1 Introduction
Cameraria ohridella (Deschka & Dimič) is a polyvoltine specialist mining moth which has rapidly spread to many European countries [1,2]. C. ohridella as the pest of the white horse chestnut (Aesculus hippocastanum L.), causing the premature loss of leaves in adult trees, has been recognized more esthetically than economically important [3]. The shortened period of photosynthesis of infested trees causes a reduction of seed quality, but not in seed quantity and the death of trees has been rarely observed [4].
During the last 20 years of C. ohridella infestation, many activities were concentrated on searching for effective tools that could help in control of the species. The use of insecticides is a difficult task for many reasons, such as the recognized partial tolerance of C. ohridella larvae to plant allelochemicals [5,6]. Specific feeding behaviour of the species reduces the use of chemical spraying, and requires the use of systemic pesticides. They might also affect other species associated with horse chestnut trees [2]. Sex pheromones have been also widely studied and tested, but their application is not common [7–9].
Trees are sensitive to various stressing factors, such as periodic water deficiency causing earlier leaf wilting and yellowing, mineral imbalance, temperature oscillations or anthropogenic contaminants. Moreover, intensive mining activity reduces photosynthetic capacity of the host trees and increased with time amounts of secondary metabolites reduce the quality of leaves for feeders [4,5,10]. All these factors may act negatively on larval development [11–13]. Direct or indirect effects of stressors on insects are seen at first as disturbances of the cellular homeostasis [14]. Insects are generally well prepared to cope with chemical stressors by integrating available protective systems. Also, stress proteins, mixed-function oxygenases, and antioxidative system play an important role as the subsystems in the defence against chemicals [14–17]. In the case of C. ohridella, this depends on specific temporary physiological adaptations, expressed by the induction of specific protective mechanisms, involving enzymatic detoxification of water-insoluble toxic substances [14,17,18]. When effects caused by chemicals are not controlled properly, a series of unwanted changes might happen, including inhibition of enzymes, unbalanced redox state or conformational changes of functional proteins [14,18].
Recognition of intrinsic possibilities of C. ohridella to cope with oxidative stress and the enzymatic detoxification of chemicals is important for adequate planning of the chemical control of this pest. Despite some earlier assays done for biosystematic purposes, the data on metabolic processes and detoxifying abilities of the horse chestnut leaf miner are lacking [19]. The quality of food is the limiting factor and trade-off mechanisms decide whether more energy is allocated to detoxification at the expense of prolonged larval development. This forces the organisms to start earlier the breeding phase [20]. Seasonal decline in foliage suitability for C. ohridella larvae suggests that the profiles of detoxifying enzyme activities might differ between generations appearing during the year [4,5].
This study aims to quantify detoxifying abilities of larvae from three generations within a vegetation period, and, moreover, to check how consistent are their enzymatic activity during three consecutive years. The food quality depends also on the age of the host tree. Older trees are usually better prepared to fight with the potent grazers and produce more secondary metabolites [4,5,13]. We also examined to what extent the age-related host plant condition may influence the enzymatic response of C. ohridella larvae. Another task was to compare detoxifying abilities of C. ohridella on the basis of data obtained in studies on other lepidopteran species.
Functionally, the intestinal cells are in the first line of chemical attack linking external and internal milieu [21]. The last actively feeding larvae consume the largest amounts of the leaf parenchyma and could be easily recognized inside the damaged part of the leaf. This developmental stage was chosen for experiments, and a target organ was the midgut. This stage is large enough to collect a sufficient number of larvae for enzymatic analysis without disturbing fitness of the host trees.
Activities of six enzymes in the midgut were assayed. The extent of inhibition of acetylcholinesterase (ACHE) was considered to be the most valuable biomarker of exposure to organophosphate and carbamate pesticides [22,23]. In insects, carboxylesterases (CarE) are important in catalytic hydrolysis of carboxyl esters including organophosphates or pyrethroids [24]. Plant allelochemicals may up regulate CarE genes that may lead to higher resistance to insecticides rich in ester bonds [25]. Glutathione-S-transferase (GST) and Se-independent glutathione peroxidase (GSTPX) form the second pair of selected target enzymes and are closely related with the cellular metabolism of glutathione. The final products of conjugation with GSH are more polar and more easily eliminated from the cell. The level of secondary metabolites of the host plants correlates well with the induction rate of GST [26]. Various GST isoenzymes might also play an important role in acquired resistance to pesticides [27]. GSTPX functionally neutralizes organic prooxidants in the cell, playing the functional role of glutathione peroxidase [28]. The increase in GSTPX activity in insects is often considered as the adaptive mechanism, protecting against environmental pollutants [29]. Superoxide dismutase (SOD), catalase (CAT), and GSTPX are at the first line of antioxidative protection of the organism. Induction of the enzymes involved in the elimination of free radicals can provide evidence for the presence of oxyradicals, and some indication of their cellular concentrations [23].
In a parallel study, digestive capability of the last instar C. ohridella larvae from the same sites using the same material was also investigated [30].
2 Material and methods
2.1 The areas and the object of study
Two sites were selected in urbanized areas of Southern Poland: Chorzow (50°17′24″N,19°58′17″E), and (2) Czeladz (50°18′36″N,19°04′17″E). Chorzów is located in a large park with the 15-year-old horse chestnut trees infested by C. ohridella. Czeladz is a green square in urbanized area of the small town with about 30-years-old horse chestnut trees infested by C. ohridella. The initial stage of the leaf miner infestation was similar in both sites. Presence of C. ohridella in the second site was recorded at least 6 years before the experiments had started. Raking and removal of fallen leaves with diapausing pupae was performed only at the first site. Insects were collected from trees untreated with pesticides.
The rate of development and the overall number of nonfeeding instars depend on seasonal weather and climate changes. The pupa has the obligatory diapause and overwinters in litter created by the fallen leaves of the host trees. Moths emerge in the next spring [3,31]. Total life cycle lasts 6 to 11 weeks and generations (usually three annually) may partially overlap [2,32]. Most of the first generation larvae appear usually in May. The second generation is observed from the middle of July to the middle of August. The final one develops in September and October, depending on local weather conditions.
2.2 Sample collection and preparation
Functionally, the intestinal cells are in the first line of chemical attack linking external and internal milieu [21]. The last actively feeding larvae consume the largest amounts of the leaf parenchyma and could be easily recognized inside the damaged part of the leaf. This developmental stage was chosen, and a target organ was the midgut. They are large enough to collect a sufficient number of larvae. The quantity of biological material for laboratory assays of enzymes had to be reduced to minimal necessary replicates supporting statistical needs, minimizing the impact upon the fitness of the host trees by experimental defoliation.
Leaves with larvae were collected three times annually at about 4 weeks intervals, from the end of June to September, synchronized with the appearance of the three generations of the insects. Usually 1000 individuals were sampled from each site. Leaf samples were taken from four trees at each sampling plot. Leaves containing visible irregular mines, 30 to 40 mm long, with actively feeding L4 caterpillars inside, were excised and taken to the laboratory. Then, larvae were carefully removed from the mines and kept for some hours on wet lignite in order to remove their gut contents. Digestive tracts were dissected in 1.15% KCl under stereomicroscope from larvae immobilized on ice. Midguts were isolated and remains of the gut contents were washed out. Each sample consisted of 10 midguts was gently homogenized in 1.0 ml of a solution containing 0.1 M sodium-phosphate, 1 mM EDTA and 10% glycerol, centrifuged twice for 10 min at 3000 × g at 4 °C and the supernatant was centrifuged at 9000 × g. Obtained supernatant was ultracentrifuged at 100,000 × g for 1 h. A cytosolic fraction was stored for further biochemical examinations in Eppendorf tubes in medium at –70 °C.
2.3 Enzyme assays
Acetylcholinesterase (AChE) [EC 3.1.1.7] activity was assayed as in Scaps et al. [33], using acetylthiocholine iodide (AChI) as a substrate in a mixture solution of 0.05 M phosphate buffer, pH 7.4; 0.001 M 5,5 dithio-dinitrobenzoic acid (DTNB) in phosphate buffer pH 8.0 and a supernatant. Samples were incubated for 5 min. The rate at which AChI was hydrolyzed by AChE was measured at 410 nm at 25 °C. The results were calculated using extinction coefficient of 13,600 M/l/cm and expressed as nmol AChI/min/mg protein.
Carboxylesterase (CarE) [EC 3.1.1.1] activity was determined spectrophotometrically according to van Asperen [34], with α-naphtyl acetate (α-NA) as a substrate which reacts with o-dianizidin, and the changes of absorbance were measured at 600 nm with a spectrometer Helios Epsilon (UNICAM). The reaction was measured in a solution containing 0.05 M phosphate buffer, pH 7.4, a supernatant and 1.5 μM solution of α-NA dissolved in acetone. After 10 min of incubation, the reaction was stopped with a mixture of o-dianizidin with a sodium laurate sulphate. Colour intensity was measured after 15 min. Activity was calculated on the basis of α-naphtol standard curve corrected to the blank sample [35]. Specific activity of CarE was expressed as nmol of α-naphtol/min/mg protein.
Glutathione-S-transferase (GST) [E.C. 2.5.1.18] was assayed by measuring the rate of conjugation of 1-chloro-2,4-dinitrobenzene (CDNB) with glutathione as the substrate [36]. Changes in absorbance were recorded at 340 nm. The reaction mixture was 0.05 M phosphate buffer, pH 7.4, 5 mM reduced-glutathione, a supernatant and 15 mM CDNB dissolved in 96% ethanol. An increase of absorbance was recorded at 340 nm for 5 min. Enzymatic activity was expressed in terms of nmol of CDNB/min/mg protein.
Selenium-independent glutathione peroxidase (GSTPX) [EC 1.6.4.2] was determined spectrophotometrically, according to Simmons et al. [37], measuring the rates of hydrogen peroxide or cumine oxide reduction. The presence of nicotinamide adenine dinucleotide phosphate (NADPH) and glutathione reductase is necessary to maintain a constant level of glutathione. Sodium azide was used as an inhibitor of catalase present in a supernatant. A reduction of absorbance at 340 nm caused by oxidation of NADPH per minute and per milligram protein was calculated with the extinction coefficient for NADH = 6.22 × 103/(m × cm) and 1IU = 1 nmol NADPH/min/mg protein [38]. Results were expressed in nmol GSH/min/mg protein. The reaction mixture contained: 0.05 M phosphate buffer, pH 7.2; 2 mM EDTA; 2 mM NaN3; glutathione reductase (6 AU/ml H2O); 10 mM glutathione reduced (GSH); 2.5 mM NADPH and a supernatant. The reaction was started by the addition of a 15 mM water solution of cumine oxide (cumOOH).
Catalase [EC 1.11.1.6] CAT activity was measured at 240 nm in a mixture containing 0.05 M phosphate Sørensen buffer, pH 7.4, supernatant and 10 mM H2O2 according to the method of Aebi [39]. In the blank sample 0.5 ml H2O was replaced by H2O2. Readings were done for 30 s on UV–VIS CECIL 3000 spectrometer, using two chambers simultaneously with a blank and experimental sample. The enzyme activity was expressed in μmol H2O2/min/mg protein.
Superoxide dismutase (SOD) [EC 1.15.1.1] was determined according to the Misra and Fridovich method based on a spontaneous autooxidation of adrenaline to adrenochrome [40]. Adrenaline autooxidation is a source of superoxide radicals inhibited by SOD. Enzyme activity was measured in two steps in the mixture 0.05 M Na2CO3/NaHCO3 buffer, pH 10.2 with 0.1 M HCl, (pH 2) containing epinephrine (10 mM adrenaline solution in 0.01 M HCl). In the first step the rate of epinephrine autooxidation was established at 480 nm using UV–VIS CECIL 3000. The absorbance rate for 0.33 mM epinephrine solution without SOD is equal to 0.025 absorbance units per minute. In the second step, the volume of the sample that causes a 50% inhibition of adrenaline autooxidation to adrenochrome was established in a series of samples from 10 to 100 μl. Validation of correctness of the assay was done with a commercial SOD standard from bovine erythrocytes (Sigma, S-2515). The activity unit of Misra and Fridowich is defined as the amount of enzyme that inhibits 50% of the control reaction of epinephrine autooxidation/min/mg protein.
All enzymatic assays with the same sample were repeated twice. Measurements were done at a temperature of 25 °C. Protein concentration was determined by Bradford method [41] using bovine albumin as the standard.
2.4 Statistical analysis
The data were analysed using STATISTICA 9.0 packages. The Q Dixon test was performed in order to remove extreme results [42]. The data were tested for equality of variance and normality with Shapiro-Wilk test. If the results showed normal distribution they were subdued to one and two-way ANOVA analysis. The homogeneity of variance was tested with Levene's and F Fisher's test. For data not showing the homogeneity of variance, the post hoc LSD test was applied. Results were considered significant at P < 0.05.
3 Results
The profile of AChE activity was dependent on site and the year of study (Figs. 1a and b). During the first year of study (2004), a significant inhibition was stated in the second generation of larvae living in leaves of older trees (Czeladz) (P1–2gen = 0.006 and P2–3gen < 0.0001). Differences between the first and third generation were insignificant. In the second year of study, a similar pattern of generation-dependent AChE activity was observed (P1–2gen < 0.0001; P1–3gen< 0.0001 and P2–3gen < 0.0001). In the third year (2006), AChE activities were similar in insects of the first and second generation, but they were lower than in the last generation (p1–3gen < 0.0001 and P2–3gen < 0.0001) (Fig. 1a). A quite different pattern of AChE activity was noted in larvae collected from younger trees (Chorzow). The year of the study appeared also a significant factor. During the first year, AChE activity in larvae from Chorzow population rose steeply in consecutive generations (P1–2gen = 0.006; P1–3gen < 0.0001 and P2–3gen < 0.0001). There were no differences in AChE activity between generations in the second year. In the last year, a decrease of AChE activity was recorded in the third generation (P1–3gen = 0.008 and P2–3gen = 0.003 (Fig. 1b).
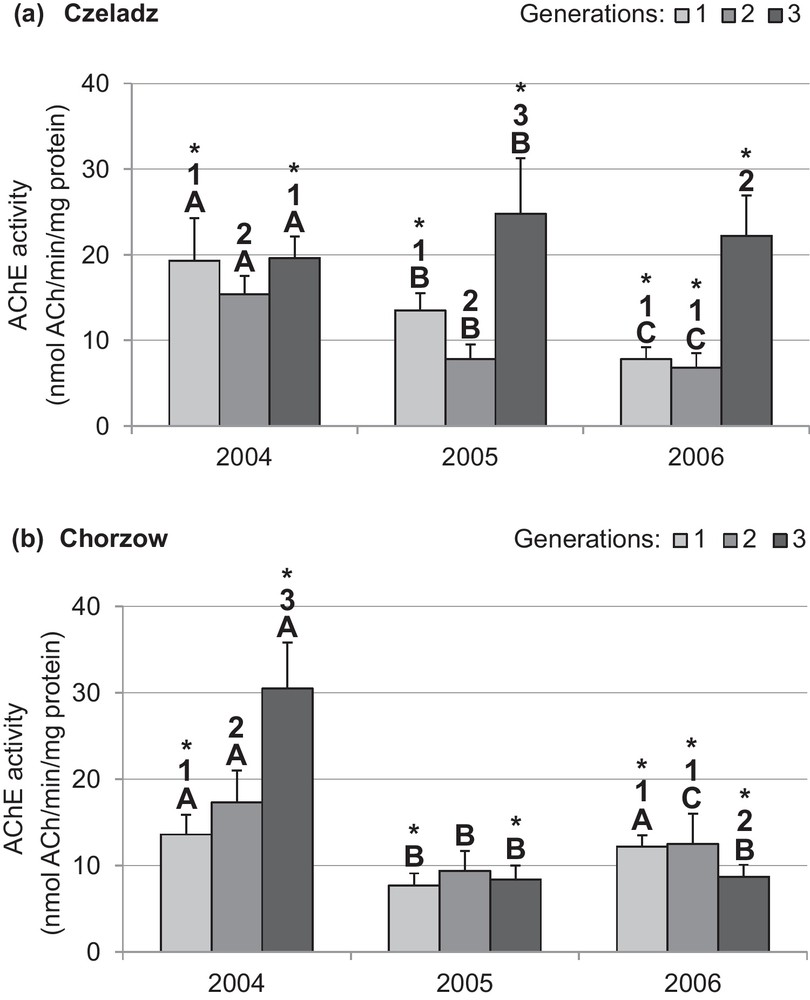
Activity of acetylcholinesterase (mean ± SD expressed in nmol Ach/min/mg protein) in the midgut of Cameraria ohridella larvae from three generations collected during 3 years of study at two localities: Czeladz (a) and Chorzow (b) ANOVA/MANOVA, LSD test; P < 0.05; n = 7–14. Explanations: asterisks indicate significant differences between localities within a generation and year; different letters (A–C) between the years of study within generations and localities; different numbers (1–3) between generations in a given year and locality.
Data of CarE activity were compared only between the second and third year (Figs. 2a and b). The data for the year 2004 were rejected because a part of samples was destroyed. The CarE activity in larvae collected in 2005 from both localities was higher than in 2006. There were site-dependent differences in the activity of that enzyme in 2005, and its activity was higher in larvae collected from older trees in Czeladz. The CarE activity of Czeladz population was in 2005 more than two times higher than in the next year. For 2005, the differences were indicated between the third and earlier generations (P1–3gen = 0.02; P2–3gen = 0.019) (Fig. 2a). In Chorzow population, CarE activity of the first generation larvae was lower in comparison with the second (P = 0.034) and the third generation (P = 0.003). Effects of generation and site for the year 2006 were insignificant (Fig. 2b).
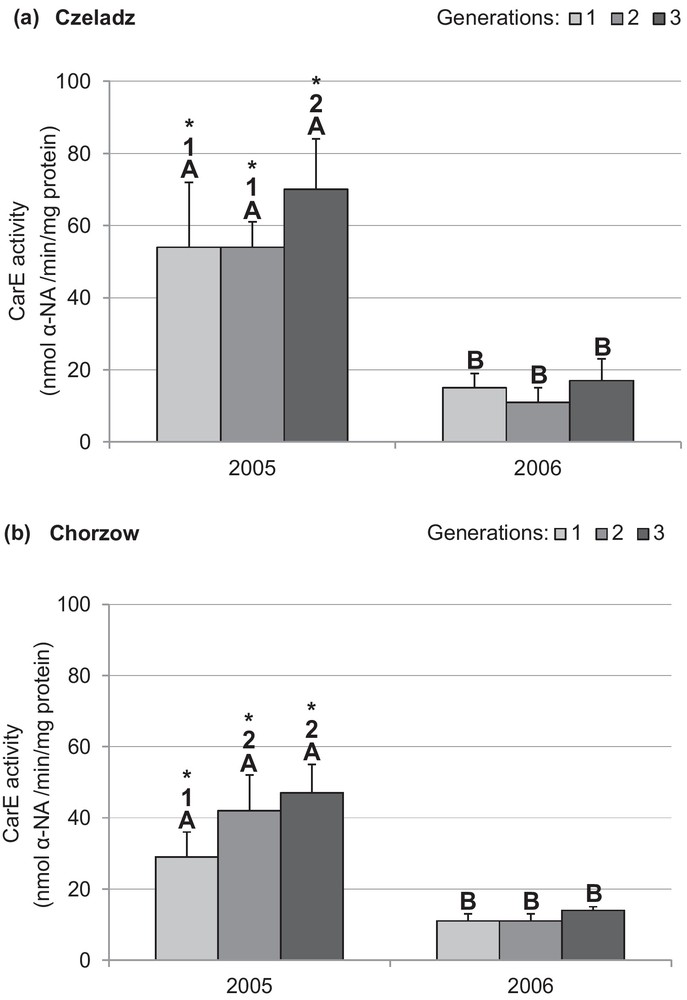
Activity of carboxylesterase (mean ± SD expressed in nmol α-NAmin/mg protein) in the midgut of Cameraria ohridella larvae from three generations collected during 2 years of study at two localities: Czeladz (a) and Chorzow (b). ANOVA/MANOVA, LSD test; P < 0.05; n = 7–12. Explanations: asterisks indicate significant differences between localities within a generation and year; different letters (A–B) between the years of study within generations and localities; different numbers (1–2) between generations in a given year and locality.
GST activity in larval midgut was year-, generation- and site-dependent (Figs. 3a and b). During the first year, the enzyme activity pattern in larvae from both sites was similar. The second and third generations of larvae had higher GST activity than indicated in the midgut of the first generation. The effect upon the generation in Czeladz was insignificant both in the year 2005 and 2006 (Fig. 3a). In Chorzow population, GST activity decreased over the season of 2005 (P1–3gen = 0.003). In the third year, GST activity of the third generation larvae from Chorzow was lower than stated in the former generation (P2–3gen = 0.033) (Fig. 3b).

Activity of glutathione-S-transferase (mean ± SD expressed in μmol CDNB/min/mg protein) in the midgut of Cameraria ohridella larvae from three generations collected during 3 years of study at two localities: Czeladz (a) and Chorzow (b). ANOVA/MANOVA, LSD test; P < 0.05; n = 7–14. Explanations: asterisks indicate significant differences between localities within a generation and year; different letters (A–C) between the years of study within generations and localities; different numbers (1–2) between generations in a given year and locality.
The activity of GSTPX was site-dependent, being higher in larvae collected from older trees in Czeladz in 2004 and 2005 (Figs. 4a and b). In the first year, the GSTPX activity decreased in consecutive generations of larvae from both sites: Czeladz: P1–2gen = 0.003; and P2–3gen < 0.0001; Chorzow: P1–3gen = 0.012 and P2–3gen = 0.034. A slightly different pattern was stated for 2005 when in larvae from Czeladz GSTPX activity was kept on the high level for two generations and diminished in the last generation (P1–3gen = 0,001; P2–3gen = 0.004) (Fig. 4a). In Chorzow, low activity characterized the first generation and later the activity of the second and third generation reached higher levels (P1–2gen < 0.0001; P1–3gen < 0.0001) (Fig. 4b). In the last year of study (2006), GSTPX activity was generally lower than in former years. In Czeladz, the activity increased with time (P1–3gen = 0.004), while in Chorzow a bell-shape GSTPX activity pattern was indicated (P1–2gen = 0.0001; P2–3gen = 0.002).
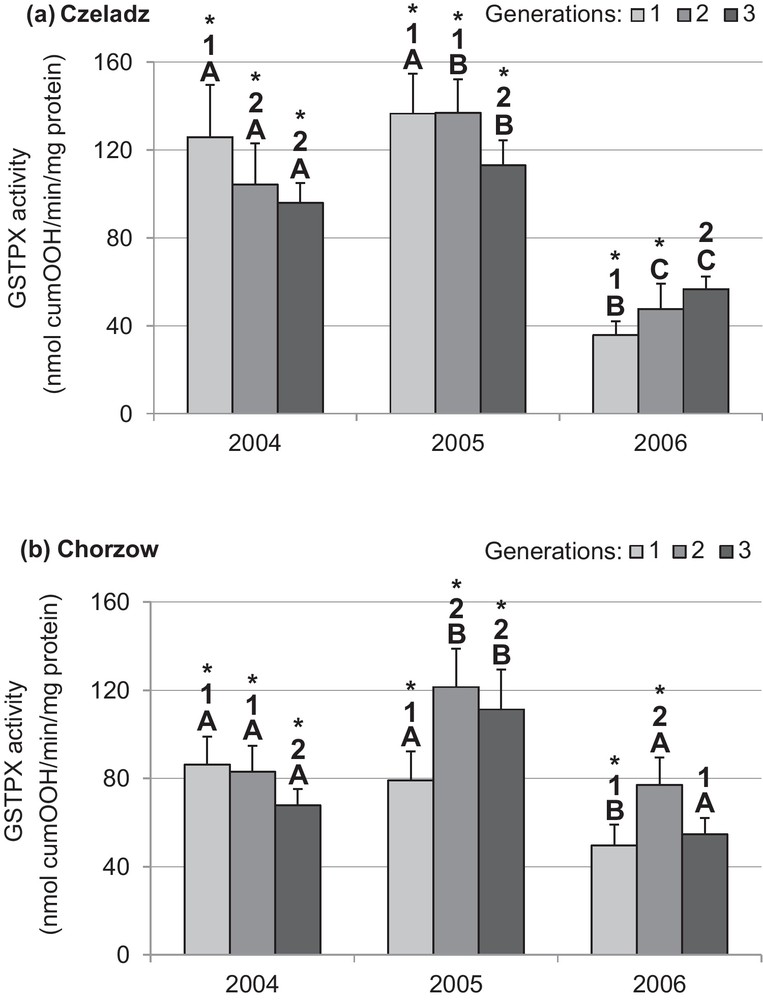
Activity of selenium-independent glutathione peroxidase (mean ±SD expressed in nmol cumOOH/min/mg protein) in the midgut of Cameraria ohridella larvae from three generations from three generations collected during 3 years of study at two localities: Czeladz (a) and Chorzow (b). ANOVA/MANOVA, LSD test; P < 0.05; n = 7–14. Explanations: asterisks indicate significant differences between localities within a generation and year; different letters (A–C) between the years of study within generations and localities; different numbers (1–2) between generations in a given year and locality. Masquer
Activity of selenium-independent glutathione peroxidase (mean ±SD expressed in nmol cumOOH/min/mg protein) in the midgut of Cameraria ohridella larvae from three generations from three generations collected during 3 years of study at two localities: Czeladz (a) and Chorzow (b). ANOVA/MANOVA, ... Lire la suite
CAT activity in larvae collected from older trees in Czeladz was gradually decreasing in consecutive years, but the effect of generation was insignificant (Fig. 5a). In larvae from younger trees in Chorzow, the CAT activity in 2004 progressively decreased: P1–2gen = 0.004; P1–3gen < 0.0001; P2–3gen < 0.0001. During the second year, the larvae from Chorzow had the lowest activity in the first generation (P1–2gen = 0.005; P1–3gen < 0.0001). There was no effect of generation on CAT activity in larvae from this site in the last year of study (Fig. 5b).
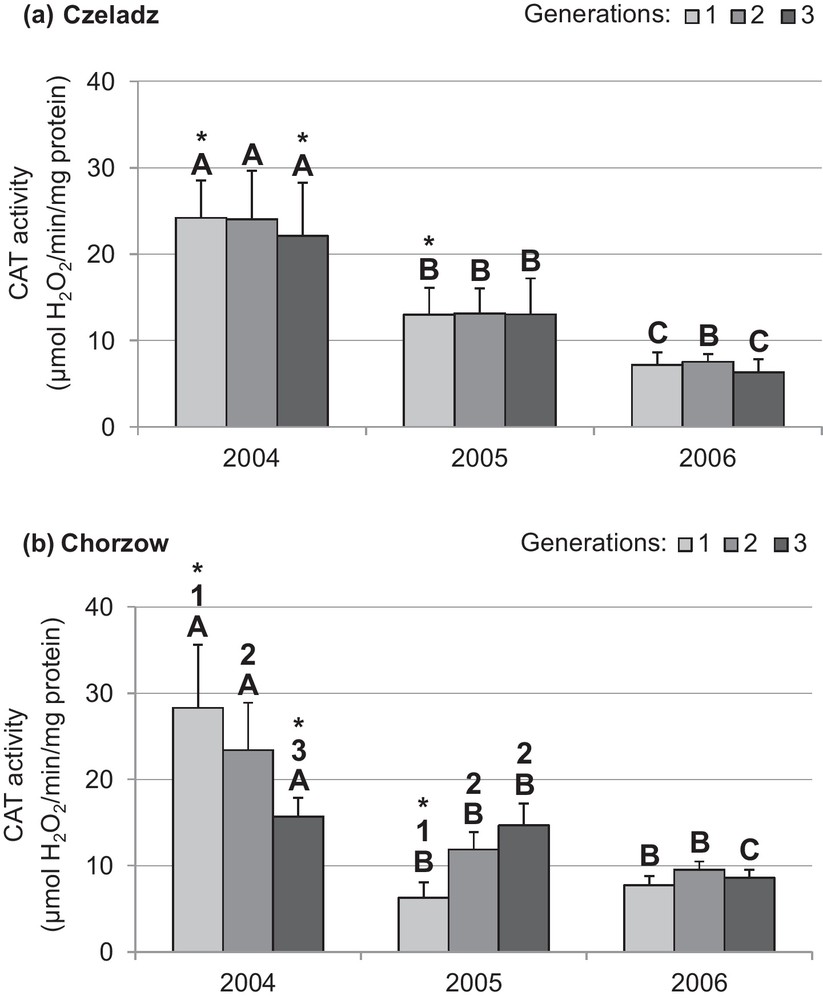
Activity of catalase (mean ± SD expressed in mol H2O2/min/mg protein) in the midgut of Cameraria ohridella larvae from three generations from three generations collected during 3 years of study at two localities: Czeladz (a) and Chorzow (b) ANOVA/MANOVA, LSD test; P < 0.05; n = 7–14. Explanations: asterisks indicate significant differences between localities within a generation and year; different letters (A–C) between the years of study within generations and localities; different numbers (1–2) between generations in a given year and locality.
SOD activity was site-dependent in 2004 and 2005 (Figs. 6a and b). In both years, the second generation larvae of the Czeladz population had the highest SOD activity. In 2006, the activity of SOD was kept at low levels in all generations, and the effects upon that generation were insignificant. In the larvae from Chorzow, the generation-dependent pattern of activity was bell shaped, with the highest levels in the second year. During the first year, the lowest SOD activity characterized the first generation (P1–3gen = 0.021; P2–3gen = 0.009). The effect of generation on SOD activity in larvae from this site was proved only between the second and third generation (P2–3gen = 0.0002). An opposite pattern was indicated in activity of the last year larvae (P1–3gen < 0.0001; P1–3gen = 0.015).
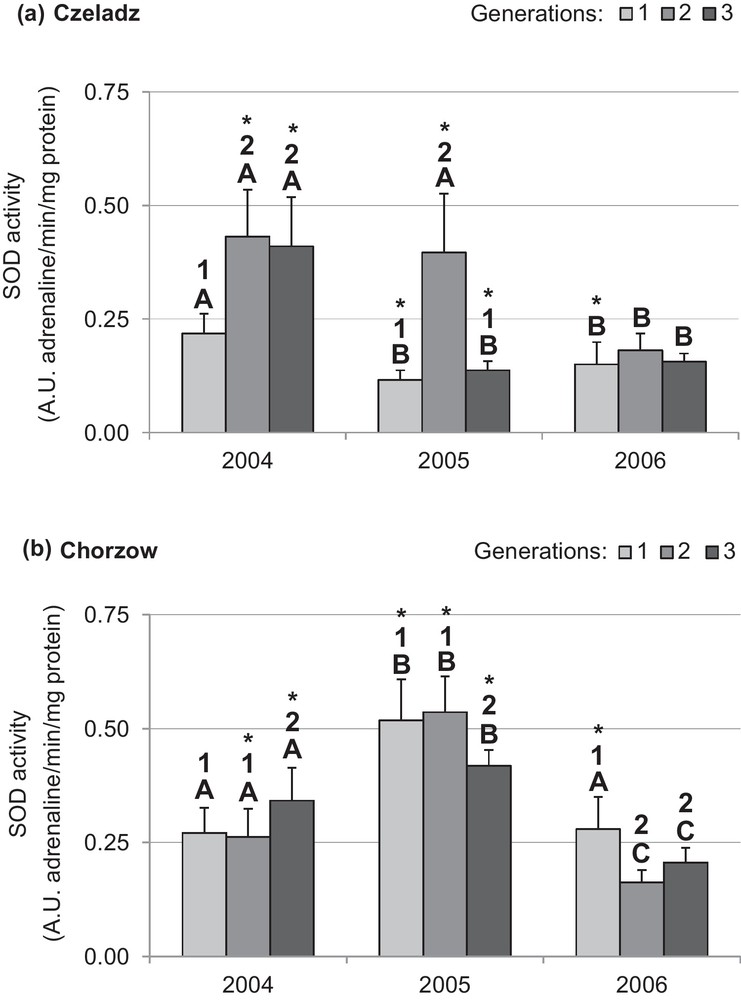
Activity of superoxide dismutase (mean ±SD expressed in nmol adrenaline/min/mg protein) in the midgut of Cameraria ohridella larvae from three generations collected during 3 years of study at two localities: Czeladz (a) and Chorzow (b). ANOVA/MANOVA, LSD test; P < 0.05; n = 7–14. Explanations: asterisks indicate significant differences between localities within generation and year; different letters (A–C) between the years of study within generations and localities; different numbers (1–2) between generations in a given year and locality.
4 Discussion
Studies on insects collected in natural conditions give a possibility to apprehend intrinsic detoxifying abilities expressed by the activity levels of enzymes involved in various metabolic pathways. Enzymatic responses demonstrate the overall effects of various interactions between natural or anthropogenic environmental stressors. They are reflected in higher variability than that observed under controlled laboratory experiments [18].
The collected data demonstrate a wide spectrum of expected variability in the background level of analysed enzymes in the midgut of the examined C. ohridella larvae. The long term study allowed comparing profiles of enzymes that participate in antioxidative and detoxifying processes in relation to three main factors: (i) generation; (ii) site; and (iii) annual differences.
- (i) During this study C. ohridella had three generations annually. The success of the first generation depends on the number of overwintering pupae that emerge into adults. Most of them were from the last generation of the previous year [2]. Fitness of the first and second generation and their reproductive efficiency decide on the abundance, density and the number of pupae that enter the winter diapause and spread of a local population [2,4].
- (ii) Site related differences. This factor includes mainly effects dependent on age differences between the host trees is two selected sites. Moreover, different treatment with fallen leaves containing pupae of the overwintering insects might have a weak effect on population demography. The age of the host tree decides on possibilities of its self-protection against possible invaders realized mainly through production of the secondary metabolites [40]. Effects of other natural and man-made environmental stressors could be postponed because both sites were under similar pressure of industrial pollution and climatic conditions were similar. The removal of fallen leaves and their destruction reduce the population to a relatively low level [43,44].
- (iii) Consecutive years differ in fluctuations of important climatic factors [45] during the period of active larval development of C. ohridella and resulted in time dependent effects on enzyme activity
4.1 Effect of generation
With the exception of AChE, activities of other studied enzymes were the highest in the second generation. Among digestive enzymes studied on the same material also the second generation larvae had the highest activities of important glycolytic enzymes – α-amylase and maltase. Other studied enzymes did not show a significant generation related trends [30]. One of the reasons explaining differences in generation-dependent enzyme activity changes are reduced amounts of photosynthetically active tissue causing a severe defoliation of the horse chestnut trees. Such effects are common in the summer [3,30]. The first generation of C. ohridella usually exploits the lowest branches of the tree. Then, due to limited food resources, next generation starts the intra-plant dispersal and the third generation is observed in the top of the trees [4,11,44]. Long-distance migration is only in crowded conditions of the third generation moths [44]. The response of the tree to leaf injuries is enhanced with time production of secondary metabolites [46]. This mobilizes insects to counteract increased toxicity of consumed food [47] and probably movement upwards of the older generations is to avoid too high concentrations of allelochemicals. Insects developed several strategies to overcome plant defence barriers through metabolization, sequestration or avoidance [14,18,20,35,48,49]. For example, analyses of CAT, SOD and GSTPX activities in S. littoralis kept on natural (potato leaves) and artificial diet indicated higher amounts of prooxidants in natural diet that induced antioxidative response of the larvae [16]. Yu experiments with S. frugiperda larvae proved an important role of GST in the second phase of detoxification processes [50]. These studies confirm an enhancement of activity of antioxidative enzymes observed in the second generation larvae of C. ohridella.
Concentration of allelochemicals in an insect diet decides on stimulatory or inhibitory response of the Mixed Funtion Oxidase (MFO) system [51]. Leaves of the horse chestnut are rich in flavonoids and terpenes. In the case of C. ohridella, their concentrations were probably at the stimulatory phase and were easily eliminated by C. ohridella through a well-functioning MFO system and antioxidative enzymes. Jakubczyk [52] analysed activity of cytochrome reductase b5 and cytochrome P-450 NADPH reductase in three generations of C. ohridella and indicated the lowest value of the first enzyme in the second generation, and no generation-dependent effects on activity of the second one. During the second phenological stage (when seeds start swelling), severely defoliated trees produce less allelochemicals [3–5]. A decrease of enzyme activities of the third generation to the levels similar to that in the first generation seems to be rather an additive effect of various factors. Nutritional quality of the older foliage is lowered, and (in case of high larval density) availability of leaves not injured earlier by consumers is low. Some leaf miners are able to avoid plant defences by selecting the most nutritious parts of the leaf tissue and their average feeding efficiencies are higher in comparison to those of external feeding on oak [10]. We suppose that the trade-off compensatory mechanism in larvae of the last generation switches rather towards activities supporting larval growth and to contend with limited nutrients than to energetically costly detoxification processes [20].
4.2 Effect of locality – age of the host trees
One of the main factors which differentiate sites in our study was the age of trees rendering to differences in chemical composition of consumed leaves. The host trees at Czeladz were twice as old as the host trees at Chorzow. Moreover, fallen leaves in this site were never raked. This means that larvae form Czeladz had to cope with higher concentrations of allelochemicals produced by older trees, but at the same time more leaves were available for oviposition and more pupae could survive winter period. In favoured conditions, higher number of emerging moths could initiate next generation. Differences in distribution and concentration of produced allelochemicals depend also on light intensity, availability of nutrients, place of a given leaf in canopy (in sun or in shade; low, medium, high canopy) or impact of environmental pollutants [53–56]. Site-dependent differences in activities of SOD, GSTPX and CarE between larvae from both localities, resulted probably from other quantitative and qualitative spectra of digested xenobiotics. Enhancement in SOD activity could be a stimulatory effect of higher concentrations of toxic substances produced by older trees in Czeladz that induced dismutation processes finalized by higher activity of GSTPX. Activity of this enzyme was also high in larvae feeding on leaves from younger trees in Chorzow. Insulation at this site was higher than that of Czeladz. Mining insects are more photosensitive than external-feeders [56]. Larvae of Cameraria hamadryadella better survived in the shade than in the sun. Specialist insects living on phototoxic plants might have higher constitutive levels of antioxidant enzyme activity [56–58] and possibly this factor induced GSTPX activity [59]. Stygar et al. [30] showed that energy substrates for C. ohridella larvae are supported mainly by hydrolytic activity of saccharolytic enzymes. Higher activities of disaccharidases (maltase, α- and β glucosidase), stated in larvae living on older trees, were explained by indirect effects of consumed food. High β-glycosidic activity allows insects to cope with β-glucosides and their aglycones, and by reducing their toxicity utilization of food containing higher concentrations of allelochemicals. Moreover, in urbanized areas horse chestnut trees had lower concentration of unsaturated fatty acids and higher level of linolic acid than trees grown in unpolluted area [60]. They also accumulate heavy metals such as Zn, Cu, Pb or Cd [61,62]. These metals were also detected in tissues of C. ohridella larvae collected at the same site as in our study. All these dietary components may directly influence detoxifying abilities of C. ohridella. Metals are known from their prooxidative action [14,22,63]. Chronic exposure of S. exigua larvae for many generations to dietary Zn and Cd induced stimulatory effects at lower metal concentrations and inhibitory effects at higher concentrations on activity of glutathione dependent enzymes – GST, GPOX, GSTPX [64,65]. In our study heavy metal loads were similar in both sites and could not explain differences in enzyme activity patterns. Nevertheless, this factor should not be neglected in any case of planned chemical treatment against the pest.
4.3 Annual differences
Connor and Taverner [10] documented several advantages and disadvantages of being a leaf miner in comparison with external-feeding insects. Leaf-mining insects escape from disease infections of leaf miners is considerably less than that for external-feeders. Leaf epidermis partly protects against UV radiation, and minute larvae are not at risk of being washed out or blown from the surface by a strong wind. Their feeding efficiency is also higher, presumably due to consumption of more nutritious parts than do external-feeding insects. Disadvantage of a partly discrete larval life is reduced mobility. Incidence of reports of attack by predators and parasites on leaf miners is significantly higher than external folivores. Leaf miners may use leaf vibrations to escape ovipositor stings, demonstrated in experiments with the gracillariid moth Phyllonorycter malella and its parasitoid, Sympiesis sericeicornis [66].
Larvae of C. ohridella are sedentary and their development is restricted to one leaf. Feeding inside the leaf tissues in a specific microclimate C. ohridella larvae reduce the effects of desiccation. They are secure as long as the host leaf remains attached. Premature leaf-abscission results in higher mortality. In experimental conditions, day and night internal temperatures of the leaf mines produced by Cameraria hamadryadella) were slightly lower than external leaf-mine temperatures [56]. Due to reduced ventilation, temperature could be even higher than outside. During hot summer days, overheating and desiccation may also increase larval mortality. These, in turn, enhance fitness of the host tree [10,66]. Such fluctuations of the abiotic factors were recorded in consecutive years of studies. According to Mattison et al. [67], leaf miners associated with their host plants should encounter a greater array of chemical defences and this was reflected in different seasonal profiles of CAT, SOD, CarE and AChE activities in the midgut of C. ohridella larvae.
In the first year, the mean temperature during the vegetation season (IV–X) in areas selected for this study was 16.1 °C and monthly precipitation 61.2 mm. During this year, the highest activities of CAT, SOD CarE and AChE were reported. The following year, the temperature was slightly higher (16.8 °C) but monthly precipitation reached as much as 82.3 mm. In the last year, temperature was the highest (18.1 °C) while the rainfall was similar to that in the first year (62.9 mm) [45]. A dry and hot summer reduces the overall fitness of insects [67] and probably in our study indirectly affected enzyme activities recorded in the third year. Weaker phenological condition of their host trees resulted from reduced assimilative capacity, which in turn depends on feeding intensity of its grazers. Annual differences were also indicated in changes of activity patterns of the digestive enzymes of C. ohridella. During the three consecutive years of study a decrease of activity was indicated for maltase, saccharase, trehalase and cellobiase [68].
4.4 Profiles of detoxifying enzymes in the midgut of C. ohridella – comparisons with other lepidopteran species
The selection of midgut as the main target organ for studying changes in enzyme responses has been given in many earlier studies and indicate its functional importance not only in the digestion processes [30,59,68]. As the main organ, the midgut is in the first line of protection against various contaminants transported with consumed food [16,18,22,64,69–71]. Activity of many enzymes, important in detoxification of xenobiotics, express the highest activity in the midgut cell, e.g. activity of CarE in Lymantria dispar larvae [71], CAT in Trichoplusia ni [72] or GST, ACHE, CAT and SOD in Spodoptera exigua larvae [73].
Scarcity of data exists on enzymes involved in detoxifying processes in the midgut of leaf-mining moths. They are restricted to enzymes related to detoxification of specific xenobiotics, mainly pesticides used in insect control and concentrated on induction or inhibition of enzymes in conditions of applied specific stress from chemicals, thus were invalid for direct comparisons [74]. More available are data from the studies of plant-insect chemical interactions. Our comparisons with enzyme profiles in C. ohridella were restricted only to these where similar assays were used and units were comparable or the recalculations of published data were possible (Table 1).
A comparison of data on specific activity of four enzymes in the midgut of Cameraria ohridella and in the midgut of other lepidopteran larvae.
Species | CAT | SOD | GSTPX | GST | Source |
Cameraria ohridella (Gracilariidae) [S] | 9.8 ± 3.6 | 0.27 ± 0.12 | 86 ± 38 | 2.4 ± 1.2 | This study |
Spodoptera frugiperda | 0.28 ± 0.03 | [77] | |||
(Noctuidae) [G] | 0.47 ± 0.06 | [79] | |||
0.69 ± 0.03 | [50] | ||||
S. littoralis [G] | 0.25 | 0.11 ± 0.02 | [16] | ||
S. eridania [G] | 163 ± 30 | 4.26 ± 0.11 | [58] | ||
134 ± 15 | 5.0 | 8.0 ± 0.7 | 0.28 ± 0.01 | [87] | |
Trichoplusia ni | 270 | 4.0 | 41 | 0.2 | [38] |
(Noctuidae) [G] | 292 ± 6 | 2.15 | 1.35 ± 0.3 | [69,79] | |
1.82 | 12.3 | 0.37 ± 0.11 | [87] | ||
Heliothis zea (Noctuidae) [G] | 1.3 ± 0.04 | [79] | |||
H. virescens [G] | 0.47 ± 0.1 | [79] | |||
Anticarsia gemmatalis (Erebidae) [G] | 0.07 ± 0.01 | [79] | |||
Lymantria dispar (Lymantriidae) [G] | 0.21 ± 0.17 | 6.75 ± 0.65 | 4.8 ± 0.6 | 0.25 ± 0.16 | [13] |
Orygia leucostigma (Crambidae) [G] | 103 ± 19 | [70] | |||
Ostrinia nubilalis (Crambidae) [G] | 33.6 ± 3.7 | 1.4 ± 0.1 | [59] | ||
Manduca sexta (Sphingidae) [G] | 153.9 ± 16.7 | 1.5 + 0.2 | 17.3 ± 1.0 | [59] | |
Anaitis plagiata (Geometridae) [S] | 171.1 ± 14.0 | 1.3 ± 0.3 | [59] | ||
Papillio polyxenes (Papilionidae) [S] | 1099 ± 51 | 4.29 ± 0.07 | 18.3 ± 0.7 | 1.66 ± 0.04 |
[26]
|
1.8 ± 0.08 | [57] | ||||
P. cresphontes [S] | 994 ± 27 | 3.38 ± 0.22 | 42.5 ± 2.2 | 0.44 ± 0.02 | [26] |
P. glaucus [S] | 73.3 ± 8.2 | 0.4 ± 0.49 | [24,26] |
Inhibition of AChE activity is one of the basic biomarkers allowing toxicity assessment of organophosphate and carbamate pesticides in insects and indirectly effects of other xenobiotics including heavy metals [18,22,75]. Among external-feeders the mean midgut activity of AChE in C. ohridella (14.2 ± 7.2 nmol/min/mg protein) indicate its good protective capacity against organophosphate or carbamate insecticides suggesting low efficiency of these compounds against these insects. For example, Kanga et al. [76] reported slightly lower values (8.6–12.9 nmol/min/mg protein) in the adult tortricid moth Grapholita molesta, while in unexposed to insecticides heads of the generalist fall armyworm, Spodoptera frugiperda the activity reached 26 nmol/min/mg protein [75].
In herbivore insects, carboxylesterases play a significant role in decomposition of secondary metabolites produced by the host species. Their level depends on species sensitivity to a specific compound and expresses a wide range of variability [17,24,59]. C. ohridella places among other insect species expressing very low esterase activity. Mean CarE activity of C. ohridella measured against α-naphtol (31.2 ± 6.9 nmol/min/mg protein) was a bit higher than reported in S. frugiperda (22.3 ± 2.7 nmol/min/mg protein) [77] and much lower than reported in other lepidopteran larvae, showing low sensitivity to allelochemicals: 1.5 μmol/min/mg protein in L. dispar [78] or nearly 3 μmol/min/mg protein in Papillio glaucus [24]. Various allelochemicals and herbicides act as inducers of this enzyme. Activity of CarE in the midgut of S. frugiperda exposed to 0.1% atrazine was nearly three times higher than the constitutive level [77].
In contrast, GST, important in the second phase of detoxification and used a biomarker of a general stress [14,63] in the midgut of C. ohridella had higher activity than reported for many other lepidopteran species). Anticarsia gemmatalis or Spodoptera littoralis showed GST activity 10 to 100 times lower [79] while only in one papillionid larvae GST it was kept at a similar level to C. ohridella [26]. Low GST activity profile characterizes rather oligophagic (specialist) species. Polyphages (generalists) must utilize diet containing a wider spectrum of allelochemicals and adapt themselves using more specific esterase isoenzymes [13,26,70]. The main host plant of C. ohridella is the white horse chestnut but the profile of esterase suggests that wider dietary spectrum is possible. This is confirmed by observations from various regions where larvae of C. ohridella were recorded on other tree species, such as the red horse chestnut, sycamore or maple [30,80,81]. High GST activity may simply indicate intensification of conjugation processes with lipid peroxides or products of prooxidative action of metals [64]. It is also possible that C. ohridella produce some GST isoforms that could also play a peroxidative role compensating a weak expression of glutathione peroxidase participating in decomposition of hydrogen peroxide and other oxygen derivatives into H2O [14,23,58,59]. Certain allelochemicals, such as flavonoids, unsaturated carbonyl compounds or phenols may act also as inhibitors of GST [82].
Among various ways of antioxidant defence against oxidative stress, the crucial role of CAT, SOD and GSTPX is beyond any doubt [14,15,26,59,70,73,83]. SOD, together with CAT, is of high importance, alleviating the damages caused by free radicals [83]. Tolerance of reactive oxygen species (ROS) in lepidopterans is species specific and correlate well with activities of antioxidative enzymes [13,70].
Mean activity of SOD (0.27 ± 0.12 A.U./min/mg protein) in the larval midgut was much lower than in many representatives of lepidopterans: 10-20 times lower than in the midgut of Trichoplusia ni [38,69], 15 times lower in Spodoptera eridania or P. polyxenes [57,58]. Also activity of CAT (9.8 ± 3.6 μmol H2O2/min/mg protein) was lower than in other analyzed species of Lepidoptera (Table 1). Such activity profile of both enzymes results from comparably lower amount of allelochemicals in horse chestnut leaves [26]. Larvae of the first generation utilize young leaves containing low amounts of allelochemicals. As time went by the season, due to drastically shortened period for photosynthesis caused by the invaders, the trade-off mechanisms force the attacked tree to switch its metabolic processes into energy saving for quantitative reproductive effects [3,4,59].
Higher mean activity of GSTPX (86 ± 38 nmol cumOOH/min/mg protein) in C. ohridella is in contrast to these stated in other compared Lepidoptera species (Table 1). The mean activity exceeded 350 times the mean activity stated in S. littoralis larvae feeding on potato leaves [16]. Low CAT/GTPX ratio suggests importance of the second enzyme in reduction of organic superoxides to lipid hydroxides. Low SOD activity in relation to CAT and GSTPX suggest higher participation of ROS scavenging reactions using glutathione (GSH). The major scavenger of oxidants is glutathione (GSH), and vitamins A, C and E, participating in elimination of thiols and ascorbates, or the repression of lipid peroxidation [14,84,85]. Relatively low activity of SOD in C. ohridella midgut may result from prooxidative activity of allelochemicals produced by its host plant. Pyrzanowska et al. showed that metabolites produced by horse chestnut leaves are active in antioxidative reactions protecting against oxidation of flavonoids [86]. Known allelochemicals produced in leaves of the horse chestnut tree are: triterpene saponins (aescin), coumarin (aesculin, fraxin, scopolin), flavonoids (quercetin, campherol, astragolin, isoquercetin, rutin, leucocyanidin), oleic and linoleic acids, alantoin and tannins [53,86]. This indicates that the host plant of C. ohridella has at its disposal severe chemical weapons against grazers. It is known that the number and concentration of allelochemicals are season dependent and distinct relations between given allelochemicals and insect responses are difficult to express in natural conditions without a detailed chemical analysis.
A comparison of the above mentioned enzyme activities between C. ohridella and other lepidopteran species indicate distinct species specificity (Table 1). Quantity and quality of consumed food and different feeding habits are the main factors that decide on interspecies variability of enzyme responses. Moreover, caterpillars of C. ohridella are feeding inside the leaf while the compared species are whole leaf chewers. Despite the fact that constraints in detoxifying abilities of the leaf miners depend on similar factors, their enzymatic responses could differ from these of larger external insect grazers.
4.5 Concluding remarks
Overall, our results indicate that activity patterns of detoxifying and antioxidative enzymes in the midgut of C. ohridella larvae were kept in narrow range of activity changes. This suggests that consecutive generations that appear in the course of time of the same year could easily respond to the chemical stressors within their intrinsic tolerance limits. Their genetic plasticity decides on vitality of local populations and analysis of three main factors (generations within the season, three consecutive seasons and age of the host trees at the site) confirmed their significant influence on insect enzymatic responses. The highest effects on enzyme activity patterns were indicated in relation to annual changes of external physical factors. Combined effects of higher average temperature and optimal rainfall resulted in lower enzyme activity levels in the third year of study. Within the season enzyme activities were usually higher in the second generation than in the first one, in order to counteract increased toxicity of the consumed food. The activities remain unchanged or slightly increased in the third generation. General enzyme activity patterns were further modified by the ability of the host tree to counteract intensive defoliation. Responses of larvae living on older host trees, which produce more allelochemicals to cope with miners, were more strongly pronounced in CarE, GST and GSTPX activities. Effects on antioxidative enzymes (CAT, SD) were weaker. Comparisons of antioxidant and detoxifying enzyme activities in the midgut of C. ohridella larvae with the larvae of other species of Lepidoptera indicated some advantages of a discrete life in the mine. In comparison with external folivores consumption of more nutritious parts of the leaf and reduced mobility could explain low activity levels of the antioxidative enzymes of C. ohridella. They more effectively use glutathione dependent system as the major scavenger of oxidants supported by other prooxidants present in the leaf of the horse chestnut.
Disclosure of interest
The authors declare that they have no conflicts of interest concerning this article.
Acknowledgements
This work was partly supported by the Polish State Committee for Scientific Research Grant No. 2 P04F 033 30. The authors are greatly indebted to Gary McLeod for the improvement of the English text and to Prof. Jacques Lehouelleur for linguistic reviewing of the abstract.