1 Introduction
Two-component systems (TCS) are signal transduction mechanisms based on phosphotransfer reactions between histidine and aspartate residues that are used by living cells to sense and respond to extracellular or intracellular processes [1,2]. TCSs are prevalent in prokaryotes, and these signaling cascades are also present in eukaryotes, such as fungi and plants. The prototypical prokaryotic TCS contains a simple phosphotransfer mechanism composed of two signaling proteins, the transmembrane sensor histidine kinase (HK) and the effector response regulator (RR) proteins [3,4]. The modular nature of TCS proteins has originated a wide range of sensory and signaling domain architectures in prokaryotes [1,2,5].
In a typical prokaryotic TCS system, the sensor HK protein generally contains a functional transmitter module (T) characterized by two structurally conserved domains: a dimerization/histidine phosphotransfer domain (P) that typically is a HisKA, and an adjacent HATPase domain [5]. The HATPase domain binds ATP and catalyzes the autophosphorylation of the conserved His residue found within the dimerization/histidine phosphotransfer domain in response to a perceived signal. The phosphoryl groups are subsequently transferred to the Asp residue in the receiver domain (R) of the RR protein. On the other hand, classical hybrid HKs carry both the transmitter module (T) and the receiver domain (R) within a single polypeptide, and are involved in consecutive phosphorylations between the conserved His and Asp residues, known as phosphorelay TCS [6,7].
Fungal TCS signaling cascades generally are more complex with a multi-step His–Asp phosphorelay composed of 3 functional modules: a hybrid HK, a histidine-containing phosphotransfer (HPt) protein, and a RR protein [8,9]. Fungal TCS proteins are components of complex signal transduction pathways with essential roles in the regulation of several cellular functions and responses [10].
Basidiomycetes are a large and diverse fungal phylum that comprises 3 subphyla: Agaricomycotina, Pucciniomycotina and Ustilaginomycotina [11–13]. Studies of the TCS pathways in the human pathogen Cryptococcus neoformans have identified the unusual domain architecture of Tco2 and Tco4 hybrid HKs containing 2 HK and 2 RR domains [8,14]. The function of C. neoformans Tco4 remains unclear, and Tco2 is responsible for drug sensitivity and osmotic and oxidative stress and has a redundant role with Tco1 in activating the high osmolarity glycerol (HOG) mitogen-activated protein kinase (MAPK) pathway [8,14]. In previous comparative genomics studies of basidiomycetes, we have shown that the presence of Tco2 and Tco4 orthologs is limited to some particular species of basidiomycetes and they are absent in the other fungal phyla [15,16]. Considering the domain structure of this protein family of hybrid HKs (2 HK and 2 RR domains), we named them dual-HKs. In the present manuscript, we extend the analysis of dual-HKs to 80 fully sequenced genomes of basidiomycetes, and we show that the atypical domain architecture of dual-HKs is similar to that of some other bacterial hybrid HKs.
2 Materials and methods
2.1 Sequence data
Proteomes encoded in 80 fully sequenced genomes of basidiomycetes were obtained from MycoCosm (as of June 2013) (http://jgi.doe.gov/fungi) [17]. For consistency, we used in our analysis the species names as provided by the database source.
2.2 Identification and analysis of dual-HKs
Genes coding for dual-HKs in the complete genome sequences of basidiomycetes were identified using the pipeline web server BASID2CS (http://bioinformatics.unavarra.es:1000/B2CS/BASID2CS.htm) [16]. Recently, we have developed this bioinformatics platform for the identification, classification and functional annotation of putative TCS proteins from any genome of basidiomycetes. A Hidden Markov Model (HMM) targeting dual-HKs was used, and hits with an E-value below a defined cutoff (10−20) were extracted. In addition, genome sequences were also analyzed using TBLASTN [18]. Functional domains of dual-HKs were identified by searching the Conserved Domain Database (CDD) with Reversed Position Specific BLAST [19] or the PROSITE database with the ScanProsite tool [20]. Multiple-sequence alignments were performed using MUSCLE [21] or Clustal Omega [22]. Prediction of transmembrane spanning regions was carried out using TMHMM v 2.0 (http://www.cbs.dtu.dk/services/TMHMM-2.0/) [23].
2.3 Phylogenetic analysis
Phylogenetic analysis was performed on whole-protein sequences using the Phylogeny.fr platform (http://www.phylogeny.fr) [24] and comprised the following steps. A multiple-sequence alignment of dual-HK protein sequences was generated using MUSCLE v3.7 [21] configured for highest accuracy (MUSCLE with default settings). After alignment, ambiguous regions (i.e. containing gaps and/or poorly aligned) were removed with Gblocks v0.91b [25] using the default settings and a minimum length of a block after gap cleaning of 10. A phylogenetic tree was reconstructed using the maximum likelihood method implemented in the PhyML program v3.0 aLRT [26,27] using the default settings. Reliability for internal branch was assessed using the bootstrapping method with 100 bootstrap replicates. Graphical representation and edition of the phylogenetic tree were performed with TreeDyn v198.3 [28].
3 Results
3.1 Dual-HKs in basidiomycetes
In this work, we have analyzed the presence of genes coding for dual-HKs in 80 complete genome sequences of species belonging to the phylum Basidiomycota using the web server BASID2CS [16]. This study includes 71 genome sequences of species from the subphylum Agaricomycotina, six from the subphylum Pucciniomycotina (Cronartium quercuum, Melampsora laricis-populina, Mixia osmundae, Puccinia graminis, Rhodotorula graminis and Sporobolomyces roseus) and two from the subphylum Ustilaginomycotina (Malassezia globosa and Ustilago maydis), as well as one species classified as Basidiomycota incertae sedis (Wallemia sebi) (Table S1, Supplementary data). Dual-HKs are only present in 16 of the 71 Agaricomycotina and in all the Pucciniomycotina species analyzed. However, they are absent in M. globosa, U. maydis and W. sebi. When present, there is a single or two dual-HKs in each species (Table 1). Dual-HK genes are mainly scattered across the genomes of basidiomycetes. However, it is noteworthy that Rickenella mellea, Piriformospora indica, Sebacina vermifera, R. graminis and Sistotremastrum suecium contain two dual-HK genes oriented in the same direction and mapping relatively close in the chromosome. The distance between the pair of dual-HK genes is 882 bp in S. vermifera, 10,430 bp in S. suecium, 10,726 bp in P. indica, 30,868 bp in R. graminis and 135,241 bp in R. mellea. Moreover, both dual-HK proteins are highly similar in S. vermifera (86%) and P. indica (80%). Analysis of the P. indica genome has provided evidence for a high number of expanded protein families, which are typically present in clusters of 2 to 7 genes within the genome, and it has been proposed that expansion of these genes is likely to be due to local duplication events [29].
Dual-HKs in basidiomycetes.
Species | Subphylum | Dual-HKs |
Cryptococcus neoformans var. neoformans JEC 21 | Agaricomycotina | CNH02350 (Tco2) CNG02240 (Tco4) |
Auricularia delicata SS-5 | Agaricomycotina | Aurde1_156314 |
Cronartium quercuum f. sp. fusiforme G11 | Pucciniomycotina | Croqu1_77426 |
Cryptococcus neoformans var. grubii H99 | Agaricomycotina | Cryne_H99_1_5046 Cryne_H99_1_3001 |
Cryptococcus vishniacii | Agaricomycotina | Cryvi1_326214 Cryvi1_309956 |
Cylindrobasidium torrendii | Agaricomycotina | Cylto1_143793 |
Dioszegia cryoxerica | Agaricomycotina | Diocr1_362515 Diocr1_379172 |
Gymnopus luxurians | Agaricomycotina | Gymlu1_240082 |
Melampsora laricis-populina | Pucciniomycotina | Mellp1_115552 |
Mixia osmundae IAM 14324 | Pucciniomycotina | Mixos1_53668 |
Piriformospora indica DSM 11827 | Agaricomycotina | Pirin1_78110 Pirin1_78115 |
Puccinia graminis | Pucciniomycotina | Pucgr1_35920 |
Punctularia strigosozonata HHB-11173 SS-5 | Agaricomycotina | Punst1_126249 Punst1_146276 |
Rhodotorula graminis strain WP1 | Pucciniomycotina | Rhoba1_1_54592 Rhoba1_1_54600 |
Rickenella mellea | Agaricomycotina | Ricme1_650380 Ricme1_684267 |
Schizophyllum commune H4-8 | Agaricomycotina | Schco2_106551 Schco2_258402 |
Sebacina vermifera MAFF 305830 | Agaricomycotina | Sebve1_60912 Sebve1_326192 |
Sistotremastrum suecicum | Agaricomycotina | Sissu1_1046495 Sissu1_1061683 |
Sporobolomyces roseus | Pucciniomycotina | Sporo1_24426 |
Stereum hirsutum FP-91666 SS1 | Agaricomycotina | Stehi1_103850 Stehi1_107911 |
Tremella mesenterica Fries | Agaricomycotina | Treme1_69291 |
Tulasnella calospora AL13/4D | Agaricomycotina | Tulca1_18753 |
3.2 Domain structure of dual-HKs
The prokaryotic hybrid HKs have diverse and complex domain architectures and, therefore, have been classified according to the number and order of their T module and R domain [5,30]. In bacteria and fungi, the most common and simple domain architecture of hybrid HKs is the TR geometry containing a single T module and R domain (Fig. 1) [5,7,14,15,30]. However, analysis of the domain structure of the 34 dual-HKs identified in basidiomycetes showed that all of them have a different domain organization not reported among any other fungal hybrid HKs excepting diverse species of basidiomycetes [8,14,15]. Dual-HKs are complex hybrid HKs containing in a single polypeptide two HK transmitter modules (T) and two RR receiver domains (R) that are combined in a TRTR geometry (Fig. 1), and all this points towards a different type of TCS phosphorelay.

Schematic drawing of the two domain architectures of hybrid HKs. A. TR geometry of typical hybrid HKs commonly found in bacteria and fungi containing a single transmitter module (T) and a receiver domain (R). B. TRTR geometry of dual-HKs in basidiomycetes containing two T modules and two R domains plus an internal PAS domain. HisKA, dimerization/phosphoaceptor domain; HATPase_c, HK-type ATPase catalytic domain; REC, receiver domain; PAS, Per-Arnt-Sim domain.
Furthermore, the majority of dual-HKs contain an internal Per-Arnt-Sim (PAS) domain (Fig. 1). PAS domains perform a variety of functions within signal transduction proteins by promoting protein/protein interactions, signal transfer or directly sensing perceived stimuli, such as dissolved gases, redox potential or visible light [31]. Additional N- or C-terminal domains were absent in dual-HKs, except for the N-terminal M14 metallocarboxipeptidase (MCP) domain in Punctularia strigosozonata Punst1_146276, and the C-terminal Major Facilitator Superfamily (MFS) domain in Auricularia delicata Aurde1_156314 (data not shown). The presence of these N- or C-terminal domains most likely indicates that Punst1_146276 and Aurde1_156314 gene models could be incorrectly predicted during the genome annotation process.
Dual-HKs are unusually large signalling proteins ranging in size from 1028 to 2750 aa. A large number of fungal hybrid HKs is not predicted to have transmembrane domains [3,8,9]. The lack of predicted transmembrane helices in the N-terminal end is a common feature of all dual-HKs in basidiomycetes and suggests that they are not membrane-associated proteins.
3.3 Phylogenetic analysis of dual-HKs
In order to evaluate potential relationships between dual-HKs of basidiomycetes, we performed a phylogenetic analysis (Fig. 2). The overall branching pattern of the phylogenetic tree derived from the protein sequences of dual-HKs is broadly consistent with the phylogenetic classification of the phylum Basidiomycota [11]. Dual-HKs of species from Pucciniomycotina and Agaricomycotina are clustered separately, and a clear clustering pattern can be observed at the level of class in both subphyla (Fig. 2). The only major exception is represented by the Aurde1_156314 protein sequence that appears to have little affinity with other Agaricomycotina dual-HKs.
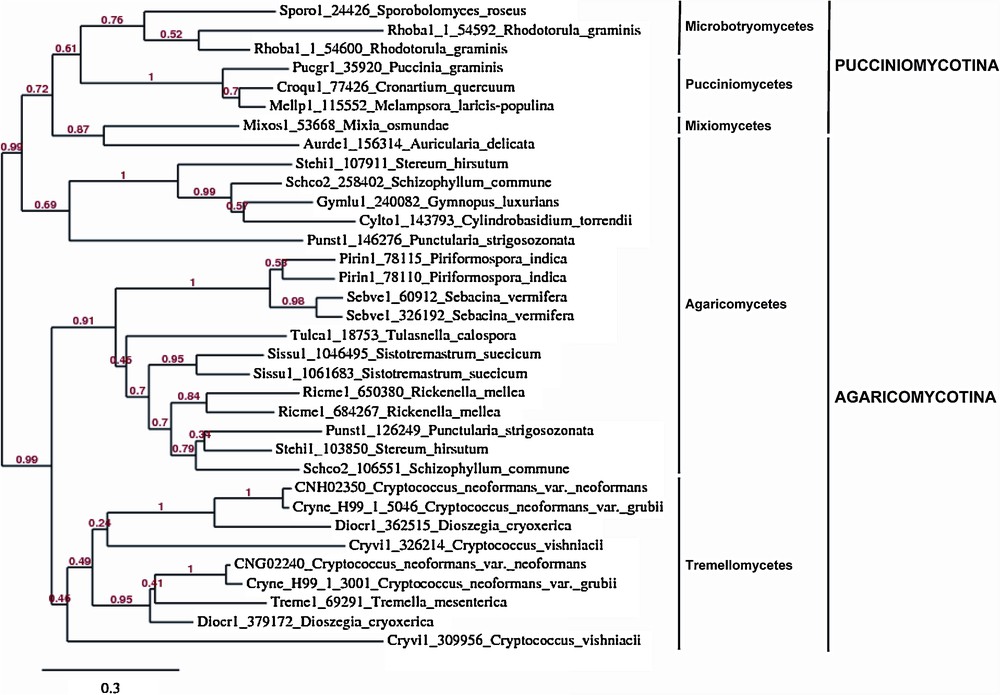
Maximum likelihood phylogenetic tree for dual-HKs of basidiomycetes. Numbers at nodes are bootstrap values. Clusters corresponding to the different classes and subphyla of basidiomycetes are indicated by dashes beside the tree. The horizontal bar represents the scale for the average number of substitutions per site.
4 Discussion
The availability of complete genome sequences has made possible to assess the genomic complements of TCS proteins within the phylum Basidiomycota [15,16]. Here, we extend the analysis of dual-HKs to 80 genomes of basidiomycetes and confirm that this protein family is limited only to some particular species of this fungal phylum. These atypical hybrid HKs with a particular TRTR domain arrangement could contain multiple routes of phosphotransfer within each protein and act as important points for TCS signal integration. It has been proposed that in TCS phosphorelays involving two T modules and multiple R domains the P domain within the second T module could carry out a function similar to the P domain of the HPt protein in classical TCS phosphorelays of hybrid HKs with TR geometry [5].
In addition to the typical TR geometry of hybrid HKs, complex combinations of the T module and R domain have been described in bacteria, particularly in the cyanobacteria and myxobacteria [5,7,30,32]. Similar to dual-HKs of basidiomycetes, hybrid HKs with a TRTR domain architecture encoded in a single gene have been identified in the myxobacteria Sorangium cellulosum (sce0713, sce3507) and Stigmatella aurantiaca (STIAU_3449) [30], the cyanobacteria Anabaena variabilis (AVA_1086) and Nostoc punctiforme (NPUN_F2346, NPUN_F2908), and in other bacteria, such as Bradyrhizobium sp. (BBTA_5808, BRADO5320), Delftia acidovorans (DACI_4352), Ralstonia eutropha (REUT_B4539) and Rhodopseudomonas palustris (RPD_1666, RPB_3818, RPAL_4456) [5]. Most bacterial hybrid HKs with TRTR domain architecture were also found to contain an internal PAS domain similar to dual-HKs, and furthermore some of these proteins possessed additional signal sensor PAS and/or GAF domains. Up to date, the role of these bacterial hybrid HKs has not been experimentally characterized.
The evolution of prokaryotic hybrid HKs to complex domain architectures could be originated by sequential addition of individual TCS domains or alternatively by recombination between simple hybrid HKs [7]. Similar events may perhaps give rise to hybrid HKs with TRTR domain architecture in basidiomycete fungi.
Disclosure of interest
The authors declare that they have no conflicts of interest concerning this article.
Acknowledgments
These sequence data were produced by the US Department of Energy Joint Genome Institute http://www.jgi.doe.gov/ in collaboration with the user community. This work was supported by research projects AGL2011-30495 of the Spanish National Research Plan, Bioethanol-Euroinnova from the Autonomous Government of Navarre and by additional institutional support from the Public University of Navarre.