1 Introduction
Researches on the possible consequences of electromagnetic waves on microorganisms are more relevant than ever. In recent years, the use of microwave radiation has become popular in the food industry for thawing, drying, and baking, as well as for the inactivation of microorganisms in food [1]. In particular, microbial destruction by microwave radiation has great potential in the pasteurization of food [2].
There have been many studies on the use of microwaves for the reduction of microorganisms in various foods, including turkey beef, corn-soy milk, chicken, frozen foods, and potatoes [3–5]. All of these works have led to the conclusion that microwave radiation extends food preservation by reducing microbial cells in food. Microwave heating is known to inactivate many microorganisms, such as Escherichia coli, Streptococcus faecalis, Clostridium perfringens, Staphylococcus aureus, and Listeria spp. [5–7].
Antibiotics such as β-lactams [8] and chloramphenicol [5] permeate the Gram-negative outer membrane via porins. As such, changes in porin copy number, size or selectivity will alter the rate of diffusion of these antibiotics [9].
So, modifications in sensitivity or resistance of bacteria to antibiotics after irradiation with microwaves will confirm alteration of bacterial membrane by this type of radiation.
The lipid bilayer forms the framework of the cytoplasmic membrane. The most common membrane fatty acids are 14 to 20 carbons long [10]. Acyl chains of this length correctly balance the stability and fluidity of the membrane. Membrane lipids are among the most adaptable molecules in response to physicochemical perturbations. The most common mechanism used to modulate membrane fluidity is the changing of the ratio of saturated–unsaturated fatty acids [11]. The increase of unsaturation can be regarded as a universally conserved adaptation response and this mechanism has been described for several microbes [12]. In fact, UFA synthesis tends to increase membrane fluidity [13].
Indeed, for exposure to gamma radiation (1 kGy), Ayari et al. (in 2009) [14] have demonstrated an increase in the percentage of unsaturated fatty acids in Salmonella typhimurium and Bacillus cereus, which promote membrane fluidity.
Sodium deoxycholate is a chemical inhibitor used to isolate Gram-negative bacteria by inhibiting the growth of Gram-positive bacteria after the activation of autolytic enzymes that cause cell lysis [15,16].
However, it turned out that when the bacterial membrane of S. typhimurium is impaired, sodium deoxycholate can permeabilize the cell, inducing the inhibition of Salmonella growth [17]. Then, the test of viability in the presence of sodium deoxycholate was made to study a possible weakening of the membrane of S. typhimurium by microwave (f = 2.45 GHz) radiation.
In Gram-negative pathogenic bacteria, the outer membrane plays an important role in the infection and pathogenicity to host. The outer membrane proteins (OMPs) play a crucial role during many cellular and physiological processes [18]. OMPs play a key role in the adaptation of the organism to changes in external environments, due to their location at the outermost area of the cell [19]. Several works have shown that when bacteria are transferred to a new environment, the synthesis of their OMPs would change [20].
Utilization of microwave irradiation as a preservation method of foodstuff raises the need for more information on the behaviour of pathogenic microorganisms that may adapt to a sub-lethal radiation dose.
2 Material and methods
2.1 Bacterial culture and microwave treatment
S. typhimurium wild-type strain SL 1344 was kindly provided by Dr. Josef Casadesus (Departamento de Genética Facultad de Biología, Universidad de Sevilla, Spain).
Pure cultures of bacterial strain were maintained on nutrient broth agar (NB-agar) plates at 4 °C. Precultures were prepared by transferring an isolated colony from a plate into a test tube containing 10 mL of sterile NB followed by aerobic incubation in a shaker water bath at 37 °C for 24 h.
Erlenmeyer flasks containing 50 mL of sterile NB were inoculated with the subculture to a final concentration of 103 CFU/mL.
The optical density of the cultures and the dry-weight contents were assayed as described by Ames (in 1968) [21].
For the microwave heating, a 2,450-GHz microwave oven (LG) was used. The glass tube containing 5 mL of cell suspension with a concentration corresponding to 0.5 OD600 (exponential phase) was placed in the centre of the oven and exposed to microwaves at a power of 90 W.
2.2 Measurements of viable cell counts
The dynamics of bacterial growth was estimated by the number of viable cells.
The pure bacterial strain was incubated at 37 °C for 24 h in the Nutrient Broth at neutral pH. Serial dilutions were prepared in 9 mL of 0.85% (w/v) sterile saline solution and final density was adjusted to 0.5 OD600. The experiment was performed in various durations of exposure (10, 20, 30, 40, 50, 60, 70, 80, 90 and 100 s) to determine the effect of microwave radiation on the viability of S. typhimurium. Each glass tube containing 5 mL of cell suspension was placed in the centre of the oven and exposed to microwaves during the appropriate time of exposure. Then, they were diluted with sterile saline solution and stirred for suspension of cells. After serial dilutions, 100 μL of bacteria in the sterile saline were spread over a standard agar plate (nutrient agar).
The plates were incubated at 37 °C for 24 h and colonies were counted. Also, untreated samples were used as a control group of the tested microorganism.
The viability of S. typhimurium was expressed as the log10 of the surviving fraction (number of the colony-forming units [CFU] per millilitre [N]).
2.3 Antimicrobial susceptibility tests
Antimicrobial susceptibility tests were performed using the agar diffusion method. It is a comparison of the inhibition zone diameter induced by antibiotics between exposed and control bacteria.
A glass tube containing 5 mL of cell suspension was placed in the centre of the oven and exposed to a sub-lethal dose of microwave radiation. The samples were taken during the exponential phase (OD600 = 0.5). After the microwave treatment, 1 mL of the bacterial suspension was spread on agar plates. Antibiotic disks were disposed manually and plates were incubated at 37 C for 18 h.
Antibiograms were performed using the following antibiotics (BioMérieux, France): penicillin 10 U (P), oxacillin 1 μg (Ox), amoxicillin 25 μg (AMX), ticarcillin/calvulanic acid 75/10 μg (TIM), cephalotin 30 μg (CF), cefotaxim 30 μg (CTX), ceftriaxon 30 μg (CRO), neomycin 30 μg (N), gentamicin 10 μg (GM), chloramphenicol 30 μg (C30) and polymyxin B 300 UI (PB).
Untreated samples were used as a control group.
2.4 Analysis of fatty acids in total lipids
The bacterial strain was cultured at 37 °C and cells were harvested from 1000-mL cultures in exponential phase (OD600 = 0.5) by centrifugation (4000 g, 10 min, 4 °C), and washed with 1% NaCl.
The bacterial pellets were obtained from a non-irradiated bacterial culture, a bacterial culture irradiated for 20 s and another culture irradiated for 40 s.
Bacterial lysis was performed by a series of freeze/thaw. Then, the lysate was washed with 0.9% NaCl and centrifuged (9000 rpm for 2 min). The supernatant was removed and the pellet consisting of membrane debris was recovered.
The extraction of total lipids from the bacterial lysate was performed using the method of Folch et al. (in 1957) [22] modified by Bligh and Dyer (in 1959) [23].
The pellet was resuspended in 2 mL of sterile H2O. Methanol/chloroform (5 v/2.5 v) were added and centrifugation (3000 rpm, 7 min) was then performed to recover the supernatant “SN1”. To ensure better performance, the pellet obtained after centrifugation was resuspended in the previous methanol/chloroform/water mixtures in the following proportions (6.3 v/3.1 v/2.5 v). The supernatant “SN2” was recovered by centrifugation (3000 rpm, 7 min).
The two supernatants “SN1” and “SN2” were mixed with 7 mL of chloroform and 7 mL of H2O. The chloroform phase containing the lipids was recovered by centrifugation (4000 rpm, 10 min), dried at 40 °C in Rotavapor and then recovered in 1 mL of chloroform and dried under a stream of nitrogen gas.
Fatty acids were converted to their methyl esters (FAMEs) according to the method described by Cecchi et al. (in 1985). An aliquot of the solution was evaporated; then 2 mL of hexane, a known quantity of heneicosanoic acid methyl ester [C21:0] as an internal standard and 0.5 mL of sodium methylate (1%) were added. After stirring over 1 min and standing for 2 min, the mixture was neutralized by 0.2 mL of H2SO4 (1 N); then, the methyl esters were washed with 1.5 mL of distilled water. The upper phase solvent containing the FAMEs was removed under vacuum.
Fatty acids relative composition was subsequently determined as % of the total fatty acids using a HP 6890 chromatograph equipped with a flame ionisation detector (FID) and an electronic pressure control (EPC) injector.
A polyethylene glycol fused silica capillary column (Innowax, 30 m × 0.25 mm × 0.25 μm film thickness) purchased from Agilent (Wilmington, Delaware, USA) was used.
The column was operated at 150 °C for 1 min, and the temperature was raised at a rate of 15 °C min−1 to 200 °C and finally held at 242 °C for 2 °C min−1. N2 was used as the carrier gas at a flow rate of 1.6 mL min−1 and the split ratio used was 60:1.
2.5 Weakening of the bacterial membrane by sodium deoxycholate
After exposure to microwaves and serial dilutions, 100 μL of bacteria in the sterile saline were spread over deoxycholate (0.1%) lactose agar plates.
The plates were incubated at 37 °C for 24 h and colonies were counted after an incubation period. Untreated samples were used as a control group of tested microorganism.
2.6 Measurements of protein amounts
The amount of protein released from the microwave-treated and untreated cells was measured at 750 nm by the method of Lowry. Bovine serum albumin was used as the standard protein.
2.7 Statistical analysis
The experimental data were analyzed using the analysis of variance (ANOVA). Differences at P < 0.05 were considered statistically significant by the Newman–Keuls test. All values were expressed as means ± standard deviation of at least six repetitions.
3 Results
3.1 Inactivation of bacterial cells by microwave radiation
As shown in Fig. 1, the number of viable colonies was severely affected by the increase of the energy delivered by microwaves after exposure.
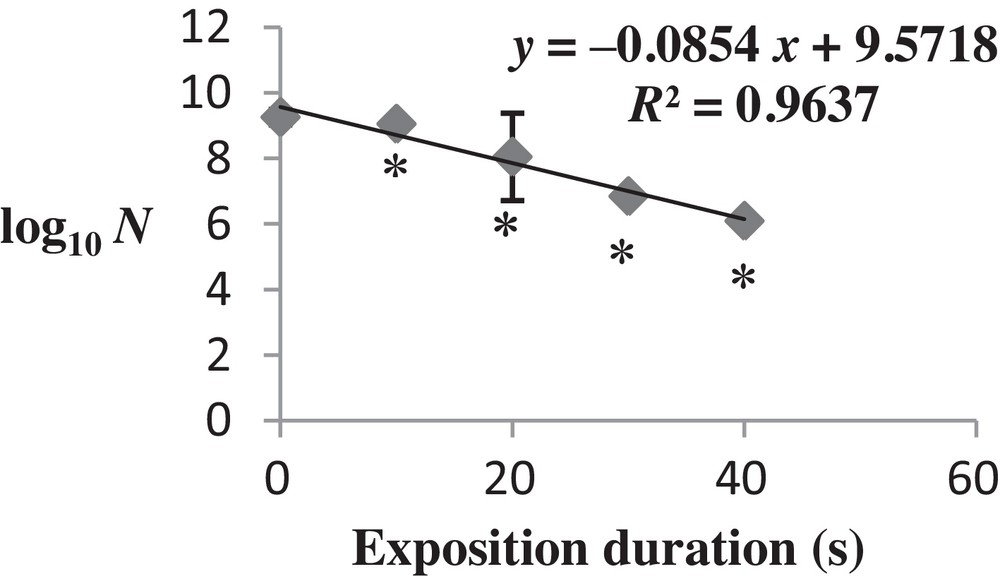
Changes in the viability of Salmonella typhimurium exposed to microwaves (2.45 GHz). The survival data are plotted as the log10 of the ratio of the number of surviving colony-forming units (CFU) per milliliter. The logarithms are plotted against time. The values are the mean SD of six different CFU measurements (n = 6) (ANOVA test). *P < 0.05 vs. control samples.
These results resumed a bacteriostatic effect of microwave radiation for a current up to 3600 J of irradiation (40-s exposure with P = 90 W).
Beyond 3600 J, the exposure of Salmonella to microwaves induced a total destruction of bacterial cells, which confirmed that microwaves have a bactericidal effect on this bacteria (P < 0.05).
Following this result, we chose to make the rest of analyzes with a duration of exposure corresponding to 40 s. This dose was the closest to the lethal dose, so such analyzes will elucidate some mechanisms of biological adaptive transformations in S. typhimurium after the application of the microwave stress.
3.2 Antimicrobial susceptibility tests
Comparative analysis of antimicrobial susceptibility has shown a significant increase in the inhibition zone diameter of tested antibiotics in exposed bacterial cells. For penicillin, a significant decrease in the inhibition zone was observed (Fig. 2). Results are detailed in Table 1.
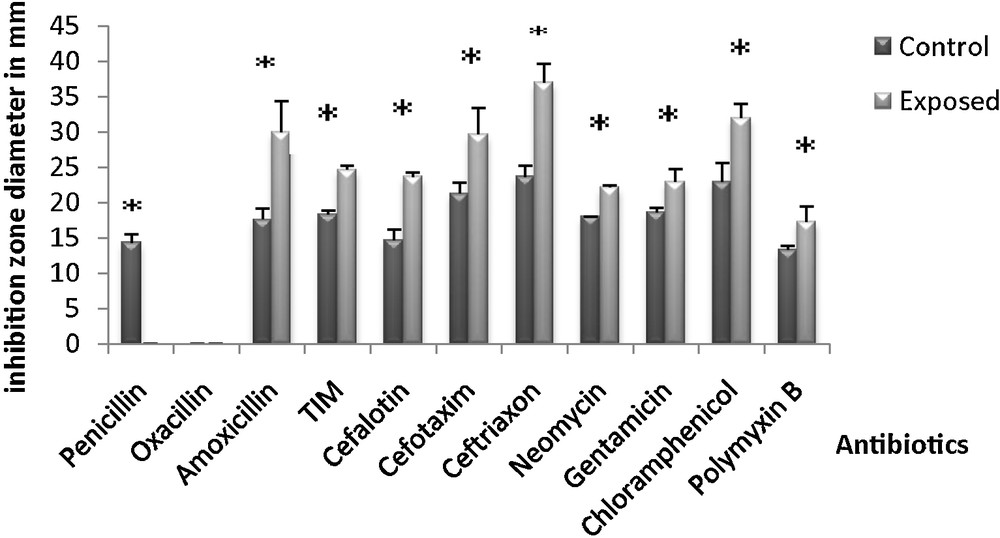
Changes in the inhibition zone diameter induced by antibiotics after irradiation of Salmonella typhimurium with microwaves (fmicrowaves = 2.45 GHz). The values are the mean SD of six different measurements (n = 6) (ANOVA test). *P < 0.05 vs. control samples.
Effect of microwaves on the resistance and sensitivity to antibiotics in control bacteria and in bacteria exposed for 40 s.
Antibiotics | Control bacteria | Exposed bacteria | P | Difference |
Penicillin G | R | R+++ | 0.0002 | S |
Oxacillin | R | R | – | NS |
Amoxicillin | I | S | 0.01 | S |
Ticarcillin/calvulanic acid | R | S | 0.0004 | S |
Cefalotin | I | S | 0.0089 | S |
Cefotaxim | R | S | 0.024 | S |
Ceftriaxon | I | S | 0.008 | S |
Neomycin | S | S+++ | 0.313 | NS |
Gentamicin | S | S+++ | 0.014 | S |
Chloramphenicol | R | S | 0.009 | S |
Polymyxin B | R | S | 0.032 | S |
It was especially the sensitivity to antibiotics belonging to the family of β-lactams (ticarcillin/calvulanic (TIM), cefotaxim (CTX 30)), chloramphenicol (C30) and polymyxin B (PB300), which was increased following microwave radiation; all of them interfere, directly or indirectly, with the synthesis metabolism of the bacterial membrane.
3.3 Analysis of total lipids by gas chromatography
Membrane lipids are among the best molecules that fit the physical and chemical disturbances suffered by cells in order to maintain membrane fluidity and integrity. This is why we were interested in studying the effects of exposure to microwaves with a frequency of 2.45 GHz on the fatty acid membrane composition in S. typhimurium.
The membrane FA composition of non-exposed S. typhimurium was determined (Table 2). Many FA were found and six main peaks were identified by comparing their retention times with those of known standards.
Rates of fatty acids (FA, expressed in %) in total membrane lipid in control bacteria and in microwave-exposed bacteria (fmicrowaves = 2.45 GHz; exposure times: 20 and 40 s).
FA | Bacteria | ||||
Control | Exposed (20 s) | P * | Exposed (40 s) | P ** | |
C14:0 | 2.596 ± 0.04 | 2.44 ± 0.05 | 0.013 | 2.113 ± 0.075 | 0.0008 |
C16:0 | 40.05 ± 0.29 | 39.4 ± 0.44 | 0.099 | 36.753 ± 0.419 | 0.0005 |
C16:1 | 10.75 ± 1 | 12.11 ± 0.33 | 0.089 | 14.030 ± 0.3 | 0.005 |
C17:0 | 2.72 ± 0.17 | 2.48 ± 0.02 | 0.072 | 2.390 ± 0.07 | 0.036 |
C18:0 | 33.924 ± 0.636 | 32.041 ± 0.926 | 0.044 | 31.16 ± 0.91 | 0.012 |
C18:1 | 8.8 ± 0.02 | 12.303 ± 0.475 | 0.0004 | 13.18 ± 0.71 | 0.0004 |
In fact, four SFAs were found: tetradecanoic (myristic) acid (C14:0), hexadecanoic (palmitic) acid (C16:0), heptadecanoic (enanthic) acid (C17:0), octadecanoic (stearic) acid (C18:0); and two mono-UFAs: hexadecenoic (palmitoleic) acid (C16:1Δ7), and octadecenoic (oleic or vaccenic) acid (C18:1Δ9).
In control bacteria, palmitic (C16:0) and stearic (C18:0) acids were the main constituents, with the following respective proportions: 40.05% and 33.92% (Table 2).
Minimum FA levels were observed for C14:0 (2.59%), C16:1 (10.75%), C17:0 (2.72%) and C18:1 (8.8%). To determine whether exposure of bacteria to microwaves affected membrane lipid components, FA composition was quantified.
Membrane FA composition (molar percent) of the control and exposed bacteria during 20 and 40 s is shown in Table 2.
Our results indicated that FA composition was significantly affected after microwave treatment. The modifications observed were more important in bacteria exposed for 40 s than in those exposed for 20 s.
A decrease in the proportions of SFA was determined in exposed bacteria compared to control bacteria. This decrease was more significant in bacteria exposed during 40 s: C14:0 (from 2.596% to 2.113%); C16:0 (from 40.05% to 36.753%); C17:0 (from 2.72% to 2.39%); C18:0 (from 33.924% to 31.16%). An increase in the rate of UFAs was also observed in the exposed ones.
This rise was more important after 40 s of exposition. So, the rate of C16:1 was changed from 10.75% to 14.03% and that of C18:1 from 8.8% to 13.18%.
Indeed, the (unsaturated fatty acids/saturated fatty acids) ratio provides information about the degree of unsaturation of fatty acids after microwave irradiation. Thus, this ratio showed a preferential accumulation of unsaturated fatty acids.
So, the UFA/SFA ratio was increased from 0.195 in control bacteria to 0.37 in exposed bacteria during 40 s, as shown in Fig. 3.

Comparative representation of (UFA/SFA) ratio in control bacteria, and microwave-exposed bacteria (fmicrowaves = 2.45 GHz; exposure times: 20 and 40 s). The values are the mean SD of six different measurements (n = 6) (ANOVA test). *P < 0.05 vs. control samples.
The application of the ANOVA statistical test showed that this increase was significant for exposed bacteria during 40 s (P < 0.05).
3.4 Weakening of the bacterial membrane by microwaves
3.4.1 Viability of control and irradiated bacteria in the presence of sodium deoxycholate
It turned out that when the bacterial membrane of S. typhimurium is impaired, sodium deoxycholate may penetrate cells and induce the inhibition of the cellular growth in Salmonella.
Thus, the test of the viability in the presence of sodium deoxycholate was performed to confirm the weakening of S. typhimurium membrane by microwave (f = 2.45 GHz) irradiation.
Our results have shown that control S. typhimurium was viable in the presence of sodium deoxycholate. However, survival of 40-s microwave-irradiated bacteria was completely inhibited (P < 0.05).
3.4.2 Effect of microwaves on the amount of extracellular proteins
In order to study the effect of microwaves on the phenomenon of release of membrane proteins to the external environment (culture medium), the amount of extracellular proteins was quantified by the method of Lowry, before and after bacteria had been exposed.
Treatment of bacteria with microwaves has led to a remarkable increase in the amount of extracellular proteins (P < 0.05) (Fig. 4).

Modification in the concentration of extracellular proteins in bacteria after irradiation with microwaves for 40 s. The values are the means SD of six different measurements (n = 6) (ANOVA test). *P < 0.05 vs. control samples.
4 Discussion
4.1 Effect of microwaves on the viability of Salmonella typhimurium
In order to investigate the potential effects of microwaves (f = 2.45 GHz) on the membrane of S. typhimurium, we began by studying their effect on bacterial growth.
Our analysis has shown that microwaves exerted a bactericidal action by inhibiting cellular growth and that it was irreversible. One of the explanations for this effect was linked to the selective absorption of microwave energy by the microorganism resulting in its inactivation [24].
According to Khalil and Villota (in 1989) [25], this effect has been induced by the important microwave heating of certain cellular components such as proteins or lipids.
Similarly, Shin and Pyun (in 1997) [26] have shown that microwaves induced an irreversible damage to the membrane of Lactobacillus plantarum, associated with an increased permeability.
According to Delaporte, when a dense microbial population was subjected to ultraviolet irradiation, the surviving seeds that divide had a revivifying action on microorganisms in their immediate environs. This is called the Delaporte effect [27].
This effect has been proven by Kanazir's work (in 2003) [28], in which the synthesis of desoxyribonucleic acid was stopped immediately after UV irradiation of a culture of E. coli in the logarithmic phase. This effect was not permanent and synthesis was resumed after periods which were approximately proportional to the irradiation duration.
4.2 Effect of microwaves on the resistance and the sensitivity of Salmonella typhimurium to antibiotics
The nature of antibiotics, their mode of penetration inside the cell, or their mechanism of action could be involved in this differential behaviour. The main theories that try to explain the biological effects of microwaves are based on the possible effects on the permeability of ionic channels in the membrane [29]. To explain the increase of Salmonella sensitivity to gentamicin, it could be hypothesized that the penetration of this antibiotic into bacteria was enhanced. Further research is needed to elucidate the related mechanism. In fact, our results are consistent with those of Pickering et al. (in 2003) [30], who treated implant infections using a pulsed magnetic field. They have shown that applying a pulsed magnetic field (72 Hz) improved the effectiveness of gentamicin against Staphylococcus epidermidis biofilm.
Similarly, 12 hours of exposure to magnetic field (200 mT) has also increased the sensitivity of Salmonella Hadar 287 to gentamicin [31].
The sensitivity to β-lactam [8] antibiotics and chloramphenicol [5] depends on the number of functional porins. An alteration, a structural modification of an essential porin or a quantitative reduction of porins are the main causes of resistance acquisition to β-lactam antibiotics [32].
So, the increase of the resistance towards penicillin may be due to a decrease in its distribution within the cell. This decrease may be caused either by a quantitative reduction of porins or by a structural modification of an essential porin such a narrowing of the porin channel.
In Gram-negative bacteria, many cationic antimicrobial peptides (APs) associate electrostatically with the bacterial outer membrane, namely the lipopolysaccharides (LPSs), via ionic bonds with unsubstituted anionic phosphate residues present on LPS lipid A and core oligosaccharide. Subsequently to binding, the APs permeabilize the outer membrane, translocate to the cytoplasmic membrane, which is the main cytotoxic target of most APs, and then form pores, resulting in leakage of cell constituents and cell death [33].
In Salmonella enterica serovar Typhimurium, the evasion of AP killing is directed in part by the PmrA–PmrB two-component regulatory system [34].
Activation of PmrA–PmrB confers resistance to polymyxin B. PmrA–PmrB accomplishes resistance to these AP by up-regulating genes involved in covalent modifications of the LPS [34]. The LPS modifications reduce the negative charge of LPS and consequently decrease the attraction and binding of AP to the outer membrane. PmrA-induced modifications to LPS include the addition of 4-amino-4-deoxy-l-arabinose (Ara4N) to the 4-phosphate of lipid A (and sometimes the 1-phosphate) and the addition of phosphoethanolamine (pEtN) to the 1-phosphate of lipid A (and sometimes the 4-phosphate), to 2-keto-3-deoxyoctulosonic acid, and to the first heptose residue of the core [35].
One of the explanations that we may suggest for the appearance of sensitivity in S. typhimurium vis-à-vis Polymyxin B is that microwaves affected the stability of the outer membrane and particularly the charge of LPSs, causing an increased attraction of this antibiotic and thus a possible connection to the outer membrane. Our result is consistent with that of Tamayo et al. (in 2002) [33], who found that insertion of the transposon tolb in S. typhimurium could cause an hypersensitivity to polymyxin B as a result of a loss of outer membrane stability. Thus, the destabilization of LPS layer allows the penetration of polymyxin B in the periplasm to reach the cytoplasmic membrane.
4.3 Effect of microwave treatment on FA composition
Analysis of the total lipid composition of the bacterial membrane in S. typhimurium after exposure to microwaves has shown a quantitative change in the proportions of saturated and unsaturated fatty acids. This change resulted in an increase of the unsaturated fatty acids rate and a decrease of the saturated fatty acids rate.
Many studies have suggested a relationship between membrane fluidity and bacterial stress tolerance, highlighting the importance of fatty acids in maintaining the membrane fluidity [36]. The increase in unsaturation can be seen as an adaptive response universally conserved, and this mechanism has been described for the most virulent microorganisms [12].
Indeed, for exposure to gamma radiation (1 kGy), Ayari et al. (in 2009) [14] have demonstrated an increase in the percentage of unsaturated fatty acids in S. typhimurium and B. cereus, which promote membrane fluidity.
Assuming that the electromagnetic field is responsible for activating the production of free radicals, according to assumptions made by Strasàk et al. (in 2002) [37], antioxidant defences should be held to protect against various biological disorders. In this context, Guersoni et al. (in 2001) [38] have shown an increase in the activity of fatty acid desaturase, which can be considered as a mechanism of reaction that consumes oxygen and reduces free radicals in damaged cells.
Thus, exposure to microwaves may influence via stress proteins the lipid biosynthesis and so regulate the transcription of genes that affect the metabolism of fatty acids.
By measuring the fluidity of E. coli after increasing the temperature, Mejia et al. (in 1999) [39] showed that the fluidity decreased; they attributed these results to the changes in the membrane's lipid composition and especially the proportion of unsaturated fatty acids in the cell.
4.4 Effect of microwave treatment on the viability of Salmonella typhimurium in the presence of sodium deoxycholate
Results have shown that bacterial cells exposed to microwaves were sensitive to sodium deoxycholate and that bacterial growth was completely inhibited, while non-irradiated cells were resistant and viable in the presence of this inhibitor.
Indeed, several authors have shown that the appearance of this sensitivity vis-à-vis deoxycholate in S. typhimurium is mainly due to an alteration in the bacterial membrane.
According to Vaara (in 1992) [40], Boziaris et al. (in 1998) [41], Mackey (in 2000) [42] and Aljarallah et al. (in 2006) [17], S. typhimurium is resistant to deoxycholate, but bacterial cells whose outer membrane is damaged by a selective factor will be fragile and will not grow in its presence.
Varra et al. (in 1980) [43] studied the effect of Polymyxin on the permeability of the outer membrane vis-à-vis the deoxycholate. They found that under normal conditions, this agent does not penetrate the outer membrane of intact S. typhimurium. But when the membrane is altered by Polymyxin, it can enter. The same result was obtained in Salmonella with LPS treated with EDTA.
In addition, Ames et al. (in 1974) [44] found that mutant strains that produce LPS without heptose, or with one or two heptose residues, were also sensitive to deoxycholate, since this destabilization affects the inner membrane by increasing its permeability to toxic compounds, including deoxycholate.
So, this result confirms that the sensitivity observed in irradiated Salmonella vis-à-vis deoxycholate was due to a weakening of bacterial membrane caused by the applied microwave stress.
4.5 Effect of microwaves on the amount of extracellular proteins
To highlight the effect of microwaves on membrane proteins, we used the Lowry assay to quantify the extracellular proteins in irradiated and non-radiated bacteria. The results have shown a significant increase in the amount of membrane proteins after irradiation with microwaves. This increase may be due to an over-expression of the extracellular proteins to ensure the adaptation of Salmonella to the stress induced by microwaves. Or, this augmentation may result from the release of membrane proteins in the extracellular medium due to the weakening of the membrane structure.
Several works have shown that when bacteria are transferred to a new environment, the synthesis of their OMPs changes [20]. Ben Abdallah et al. (in 2009) [18] showed alterations in outer-membrane proteins of foodborne pathogens V. alginolyticus and V. parahaemolyticus cells after their treatments with δ-irradiation. These alterations were manifested by the disappearance or the attenuation of the expression of certain proteins.
Environmental changes influence the expression of OMPs in E. coli [45], Stenotrophomonas maltophilia [46], Leptospira interrogans serovar Lai [47], Borrelia burgdorferi [48], and Serratia marcescens [49].
Acidic pH also induced the expression of new proteins on the surface of Yersinia pestis, and OMP expression of B. burgdorferi was also altered at different pH values [18]. The altered osmolarity of the culture medium caused changes in the OMP patterns of V. alginolyticus [19].
5 Conclusion
In this study, we tried to analyze the mechanism of defence and stress adaptation in S. typhimurium induced by exposure to microwaves.
The study of the effects of microwaves (f = 2.45 GHz) on some biological characteristics of S. typhimurium has shown that the exposure of bacteria to microwaves resulted in a significant inhibition of cellular growth. Application of microwaves and monitoring the viability of bacterial cells showed a bactericidal effect taking place during irradiation. Microwave irradiation has notably increased the effectiveness of the most tested antibiotics by the amelioration or the appearance of sensitivity in treated bacteria. Furthermore, GC analysis of membrane fatty acid composition showed that microwaves induced significant changes in lipid composition resulting in a considerable increase in the degree of unsaturation.
The weakening of the bacterial membrane by microwaves is also confirmed by the acquisition of a sensitivity to the sodium deoxycholate in irradiated bacteria. In addition, the results showed that application of microwaves has led to a significant increase in the amount of extracellular proteins in exposed bacteria compared to control bacteria.
Disclosure of interest
The authors declare that they have no conflicts of interest concerning this article.
Acknowledgments
This work was supported by the Tunisian Ministry of Higher Education, Scientific Research and Technology.