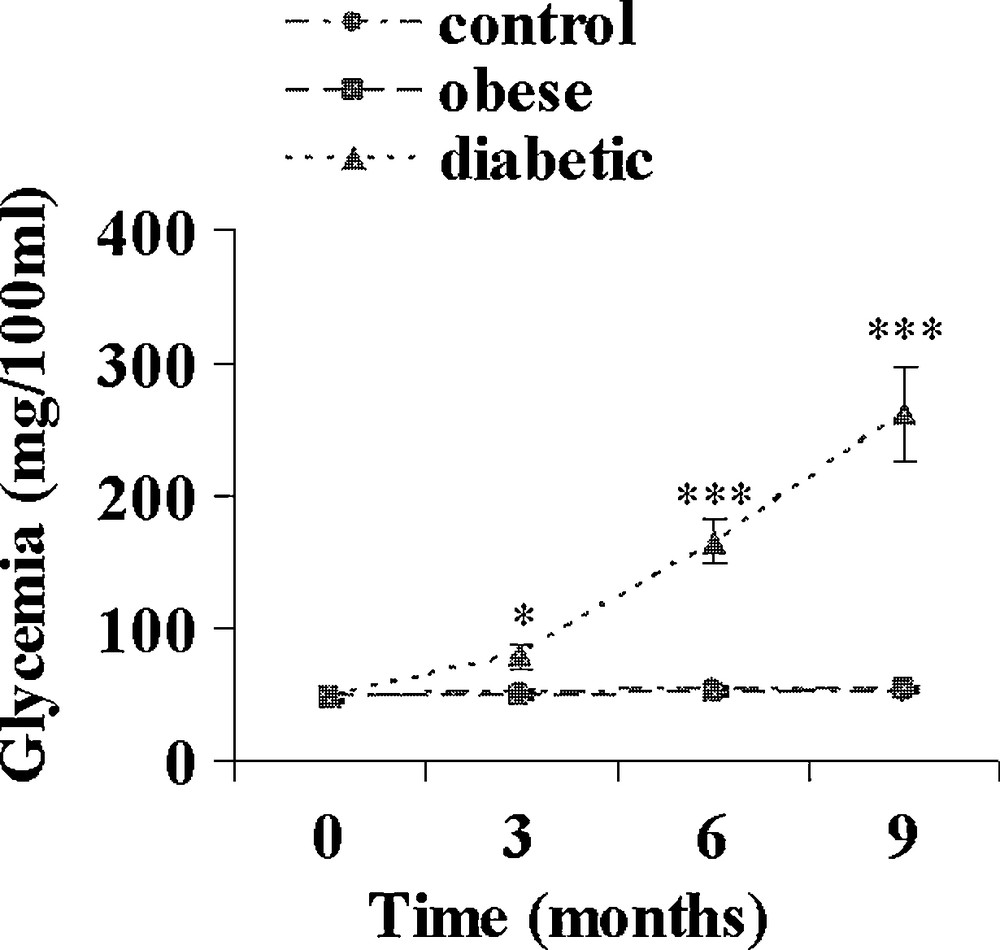
Evolution of plasma glucose during a 9-month high caloric diet with regular laboratory rodent chow in obese normoglycemic (n = 15) and obese diabetic (n = 12) Psammomys obesus. Lean controls (n = 11) received low caloric halophilic plants. *: p < 0.05 and *** p < 0.001 versus controls.
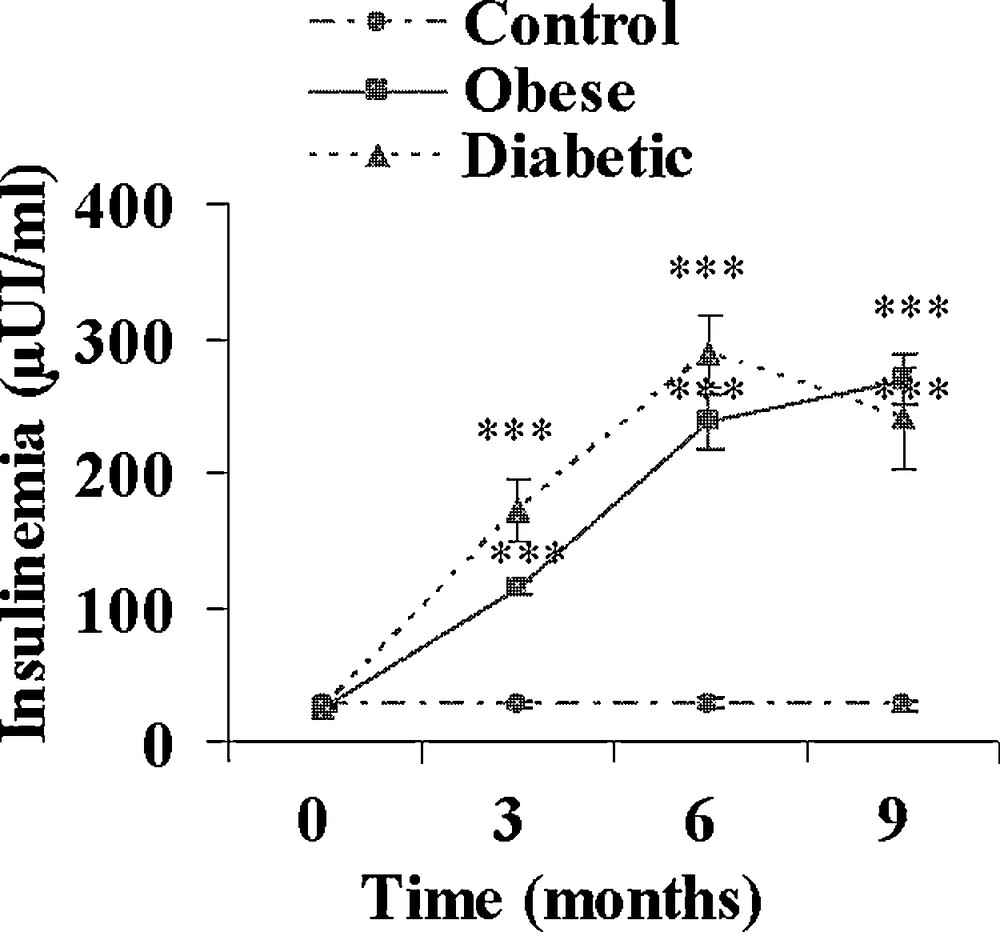
Evolution of plasma insulin during a 9-month high caloric diet with regular laboratory rodent chow in obese normoglycemic (n = 15) and obese diabetic (n = 12) Psammomys obesus. Lean controls (n = 11) received low caloric halophilic plants. ***: p < 0.001 versus controls.
3.2 Effects of the 9-month hypercaloric diet on body weight plasma triglycerides and cholesterol in Psammomys obesus
Both obese and diabetic sand rats developed a progressive but clear overweight reaching respectively + 60 and + 52% (p < 0.001) after 9 months of the high caloric diet (Table 2). Hyperlipidemia developed in both groups. The increase in plasma cholesterol was moderate in obese and diabetic animals (+ 30 and + 81%; Fig. 3), but the increase in plasma triglycerides was clearly more rapid and more marked in diabetic Psammomys obesus, reaching after 9 months + 64 and + 180% in respectively obese and diabetic animals (Fig. 4).
Changes in body weight during a 9-month high caloric diet with regular laboratory rodent chow in Psammomys obesus. Control animals received low caloric halophilic plants.
Body weight (g) | ||||
Time (months) | 0 | 3 | 6 | 9 |
Control [6] | 94.5 ± 7.4 | 98.3 ± 6.1 | 102.2 ± 5.1 | 101.2 ± 3.5 |
Obese [15] | 82.3 ± 3.8 | 102.4 ± 3.6 *** | 117.7 ± 3.2 *** | 131.0 ± 1.9 *** |
Diabetic [12] | 82.3 ± 3.8 | 114.6 ± 4.8 * | 126.6 ± 2.6 *** | 124.6 ± 4.2 *** |
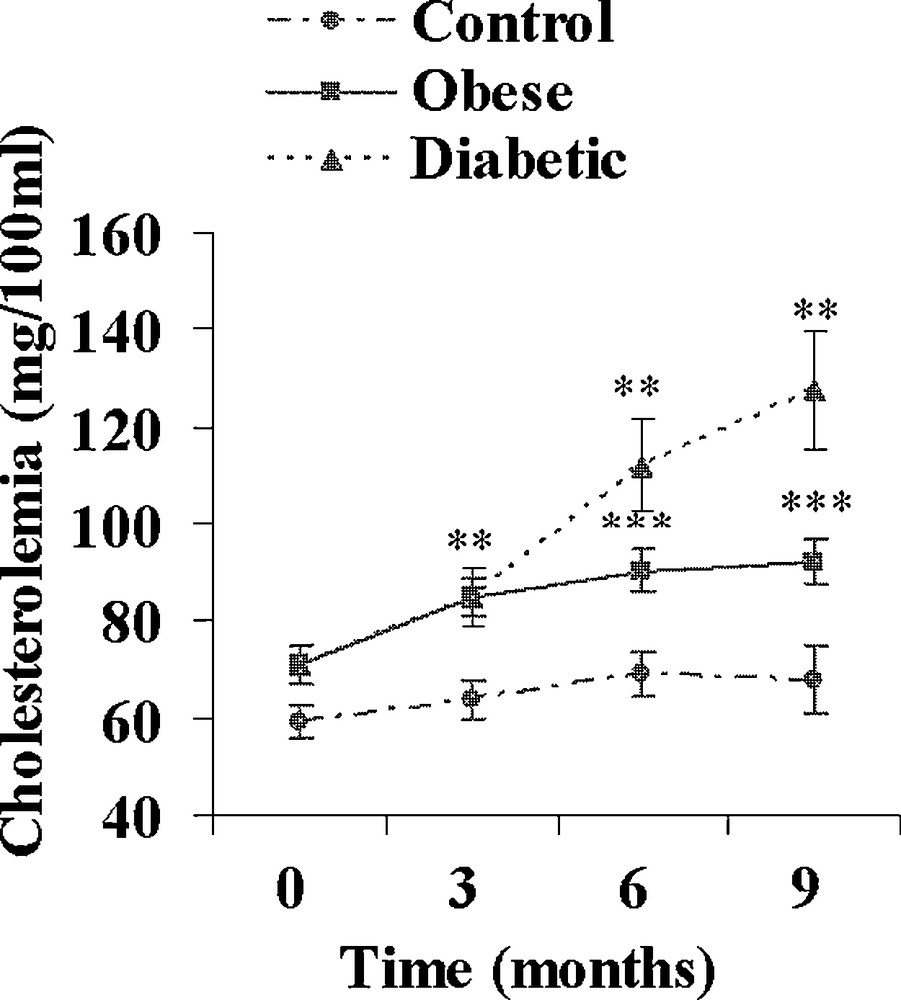
Evolution of plasma cholesterol during a 9-month high caloric diet with regular laboratory rodent chow in obese normoglycemic (n = 15) and obese diabetic (n = 12) Psammomys obesus. Lean controls (n = 11) received low caloric halophilic plants. **: p < 0.01 and ***: p < 0.001 versus controls.
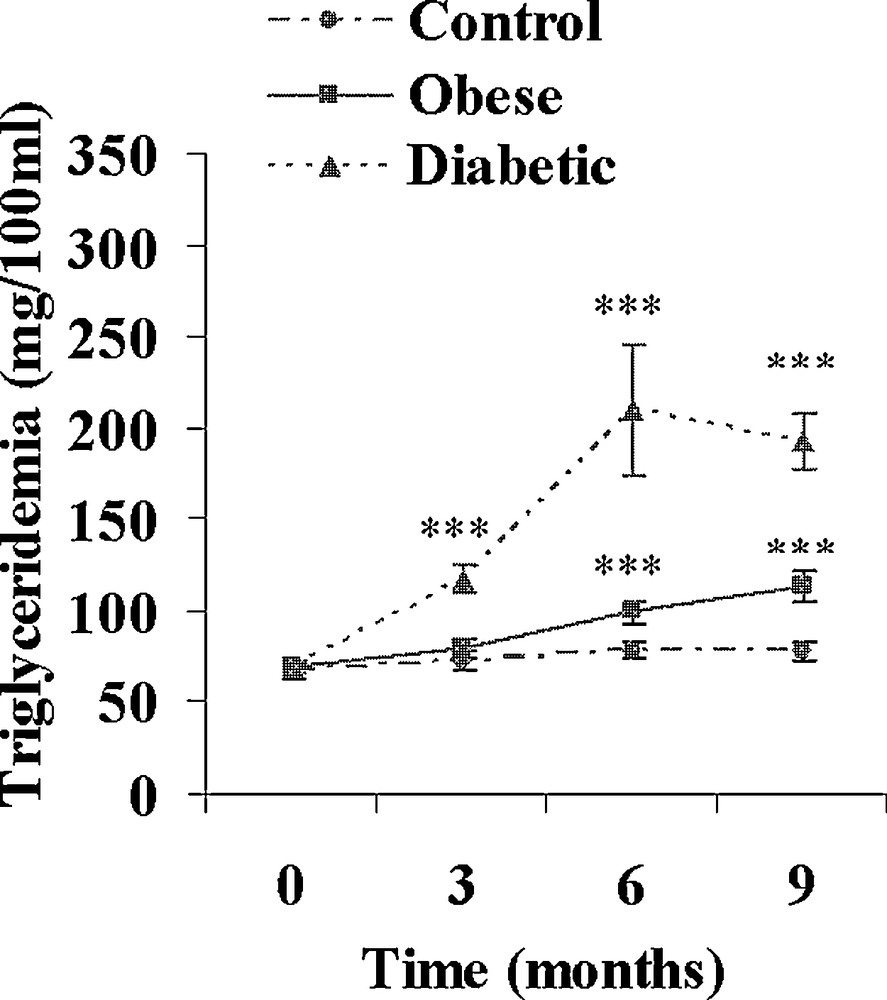
Evolution of plasma triglycerides during a 9-month high caloric diet with regular laboratory rodent chow in obese normoglycemic (n = 15) and obese diabetic (n = 12) Psammomys obesus. Lean controls (n = 11) received low caloric halophilic plants. ***: p < 0.001 versus controls.
3.3 Effect of IL-1β and IFN-γ on insulin release by isolated islets from lean control, obese and diabetic Psammomys obesus
IL-1β was tested at increasing concentrations: 1, 5, 10 and 20 UI/mL in the presence of 5 mM glucose (Fig. 5). In control sand rats, a significant 32% increase in insulin release was observed at the low 1 UI/mL concentration. At 5 UI/mL, insulin secretion further increased to 75.4 ± 4.5 μUI insulin/islet/90 min (+ 107% versus basal value; p < 0.001) a maximal value that remained stable for 10 and 20 UI/mL IL-1β. In obese sand rats, a maximal insulin release was already achieved at 1 UI/mL and a plateau occurred with values ranging between 112.1 ± 6.3 and 96.1 ± 6.4 μUI insulin/islet/90 min for respectively 5 and 20 UI/mL IL-1β. In insulinopenic diabetic Psammomys obesus, the cytokine was no longer able to affect the low basal insulin release at any of the concentrations tested. Concerning IFN-γ, no major effect could be observed in the three experimental groups at either 50, 100, 200 or 500 UI/mL (Fig. 6). It should however be noticed that a trend to a decrease occurred for the highest 400 UI/mL concentration in obese animals.
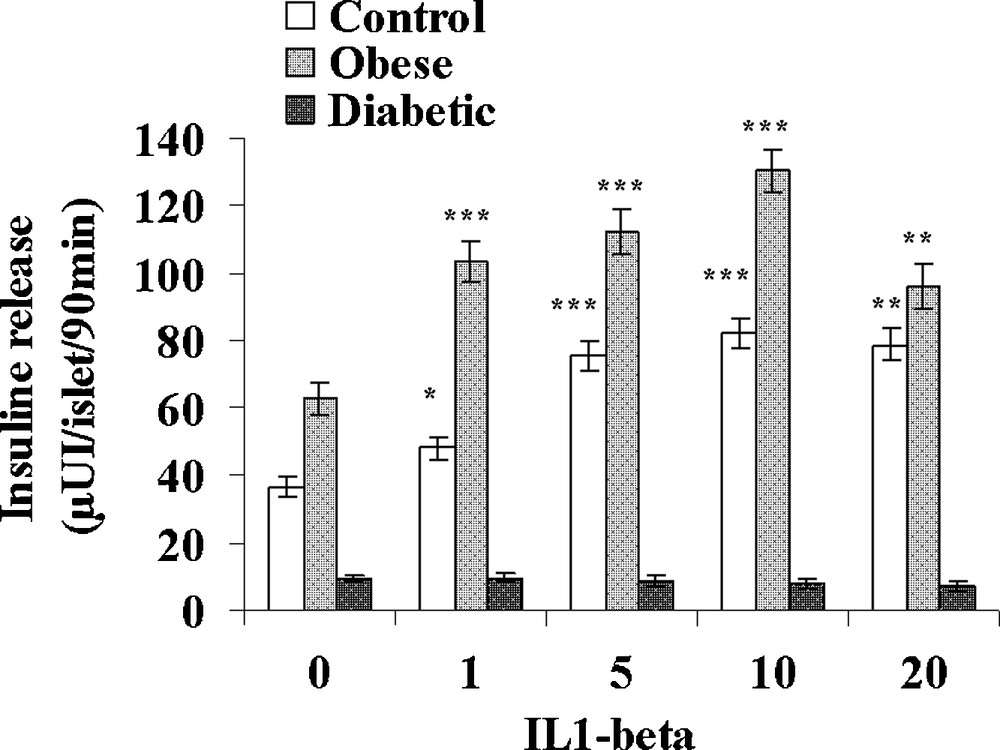
Effect of increasing concentrations of IL-1β on insulin secretion in pancreatic islets isolated from obese normoglycemic, obese type 2 diabetic and control lean Psammomys obesus after a 9-month high caloric diet with regular laboratory rodent chow. Lean control animals received low caloric halophilic plants. Each value is the mean of (n = 11 to 15) experiments; for each experiment 2 batches of 5 islets were performed for each experimental condition. *: p < 0.05, **: p < 0.01 and ***: p < 0.001 versus values obtained in the absence of IL-1β.
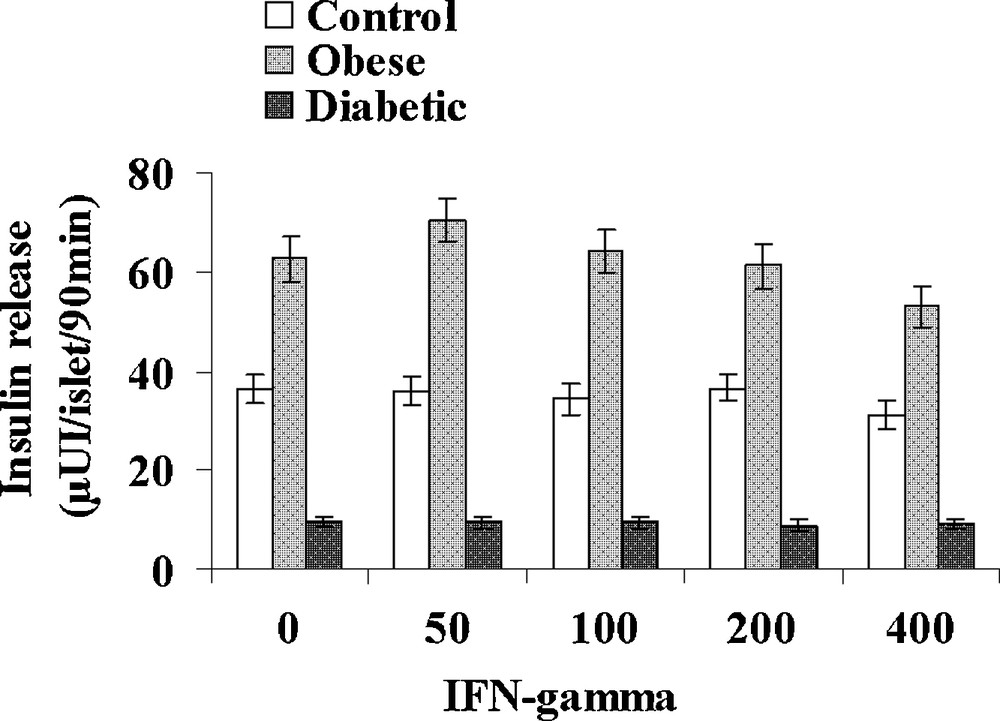
Effect of increasing concentrations of IFN-γ on insulin secretion in pancreatic islets isolated from obese normoglycemic, obese type 2 diabetic and control lean Psammomys obesus after a 9-month high caloric diet with regular laboratory rodent chow. Lean control animals received low caloric halophilic Plants. Each value is the mean of (n = 11 to 15) experiments; for each experiment, 2 batches of 5 islets were performed for each experimental condition.
3.4 Comparison of the effects of IL-1β and IFN-γ on insulin release by isolated islets from control Psammomys obesus and normal Wistar rats
Table 3 presents various parameters recorded in control lean Psammomys obesus and normal Wistar rats. Interestingly, the small rodent living in the desert displays a 50% lower glycaemia than Rattus norvegicus, together with a 41% lower plasma insulin level. In contrast, triglycerides and cholesterol levels were found slightly higher, respectively + 11 and + 29% in the sand rat. Pancreatic islets isolated from control lean Psammomys obesus and Wistar rats were incubated with either 1, 5, 10 and 20 UI/mL IL1β (Fig. 7) or 50, 100, 200 and 400 UI/mL IFN-γ (Fig. 8). In the Wistar rat, IL-1β was ineffective at 1UI/mL. At 5 UI/mL, a significant 62% increase could be observed. At higher concentrations, a very slight further increase occurred with a plateau reaching 51.6 ± 2.8 and 52.0 ± 2.9 μUI insulin/islet/90 min (p < 0.001), versus 29.2 ± 2.4 in the absence of the cytokine. As concerns Psammomys obesus, IL-1β stimulating effect occurs earlier at 1 UI/mL and at the plateau level it reaches values + 125% higher than basal ones (36.5 ± 2.9 μUI insulin/islet/90 min in the absence of cytokine). Whatever the concentration tested, IFN-γ did not affect insulin release in the two experimental groups.
Plasma insulin, glucose, triglycerides, cholesterol and body weight recorded in control Wistar and lean sand rats.
Body weight (g) | Plasma insulin μUI/ml |
Plasma glucose (mg/100 ml) |
Plasma triglycerides (mg/100 ml) |
Plasma cholesterol (mg/100 ml) |
|
Wistar rat [6] | 239.50 ± 4.40 | 50.40 ± 5.89 | 105.00 ± 5.47 | 68.63 ± 3.15 | 47.00 ± 2.42 |
Sand rat [6] | 94.50 ± 7.40*** | 27.00 ± 2.40* | 47.83 ± 4.56 *** | 67.00 ± 0.04 | 59.00 ± 0.03** |
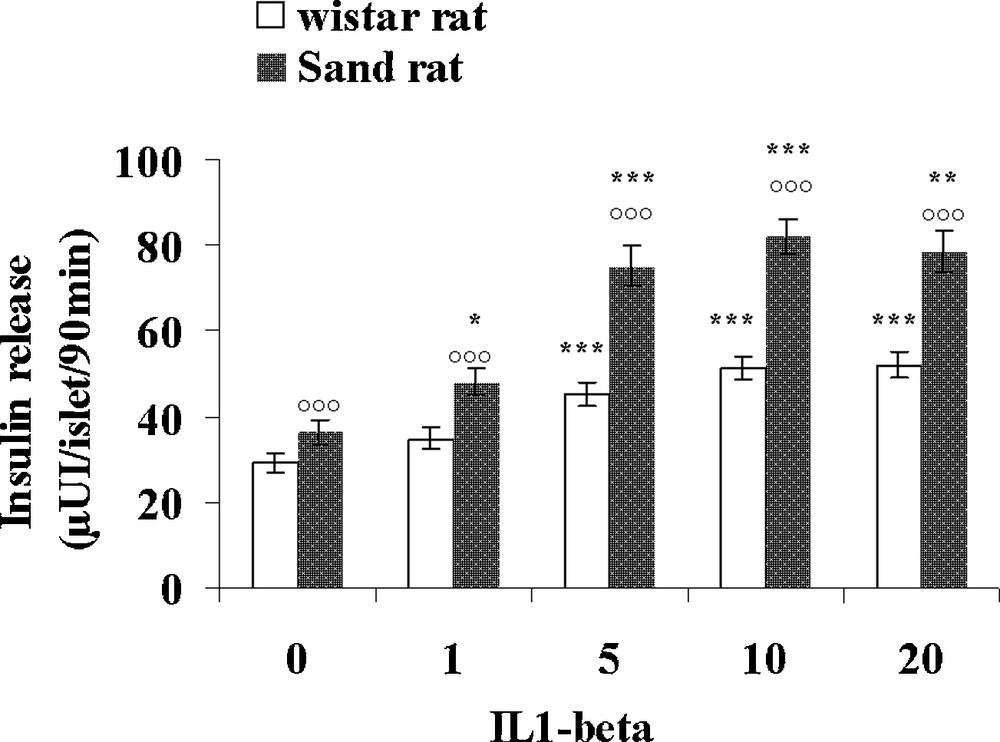
Comparison of the effects of increasing concentrations of IL-1β on insulin secretion in pancreatic islets isolated from lean control Psammomys obesus and Wistar rats. Each value is the mean of n = 12 experiments; for each experiment 2 batches of 5 islets were performed for each experimental condition. *: p < 0.05, **: p < 0.01 and ***: p < 0.001 versus values obtained in the absence of IL-1β: p < 0.001 versus Wistar rats values.
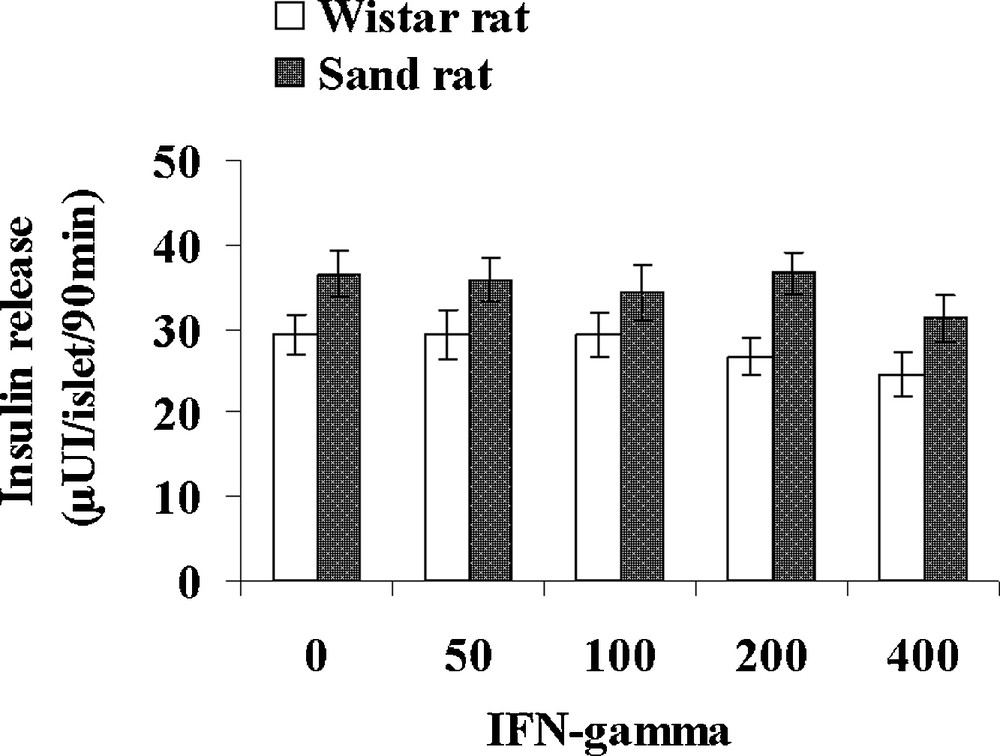
Comparison of the effects of increasing concentrations of IFN-gamma on insulin secretion in pancreatic islets isolated from lean control Psammomys obesus and Wistar rats. Each value is the mean of n = 12 experiments; for each experiment 2 batches of 5 islets were performed for each experimental condition.
4 Discussion
In our study, switching the natural low caloric diet of Psammomys obesus to normal rodent laboratory chow resulted into a progressive weight gain and after 3 months all animals were clearly obese. However, from 3 months, 2 different phenotypes developed. Part of the animals remained normoglycemic, whereas the others, probably due to a different genetic background, displayed a progressively increasing drastic hyperglycemia. In obese animals, plasma insulin levels strongly increased after 3 months of hypercaloric diet, and they further increased during the 9 months study, pointing to the worsening of a well-known and marked state of insulin resistance, which appeared fairly compensated by β cell hyperactivity. Interestingly, evidence has been reported that in these obese normoglycemic animals, resistance to diabetes is conferred by one major gene [12]. By contrast, in the diabetic group, despite an increase in apparent circulating insulin, plasma glucose rose to impressive levels. The extent of hyperglycemia, ascribed to the interplay of environmental factors with several genes [12] also indicates that the similar increase in insulin-like immuno-reactive material was unable to counteract insulin resistance. This is probably due to the fact that, in diabetic Psammomys obesus, a large proportion of circulating insulin consists in fact of proinsulin and split products that are biologically inactive [13]. These high levels of insulin, circulating in vivo, also contrast with the low secretory activity of pancreatic islets in the presence of glucose in vitro. This apparent paradox probably results from differences in pancreatic islets environment. The latter, much more complex in vivo, probably masks the well known impairment of pancreatic β response to glucose that occurs with the gradual loss of glucokinase and GLUT2 glucose transporter [14]. It must also be mentioned that the plasma lipid profile is modified; indeed, both circulating triglycerides and cholesterol are markedly increased in the 2 groups with a much higher rise in the diabetic group; these data confirm previous ones obtained by others, also reporting that both insulin resistance and diabetes up-regulate the hepatic machinery involved in intracellular VLDL assembly [15]. Taken together, all the abnormalities we observed in Psammomys obesus after a prolonged high caloric diet resemble those occurring during the natural history of type-2 diabetes in humans, and especially in PIMA Indians, as a consequence of life style modifications [16] together with a specific genetic susceptibility.
It is now well established that obesity and insulin resistance are associated with a low-grade systemic inflammation related to adipose tissue expansion and where the expression of proinflammatory cytokines, including IL-1β, is increased [17,18] probably as a result of an increase in macrophage population in this tissue [19,20]. Hence, IL-1β has been considered as a valuable target to address insulin resistance. Indeed, immuno-neutralization of IL-1β with a high affinity monoclonal antibody induces beneficial therapeutic effects on the prevention of diabetes-related traits in hyperglycemic mice with diet-induced obesity [21]. On the same line, IL-1 Receptor antagonist (IL-1Ra) is an anti-inflammatory cytokine also produced by white adipose tissue and the pancreas [22] that competitively binds to the IL-1 receptor [23]. Interestingly, a recent study showed that blocking IL-R1 with human recombinant IL-Ra improved glycemic control and β-cell function and reduced markers of systemic inflammation in obese and non-obese subjects with type-2 diabetes [24].
The discordant data resulting from studies on the expression and the effects of IL-1β directly at the β cell level make it impossible to draw definite conclusions. IL-1β gene and protein expression have been reported in isolated islets from type 2 diabetic donors and in islets from healthy donors upon exposure to high glucose during 72 hours [25,26]. These observations could not be confirmed in other studies [27]. Likewise, in islets from hyperglycaemic Psammomys obesus, immuno-histochemical studies using a rodent specific antibody, pointed to the expression of IL-1β after one week of high carbohydrate diet [28] which was not found in more recent studies by RT-PCR experiments with gerbil specific primers and by electron microscopy with immuno-gold staining [29] in the same diabetic prone Psammomys obesus line after a 1 and 3-week high energy diet. Therefore, that IL-1β could be implicated in the decrease of β-cell mass remains a matter of debate, all the more since IL-1β has been shown to exert a dual effect; indeed, exposure of human islets to low IL-1β concentrations stimulates β-cell proliferation and decreases apoptosis, whereas increasing amounts had the reverse effects [30].
Likewise, concerning insulin secretion, IL-1β is known to induce a bimodal effect on β-cell function: a stimulating or a suppressive effect depending on IL-1β concentration, duration of exposure and glucose concentration [31,32]. The secondary inhibitory phase is known for a long time [33] and is accompanied by decreases in oxidative metabolism and calcium uptake [34] secondary to nitric oxide (NO) production after induction of the inducible isoform of NO Synthase (iNOS) [35,36]. At the opposite, the initial stimulatory effect of IL-1β has been shown to be glucose-dependent and related to diacylglycerol formation and stimulation of PKC [37].
Our study brings evidence for the first time that, in addition to mice and rats, IL-1β exerts an acute stimulating effect in a desert rodent belonging to the gerbil subfamily. This effect is concentration-dependent with a maximum already obtained at 5 UI/mL. It must be emphasized that Psammomys obesus appears to be more sensitive and responsive to the stimulating effect of IL-1β than the Wistar rat. Indeed this cytokine is already effective at 1UI/mL, when no significant effect is observed in Wistar rats. Furthermore the maximal stimulation reaches +62 and +125% of basal values in respectively Wistar and sand rats. The reason for such an interspecific difference remains to be determined. More interestingly, this effect is retained after a 9-month hypercaloric diet in obese normoglycemic, but not in diabetic animals. The absence of effect in type-2 diabetic gerbils could be related to the drastic decrease in insulin stores known to occur in these animals. However a decrease in IL-R1 expression/activity cannot be excluded, since the biologically inactive insulin-like immuno-reactive material is totally irresponsive to IL-1β. In contrast in diabetes-resistant animals, glycemia remains normal thanks to the progressively increasing circulating insulin that compensates for insulin resistance. Persistence of normoglycemia throughout the very long period of high caloric diet in this genetically low insulin-responsive animal is remarkable, as is also the ability of β-cells to respond to an acute challenge with IL-1β. As specific gerbil antibodies are not available to estimate circulating levels of the cytokine, it is difficult to propose that IL-1β could at least partly account for β-cell hyperactivity in diabetes-resistant animals. Nevertheless, the IL-1β system is very complex and such an effect cannot be excluded, all the more since IL1-R1 deficient mice exhibit late onset obesity accompanied by insulin resistance and decreased glucose tolerance [38].
At present no data are available concerning the effect of IFN-γ on pancreatic β-cell; our study points to the absence of any significant acute effect of the cytokine on insulin secretion in both Wistar rat and Psammomys obesus. That IFN-γ intervenes in the pathogenesis of insulin resistance and type-2 diabetes remains to be determined. Leukocytes isolated from obese or type 2 diabetic patients display a defective IFN-γ production upon stimulation with IL-18 [39] and blood concentrations of IFN-γ were found unchanged in obese women [40]. The prototypical T-helper cytokine has however been proposed to be involved in the regulation of inflammatory response that occurs in obesity. Indeed, obese IFN-γ deficient mice present a decrease in adipose tissue expression of mRNA encoding inflammatory genes and also an improvement of glucose tolerance [41].
To conclude, switching the natural low caloric diet of Psammomys obesus to a high energy one provokes a marked body weight gain with the appearance after 3 months of 2 distinct phenotypes, i.e. obesity associated with either normoglycemia or type 2 diabetes. Throughout the 9 months of high caloric diet-induced low-grade inflammation state, insulin resistance is remarkably compensated by an increase in β-cell activity in normoglycemic animals. Pancreatic islets of Psammomys obesus are highly sensitive to IL-1β and the acute stimulating effect of this cytokine is preserved after 9 months in these obese diabetes-resistant desert rodents; we propose that IL-1β is a factor involved in pancreatic β-cell hyperactivity that compensates for developing insulin resistance in these animals.
Disclosure of interest
The authors declare that they have no conflicts of interest concerning this article.