1 Introduction
Polycyclic aromatic hydrocarbons (PAHs) are one of persistent organic pollutants (POPs) with carcinogenic and mutagenic properties [1,2]. PAHs are mainly produced from incomplete combustion of organic materials and fossil fuel, and they exist in the different environmental matrices, including soil, water, and sediment [3–6]. They are persistent in various environmental media and enter the plants through their leaf and/or root systems. Subsequently, they are transferred to the food chains and threaten human health [5,7]. Therefore, an improved understanding of PAHs toxic mechanism is essential for the assessment of the risk of PAHs exposure.
Recently, the toxic effects of PAHs on plants have been extensively documented [8–12]. It has been reported that PAHs inhibited the growth of plants, caused a decrease in the photosynthesis of antioxidant enzymes, and even led to lipid peroxidation, DNA damage in plants [13–15]. The degree of toxicity varies with the kind of PAHs and the species of plants [12,16]. Liu et al. [14] suggested that excessive production of ROS in plants was a biochemical response to PAHs. In general, oxidation of PAHs results in the generation of ROS, which in turn causes oxidative stress and subsequent damage to plant cells. However, plants have enzymatic systems, including free radical scavengers, like superoxide dismutase (SOD), peroxidase (POD), and catalase (CAT) [17]. When the elevated levels of ROS exceed the levels that the antioxidant systems can handle, the damage may occur. Thus, it is important to understand the responses of plant system to environmental stress by determining the occurrence of oxidative damages and the changes in antioxidant enzymes’ activities in plant.
Although many studies have been published on PHE's phytotoxicity against different plants, less attention has been paid to seed germination and growth of wheat seedlings. Wheat is one of the main grains in China. Its quality directly affects people's life and food security [18]. Thus, to get a better insight into the mechanism of PAHs toxicology on wheat is essential to an effective environmental management. The objective of this study was to elucidate the effects of PHE on seed germination and growth of wheat seedlings. Variations of the activities of SOD, CAT, glutathione peroxidase (GPX), the content of chlorophyll, and malondiadehyde (MDA) in wheat seedlings were detected, and the probable mechanisms were discussed.
2 Materials and methods
2.1 Plant materials and growth conditions
Authentic wheat seeds were obtained from the Institute of Agriculture Science in Shanxi Province, Taiyuan, China. The wheat (Yunmai 494) seeds were surface sterilized with 0.1% HgCl2 for 10 min, and soaked in water for 12 h. Then, the seeds were randomly divided into four groups, with each group consisting of at least 100 seeds and transplanted in 30-cm diameter Petri dishes with two filter papers. Seeds were exposed to PHE in three different concentrations. The negative control group was exposed to distilled water for the same time period. The treatment was replicated three times. All of the test groups were maintained in an incubator at 25 ± 1 °C under a dark/light cycle (14/10 h). Plants were fertilized daily with water and Hoagland′s nutrient solution. The germination rate would be counted after seven days. Seedlings were harvested after 15 days for biochemical and physiological studies, and the leaf samples were immediately frozen in liquid nitrogen and stored at –80 °C.
2.2 PHE preparation and treatment application
PHE (Sigma, 98% purity) was initially dissolved in acetone and then diluted with MilliQ water to get final concentrations of 0.05, 0.1, 0.2 mg/mL (acetone:water, v:v = 1:1000). Then, three groups of seeds were sprayed with each concentration of freshly prepared PHE. Control seeds were sprayed with MilliQ water containing equal concentrations of acetone. The concentration of acetone used in the current experiment was lower than 0.5%, which had been reported as having no negative effects on tomato seedlings [15].
2.3 Germination energy, germination rate and length of root and stem
The number of germinated seeds on the seventh day after initiation was defined as the germination rate. After fifteen days of growth, the roots and stem lengths of 100 seedlings in each group were randomly measured. Three replicates were conducted from each experimental unit.
2.4 Chlorophyll content measurements
A fresh leaf sample (0.2 g) was pulverized with distilled water and the homogenate was extracted using 80% acetone. Chlorophyll a (chl a) and chlorophyll b (chl b) were determined by spectrophotometry according to the procedure of Sang et al. [19].
2.5 Determination of enzyme activity
For the extraction of enzymes, 0.5 g of the leaf tissues was homogenized in 5 mL of an ice-cooled phosphate buffered solution (0.05 M, pH 7.8) containing 0.2 M EDTA and 2% polyvinylpyrrolidone (w/v). The homogenate was centrifuged at 12,000 rpm and 4 °C for 20 min. The supernatant was used immediately to determine the enzyme activity and the contents of MDA and H2O2.
SOD activity was measured by nitroblue tetrazolium (NBT) spectrophotometry [20]. One unit of SOD activity was defined as the amount of enzyme required to cause 50% inhibition of reduction of NBT as monitored at 560 nm and the enzymes activity was expressed as U·(g FW)−1.
CAT activity was tested through the absorbance decrease at 240 nm using the ultraviolet absorbance method [21]. Results were expressed as U (min g FW)−1.
GPX activity was determined according to the method of Lawrence and Burk [22]. The reaction mixture contained the enzyme extract, 0.2 mM NADPH, 1 mM sodium azide (pH 7.0), 1 mM GSH, 1 U glutathione reductase and 2 mM H2O2. The reaction was initiated by adding H2O2 and the absorbance was measured at 340 nm. One unit of GPX activity was defined as the amount of enzyme catalyzing the oxidation of 1 μmol of NADPH per minute [23].
2.6 Measurement of H2O2
The formation of H2O2 was measured colorimetrically as described by Ishida et al. [24]. The homogenate supernatant was incubated in 2 mL (24 h) or 5 mL (48 h) of the reaction mixture containing 50 mM Na-acetate buffer (pH 6.5), 1 mM 4-aminoantipyrine, 1 mM 2,4-dichlorophenol, 50 mM MnCl2, and 0.2 mM NADH. The increase in absorbance was measured at 510 nm.
2.7 Determination of the MDA content
The MDA content was determined according to the method of Draper and Hadley [25]. Then, 0.2 g stems were homogenized in trichloroacetic acid (TCA) and centrifuged at 3000 g for 10 min. An amount of 200 μL of homogenate supernatant were mixed with 0.8 mL of 0.5% (w/v) thiobarbituric acid (TBA) and 20% TCA, then put in a boiling water-bath for 30 min. The absorbance was measured at 532 nm. The value for non-specific absorbance at 600 nm was subtracted.
2.8 Statistical analysis
Each experiment was repeated at least three times. The data were expressed as means ± standard deviation (SD). The statistical differences (0.05) among the negative control and a series of treated groups were analyzed using one-way analysis of variance (ANOVA).
3 Results
3.1 Effects of PHE on seed germination and growth of wheat seedlings
3.1.1 Germination energy and germination rate of wheat
The germination rate may reflect the reaction rate of plant seeds to their living conditions. From Fig. 1, it can be seen that germination energy and germination rate decreased with increasing PHE concentration. The germination rate was 62.5% with 0.05 mg/mL PHE, and it showed a poor germination rate with high concentrations (0.2 mg/mL). The germination energy reduced significantly by 68%. Correlation analysis indicated that germination energy and rate are strongly correlated with PHE concentration (r = 0.95 and 0.93).
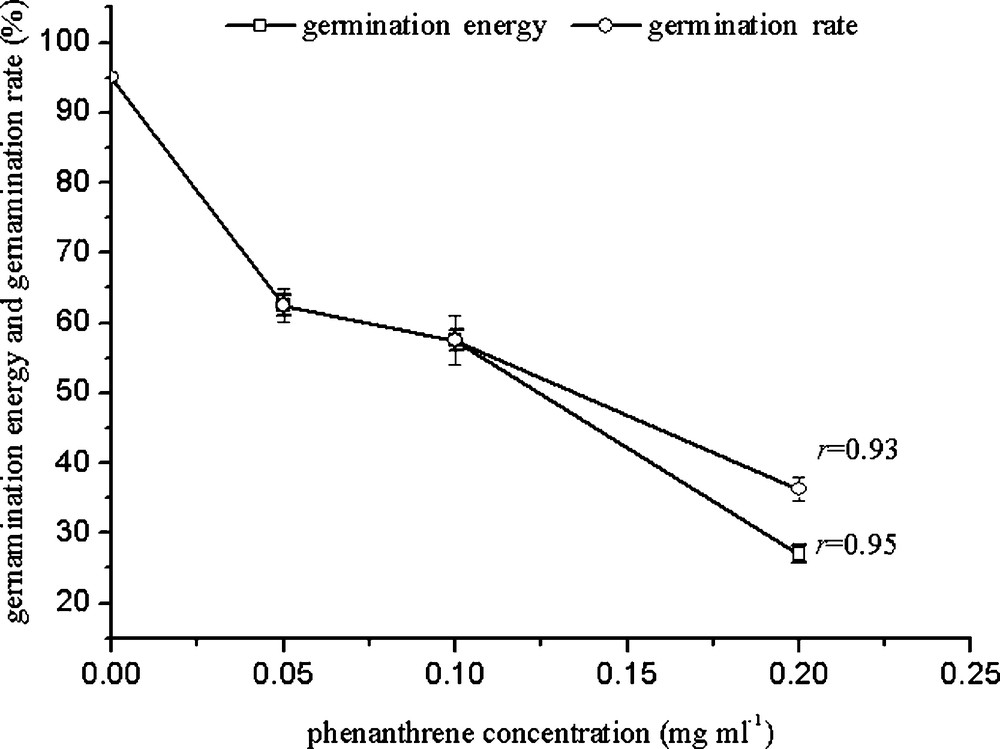
Effects of PHE on wheat germination at different concentrations.
3.1.2 Length of root and stem
Fig. 2 shows root and stem lengths. With increasing the PHE content from 0.05 to 0.2 mg/mL, the root length decreased by 61.42%, 78.24%, and 89.10%, and the stem length decreased by 54.12%, 61.73%, and 68.26%, respectively. According to statistical analysis, PHE had a significant adverse effect on root length, stem length at all concentrations (P < 0.05 or 0.01).

Effects of PHE on the root and stem length of wheat seedlings. The root length and stem length of 100 seedlings from each treatment were randomly measured. Data represent the mean ± SD. *P < 0.05, **P < 0.01 vs control.
3.2 Effects of PHE on physiological activities in leaf tissues of wheat seedlings
3.2.1 Content of chlorophyll
The effects of PHE on chlorophyll levels in the leaf tissue were shown in Table 1. After 15 days of exposure, the chlorophyll contents decreased with increasing PHE concentration, but responses of chlorophyll a and b to various levels of PHE were different. The chlorophyll a content began to decrease at low concentrations and the contents were 92.5% and 81.31% of the control values after being treated with 0.05 mg/mL and 0.10 mg/mL PHE. When the PHE concentration rose to 0.2 mg/mL, a distinct decrease of chlorophyll a was observed, and the content was reduced to 52.33% of the control.
The content of chlorophyll (mg·gFW–1).
Phenanthrene concentration (mg/mL) | Chlorophyll a | Chlorophyll b |
0 | 1.07 ± 0.01 | 0.32 ± 0.00 |
0.05 | 1.00 ± 0.02 | 0.32 ± 0.01 |
0.10 | 0.87 ± 0.01** | 0.29 ± 0.01* |
0.20 | 0.56 ± 0.01** | 0.16 ± 0.02* |
A similar trend was observed for chlorophyll b after PHE exposure. However, at the same concentration levels, the changes in chlorophyll a were more dramatic than those in chlorophyll b. For example, when the PHE concentration was 0.05 mg/mL, the chlorophyll b content was the same as that in the control. When the concentration of PHE reached 0.10 mg/mL, the chlorophyll b content was 90.6% of the control values.
3.2.2 Activities of CAT, SOD, and GPX
The activity changes of CAT, SOD and GPX in the leaf tissues after the treatment of PHE were shown in Fig. 3. The SOD activities in the samples declined with increasing PHE concentration with relative activities of 171.03, 99.01, 91.10 and 82.30 U (g FW)−1, respectively (Fig. 3a) There were significant differences between the control and the sample treated with 0.2 mg/mL (P < 0.05).

Effects of PHE on the activities of SOD (a), CAT (b) and GPX (c) in the leaf tissues of wheat seedling. Data represent the mean ± SD. *P < 0.05, **P < 0.01 vs control.
The responses of CAT to PHE were similar to the trends of SOD. At a low treatment concentration, the activity of CAT decreased slightly. At 0.1 mg/mL PHE, the CAT activity decreased significantly, and the difference was statistically significant compared to the control (P < 0.05). The decrease was more drastic with a 0.2 mg/mL PHE treatment (Fig. 3b).
The activities of GPX in the sample treated with PHE were promoted at a low concentration, and then decline. The GPX activity increased up to 125.76% of the control with a 0.05 mg/mL exposure group. With increasing the exposure PHE concentration, GPX activities decreased, and the values were 88.75% and 71.30% of the control. However, there were no significant differences between the control and PHE treatments (P > 0.05) (Fig. 3 c).
3.2.3 MDA content and H2O2 accumulation in leaf tissues of wheat
MDA was measured as an important parameter in evaluating the oxidative stress induced by PHE. In leaf tissues of wheat seedlings treated with 0.05, 0.1, 0.2 mg/mL, the lipid peroxidation level increased and the effect reached statistical significance with the 0.05 mg/mL treatment (P < 0.05) (Fig. 4).

Effects of PHE on the MDA content in the leaf tissues of wheat seedling. Data represent the mean ± SD. *P < 0.05, **P < 0.01 vs control.
As compared to the control, H2O2 increased up to 123%, 160% and 243% of the control, respectively, when treated with 0.05, 0.1 and 0.2 mg/mL PHE. A significant increase was found for the treatment with 0.05 mg/mL PHE compared to the control (P < 0.05) (Fig. 5).
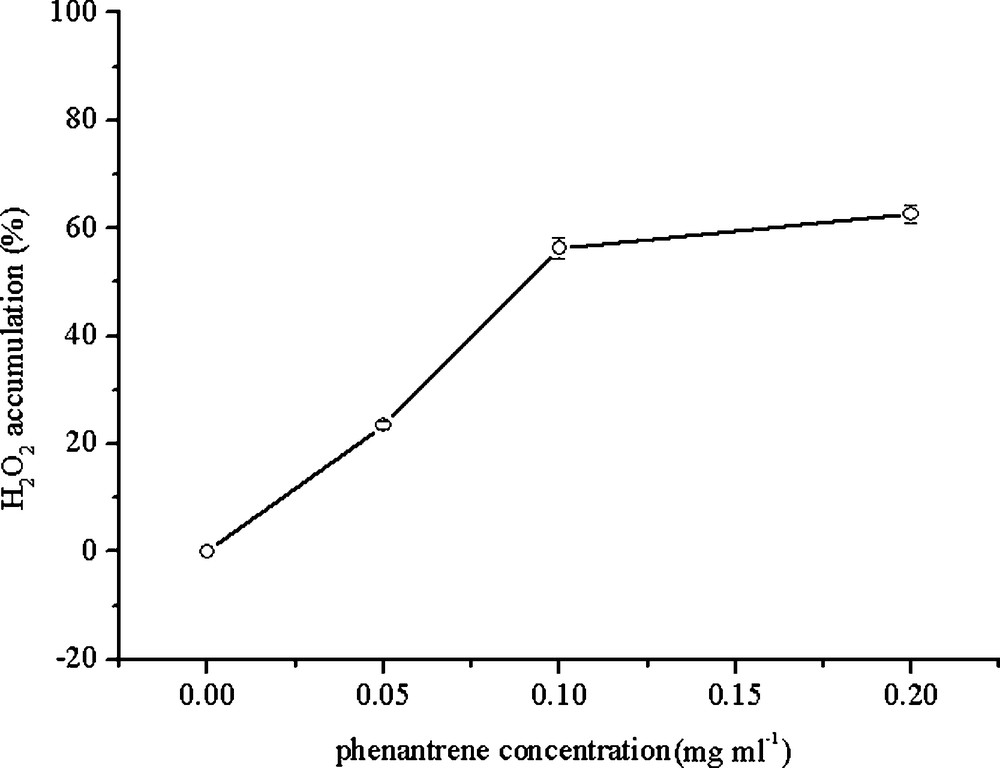
H2O2 accumulation in the leaf tissues of wheat seedling in different PHE treatments. Data represent the mean ± SD.
4 Discussion
PAHs are a large class of compounds with toxicity toward animal and plants. It exerts adverse effects on morphology, growth, photosynthetic process of the plants, and causes inhibition of enzyme activities [11,12,15,26]. Seed germination has often been used as a physiological index to examine the effects of PAHs on plants. A number of reports have showed the inhibitory and toxicity of different PAHs on the seed germination and seedling growth of some plants, and they are regarded as general responses associated with PAHs toxicity [27]. The present work is to unravel the responses of wheat to PHE with a particular focus on seed germination, morphological and physiological indicators of oxidative stress. In this study, wheat materials were exposed to a PHE solution, which effectively avoided the effects of temperature, pH and some other factors linked to soil treatment. Therefore, the results were exact and creditable, and they could reflect the toxic effect of PHE on the plants.
PHE treatment affected growth and chlorophyll content in wheat seedlings; these effects varied as functions of PHE concentration. The decreased root, stem length and chlorophyll content after PHE treatment in different concentrations, which might be a result of the hormetic effect that has been reported in other plants [11,12,14,15,28]. Normally, plants have their stress defense, but environmental pollutants decrease the ability of plants. Thus, PHE inhibited the growth and chlorophyll content of wheat seedlings in our study, which might result from the damage suffered by the defense system.
Several enzymes, like SOD, CAT and GPX, play important roles during seed germination. The activities of antioxidant enzymes (SOD, CAT and GPX) were tested under the same treatment conditions. SOD is the first defense against ROS as it can act on superoxide radicals, which were produced under stress conditions. It catalyzes the dismutation reaction of O2−• into H2O2 and O2 [29]. CAT is the primary H2O2 scavenging enzyme in plant cells [23]. GPX catalyzes the reduction of H2O2 and lipid peroxides using GSH as an electron donor [30]. PHE induced a decrease in SOD, CAT activities and GPX activities and the ability of H2O2 scavenging, which resulted in lipid peroxidation.
MDA is the production of lipid peroxidation, generally the amount of MDA reflects the lipid peroxidation level in plants exposed to different environmental stresses [31]. In this study, PHE caused a significant increase of the MDA content throughout the treatment range in wheat seedlings. At the same time, an increased accumulation of H2O2 was observed and occurred in a dose-dependent manner. These observations suggest that the accumulation of ROS may have exceeded the capacity of the plants’ antioxidant systems, and the activities of SOD, CAT and GPX were decreased, and lipid peroxidation has occurred. Finally, the cellular membrane damage is unavoidable, especially at high PHE concentrations. This excess ROS accumulation may cause a reduction in germination, root and stem growth. ROS accumulation may contribute directly or indirectly to the decrease of chlorophyll levels [14].
The results of this study indicated that PHE's toxicity could be attributed to an oxidative stress by ROS, which was consistent with previous reports. In the future, further research about the molecular mechanism of PHE's toxicity should be carried out to elucidate the PAHs’ toxicity mechanism fully.
5 Conclusions
In conclusion, PHE treatment inhibited, especially at higher concentrations, the germination of wheat seed and caused the reduction of growth and chlorophyll content, an increase of MDA contents and a decline of many enzymes activities, such as SOD, CAT and GPX of wheat seedlings, which might be ascribed to excess ROS such as O2−• and H2O2 in plant cells, which were induced by PHE. The results indicated that PHE could provoke oxidative damages in the early development stage of wheat, which occurred mainly in samples exposed to higher concentrations of this compound.
Disclosure of interest
The authors declare that they have no conflicts of interest concerning this article.
Acknowledgements
The work was supported by the National Nature Foundation of China (No. 21177078) and the International cooperation projects (No. 41211140241), the Natural Science Foundation of Shanxi Province (No. 2011011009-2).