1 Introduction
Boswellia papyrifera and Boswellia carterii, generally known as Arabian incense, are used in Ayurveda medicine for the treatment of arthritics [1,2]. Their oil has long been used in cologne industries. It is also practiced in religious ceremonies of many countries, especially in Middle East regions. Further, scientific studies also suggest that boswellic acids exert significant anticancer, antimicrobial and immune-potentiating effects [3,4]. The chemical properties of these plants were well characterized, and they contain isoincensole and incensole acetate as main diterpenic components. These plants belong to a single family, Burseraceae [5].
In addition to its therapeutic values, it causes severe pulmonary changes and decreases the ability of lung function after animals have been exposed to it [6,7]. Furthermore, the toxic effect of Arabian incense in rat liver significantly decreases the levels of alkaline phosphatase (ALP), alanine aminotransferase (ALT), and aspartate aminotransferase (AST). It also reduces the levels of glutathione (GSH), superoxide dismutase (SOD), catalase (CAT), and glutathione peroxidase (GPx). However, incense smoke significantly increased lipid peroxidation [8].
Long-term exposure to incense smoke was associated with decreased weight and adverse metabolic changes of increased triglycerides and decreased HDL-cholesterol concentrations. Exposure to incense was also associated with a transient increase of leptin levels [9]. Recent studies exposed to B. papyrifera and B. carterii on rat testis and epididymis demonstrated that it severely affects the histoarchitecture of these organs by light and transmission electron microscopes [10,11]. Taken all together, these findings suggest that incense smoke influences metabolism adversely in rats.
A relative number of studies have been reported on pathological and pharmacological effect(s) of these plants on some vital (lung, liver) and reproductive organs such as testis and epididymis. However, the information related to the effects of incense smoke on hormonal assay, biochemical composition, and function of cauda epididymal spermatozoa, are limited. Therefore, the present investigation aims at exploring the potential toxic effects of these incenses on rat cauda epididymal spermatozoa and the dysfunction they provoke there.
2 Materials and methods
2.1 Animals and incense
Wistar albino male rats (Rattus norvegicus) aged 7–8 weeks, weighing 200–210 g, were obtained from the Animal Care Center, College of Science, King Saud University, Riyadh, Saudi Arabia. The Ethical Committee of the Experimental Animal Care Center approved the study. Animals were housed in a temperature-controlled room on a 12-h light/dark cycle and had access to water and normal chow diet ad libitum. B. papyrifera (crude incense) and B. carterii (refined incense) were obtained locally.
2.2 Exposure to B. papyrifera and B. carterii smoke
After 1 week of acclimatization period, the rats were randomly divided into three groups, viz. groups I, II and III, with each group consisting of 11 animals. Each group of rats was housed separately from the other groups to avoid the cross exposure to incense smoke. Rats in group I served as control and were kept in normal conditions, while rats in group II and III were exposed to B. papyrifera and B. carterii smoke respectively, in a smoking chamber as described by Wang et al. [12]. The rats were exposed daily to the smoke emanating from the burning of 4 g of each incense material for 4 months. Smoke exposure durations lasted for 30–35 min/day. The dose and duration of incense exposure followed in this study were based on the optimized conditions from our lab studies [10,11]. At the end of exposure duration, all animals were killed by cervical dislocation.
2.3 Blood collection and hormonal assay
After 4 months of treatment, blood samples were collected from each group. They were kept at room temperature for approximately 30 min. Then, the tubes were centrifuged at 2500 rpm for 15 min, the supernatants were collected, and the serum was used for analyzing the hormone level. Serum concentrations of follicle stimulating, luteinizing and testosterone hormones (Diagnostic System Laboratories kits [DSL], USA) were measured following an immunoenzymatic method with an ELISA reader, according to the standard protocol given in the assay kit.
2.4 Evaluation of biochemical compositions
The epididymal plasma was collected by cutting the epididymides cauda in halves, and then by carefully scraping and aspirating with an insulin syringe without a needle, then by centrifugating the sample at 12,000 g for 30 min in an Eppendorf centrifuge 5415 C apparatus. The epididymal plasma was stored at −40 °C before the specific spectrophotometric determination of proteins, sialic acid, carnitine, and carbohydrates. The biochemical compositions were estimated according to Huacuja et al. [13].
2.5 Sperm analysis
The cauda epididymis was carefully squeezed into phosphate buffered glucose saline (PBGS); and a clear suspension was obtained. The epididymal plasma was used for total sperm count, sperm motility, forward velocity and relative percentage of abnormal sperms determination. The total sperm count and motility were calculated according to the method of Besley et al. [14], using a Neubauer hemocytometer. The spermatozoa were allowed to settle down in the hemocytometer for total sperm count by keeping them in a humid chamber (4 °C) for one hour. The sperm count was recorded in R.B.C. counting chambers [15]. Similarly, the total numbers of motile sperm cells were calculated immediately using a saline phosphate buffer instead of spermicidal solution and the time gap between counts were 0.5 to 10 s. The forward sperm velocity was calculated according to Ratnasooriya [16]. The assessment was made under the light microscope, fitted with a movable mechanical stage and a calibrated ocular micrometer, at 400× magnification. A drop of sperm suspension was transferred to a clean glass slide and the initial place and time of each sperm were recorded. The time taken for forward movement of the sperm from the initial place within the microscopic field was recorded using a stopwatch. The procedure was repeated for 10 spermatozoa for each sample and the average forward velocity of the sperm was calculated and expressed in μm/s. The relative proportions of abnormal sperms were analyzed by Bauer et al. [17]. Briefly, equal volumes of cauda epididymal plasma and 5% NaHCO3 were placed in a centrifuge tube, mixed well and centrifuged for 5 min at 4000 rpm. The supernatant was discarded and 5 mL of normal saline solution was added to the precipitate, mixed well and centrifuged again. The procedure was repeated two to three times until a clear precipitate was obtained. To the final precipitate, a few drops of normal saline solution were added, mixed thoroughly and a smear was prepared on a clean slide. The smear was dried at room temperature, fixed by heating over the flame for two to three seconds. Then, the smear was flushed with 95% alcohol, drained, and dried. Smear was stained in Ziehl Neelson's Carbol Fuchsin diluted with equal volumes of 95% alcohol for 3 min and counter stained with a 1:3 (v/v) aqueous solution of Loeffer's methylene blue for 2 min. After staining, the smear was rinsed in water and dried in the air. The abnormal sperms were characterized based on the presence of double tails, detached heads, detached tails, midpiece bending, and irregular heads. The relative proportions of normal and abnormal sperm were expressed in terms of percentages.
2.6 Ultrastructural study
Immediately after removal of cauda epididymis from the dissected rats, tissues were sliced into small-size pieces (1 mm3) and fixed in 3% buffered glutaraldehyde for 4 h at 4 °C. Tissue specimens were then post-fixed in 1% osmium tetraoxide (OsO4) for 1 h 30. Dehydration of the fixed tissues was performed using ascending grades of ethanol and then the tissues were transferred to resin via propylene oxide. After impregnation with the pure resin, tissue specimens were embedded in the same resin mixture [18]. Ultra-thin sections (70–75 nm) were cut on an ultra-microtome (Leica, UCT) with a diamond knife (Diatome, Switzerland) and stained with uranyl acetate and lead citrate. Sections were observed under TEM (JEOL 1011 CX) operating at 80 kV.
2.7 Statistical analysis
Data were expressed as mean values ± SE. Student's t-test was applied to compare the significance of difference. A probability level P < 0.05 or less was accepted as significant.
3 Results
3.1 Body weight and organ weights
No evident changes were noticed in the body weight of the reproductive organs in control as well as in treated groups (data not shown).
3.2 Hormone assay
Table 1 showed the diminished levels (P < 0.001) of circulating follicle stimulating hormone (FSH), luteinizing hormone (LH), and testosterone (T) in the serum after exposure to B. papyrifera and B. carterii.
Effect of B. papyrifera and B. carterii on serum hormones level.
Groups | Blood plasma level (ng/mL) | ||
FSH (mIU/mL) | LH (mIU/mL) | Testosterone (ng/mL) | |
Control | 1.36 ± 0.13 | 3.80 ± 0.10 | 0.67 ± 0.15 |
B. papyrifera | 00.53 ± 0.11 | 2.29 ± 0.11 | 0.24 ± 11 |
B. carterii | 00.49 ± 0.14 | 2.30 ± 0.27 | 0.19 ± 15 |
3.3 Estimation of biochemical compositions
The biochemical composition of the cauda epididymal plasma displayed a significant decrease in proteins, sialic acid, and carnitine in B. papyrifera and B. carterii groups when compared to the control group (Table 2; P < 0.001). At the same time, no significant changes were observed in carbohydrates of all groups.
Biochemical composition of cauda epididymal plasma exposed with B. papyrifera and B. carterii.
Groups | Protein | Total carbohydrate | Sialic acid | Carnitine |
Control | 4.85 ± 0.80 | 0.77 ± 0.19 | 3.10 ± 0.36 | 0.39 ± 0.01 |
B. papyrifera | 2.33 ± 0.10 | 0.71 ± 0.07 | 1.19 ± 0.05 | 0.17 ± 0.03 |
B. carterii | 2.10 ± 2.78 | 0.75 ± 0.11 | 1.21 ± 0.51 | 0.15 ± 0.09 |
3.4 Sperm analysis
Sperm parameters including total sperm count, total number of motile sperm (usually motile sperm swim forward in an essentially straight line, whereas non-motile sperm swim, but following abnormal paths, such as circles), forward velocity and percentage of abnormal sperm, fructose content of epididymal and seminal plasma were compared between exposed and non-exposed control rats. Relative to the control group, the animals exposed to B. papyrifera and B. carterii showed a significant decrease in the total sperm count, the total number of motile sperm and the forward velocity of the sperm (Fig. 1, P < 0.001). The percentages of abnormal sperm were increased (P < 0.001).

Sperm parameters.
3.5 Ultrastructural changes in cauda epididymal spermatozoa
In ultrastructural studies, control rats exhibited normal spermatozoa (Fig. 2A–D). The perforatorium and acrosome were enclosed with the plasma membrane (Fig. 2B and C). An intact acrosome was covered with an acrosomal membrane. The whole spermatozoon was intact with all the membranes and organelles (Fig. 2C). Conversely, animals exposed to B. papyrifera and B. carterii evidence a disruption in the plasma and acrosomal membranes. The surface became rough, with accumulated debris and vague materials (Figs. 3A–D and 4A and B). The tip of the sperm head showed a disruption of the plasma membrane as well as of the acrosome (Figs. 3A–C and 4A and B). The perforatorium was condensed and most of its surface was covered by a thin layer of acrosomal sac (Figs. 3A–C and 4A and B). Many serrations were observed in the head region of the spermatozoa. The shape and size of the sperm head has also changed considerably (Figs. 3A–C and 4A and B). There was a severe dorsoventrally constriction in the mid-head region in most sperms. The perforatorium was bulged/swelled (Fig. 3A–C). The anterior and caudal portions of the sperm heads revealed a loss of the plasma membrane and of the acrosome, and present small vesicles on the ventral surface of the perforatorium (Figs. 3A–C and 4A and B). The tail sections in sperms exposed to B. papyrifera and B. carterii showed disruption and complete degeneration of the mitochondrial sheath and a loss of plasma membrane (Figs. 3A and 4B). The commencements of degeneration of mitochondria were observed in almost all sheaths, either on one or both sides, and there was an abnormal pattern of outer dense fibers (Figs. 3B and C and 4B–D). Different parts of the tail region exhibit discontinuation of plasma membrane and fibrous sheath, respectively (Figs. 3A and 4B). Most of the spermatozoa showed a splitting of the tail and distinct visibility of balloon-like cytoplasmic droplets in the mid-region of the tail (Figs. 3D and 4C and D).
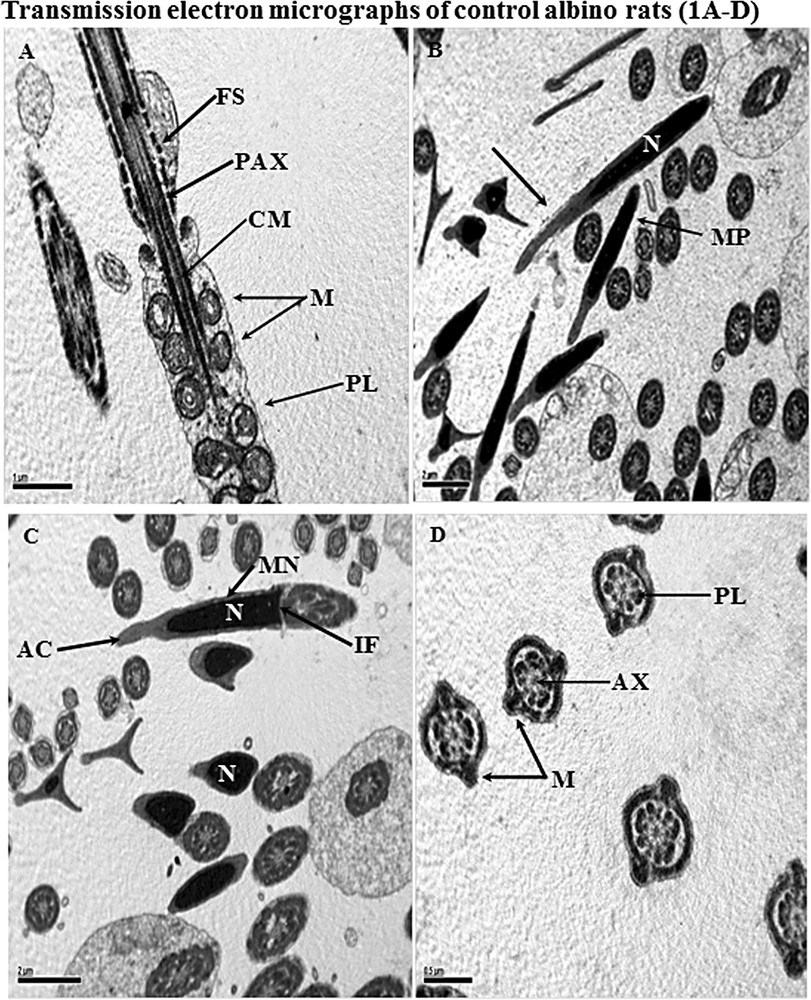
Transmission electron micrographs (TEM) of samples taken from control albino rats. A. A longitudinal section of a spermatozoon showing the midpiece along its tail region. In this section, entire features of the tail region are normal. The plasma lemma (PL) is covered with whole spermatozoa. Concentric round mitochondria (M); central microtubules (CM); peripheral of axonemal complex (PAX); and fibrous sheath (FS) all are normal in structure. B and C. Longitudinal section (LS) of the anterior portion of a sperm head illustrating normal features of nucleus (N); perforatorium (arrow); acrosome (AC); implantation fossa (IF) and plasma membrane (PM). Many CS of the tail region are also present, showing normal features. D. Cross-sections of the tail region, showing 9 + 2 microtubules as well as a central axoneme (AX). Plasma lemma (PL); and mitochondria are normal.

Transmission electron micrographs (TEM) of samples taken from albino exposed to B. papyrifera. A and B. Cross and longitudinal sections of the different parts of sperm head (←) showing complete disruption in normal features plasma membrane, acrosome, and perforatorium. In all sections of the sperm head, necrotic nuclei are seen. Sperm cells are also covered with fuzzy material on their surfaces. C. Longitudinal section (LS) of the midpiece showing the loss of plasma membrane (←). Most of mitochondria (not shown) are hypertrophied or have started degeneration. The loss of the plasma membrane is also seen in the sperm head region (←). The fibrous sheath is completely damaged (FS). D. Cross-sections of the tail and midpiece regions showing abnormal pattern of mitochondrial (*) and loss of plasma membrane. Most part of the midpiece shows increased cytoplasmic droplets (CD) displaced on one side. Coating of frothy and fuzzy material is seen on the surface of the tail sections. Exposed with B. carterii on albino rats (Fig. 3A–D).
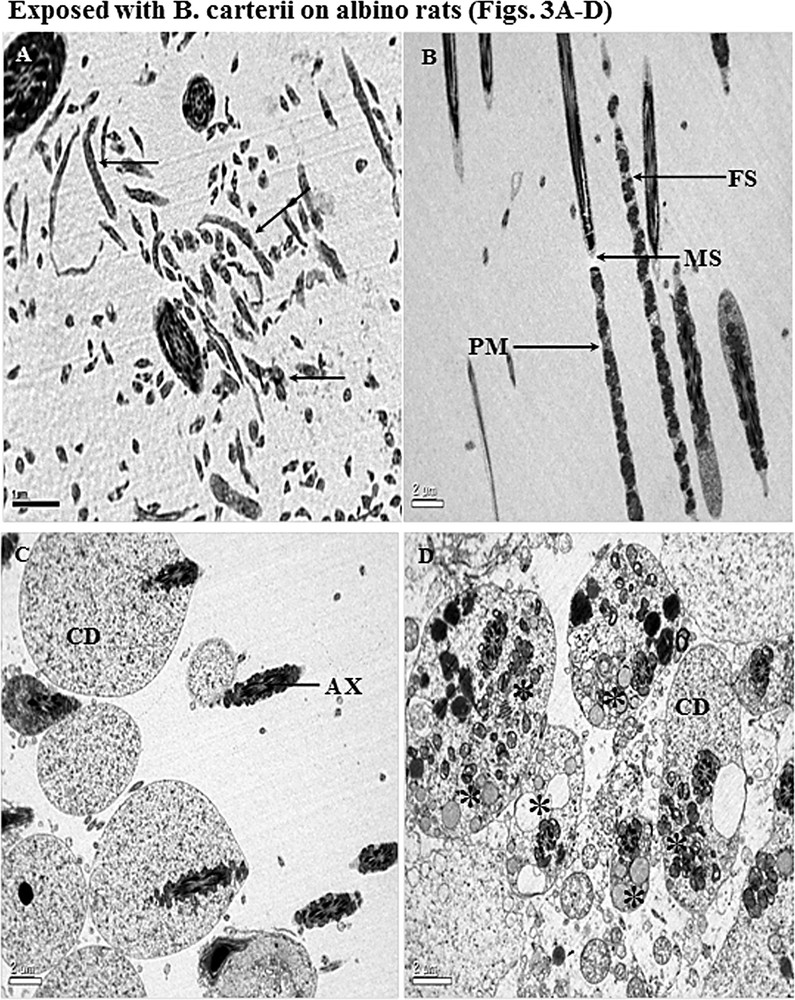
A. Cross and longitudinal sections of the different parts of sperm heads (←) showing complete disruption in plasma membrane, acrosome, and perforatorium. The sections of the sperm heads are clearly not recognizable in their features, and all the parts of the head region are disturbed, surrounding the debris. B. Longitudinal section (LS) of midpiece and principal piece illustrating well-dilapidated mitochondrial sheath (MS) and fibrous sheath (FS). Plasma membrane (PM) is broken with abnormal mitochondria (M). Fibrous sheath (FS) arrangement of outer fibers and axonemal component are completely damaged. C and D. Cross-sections of the tail regions showing an abnormal pattern of arrangement of microtubules (AX), central axoneme and damaged mitochondria (*). Most of the midpiece shows increased cytoplasmic droplets (CD). Unwanted fuzzy material is scattered everywhere with cell debris.
4 Discussion
In reproductive physiology, the process for survival of the species is controlled by a neuroendocrine axis and involves hypothalamus, anterior pituitary gland, and gonads. This hypothalamo–pituitary–gonadal (HPG) axis is comprised, at its most fundamental element, of GnRH-expressing neurons that, in the rodent, are located in the rostral forebrain. Neurons, through numerous routes direct processes to release GnRH into the hypothalamo–hypophyseal portal vasculature, where it eventually acts upon the gonadotropes of the anterior pituitary to stimulate the release of the gonadotropins, luteinizing hormone (LH) and follicle stimulating hormone (FSH) [19]. The cauda epididymal region is an important male reproductive tract that is highly androgen-dependent and plays a vital role in male fertility [20]. Cauda epididymis provides a suitable environment for morphological and biochemical changes in spermatozoa [21]. It performs both secretory and absorptive functions. Androgen deficiency causes a marked reduction in the tubular diameter, a general regression of epididymal epithelium, a sudden decline in spermatozoa, and changes in the composition of epididymal plasma [22]. In this study, we evaluated the toxicity of B. papyrifera and B. carterii smoke exposure on cauda epididymal spermatozoa, on some biochemical compositions, and on the levels of gonadotropic hormones such as FSH, LH, and testosterone. In our recent studies, we had already established the pathological and ultrastructural changes in testis and cauda epididymis in male albino rats [10,11].
In this study, animals exposed to B. papyrifera and B. carterii exhibit a significant increase in sperm anomalies with reducing sperm count, motility, and sperm speed (Fig. 1); at the same time, the biochemical content of cauda epididymis in compounds such as proteins, sialic acid, and carnitine is reduced (Table 2). Furthermore, the hormonal levels of FSH, LH and testosterone are also reduced (Table 1) [23,24]. The epididymis plays an active role in sperm development and in the sperm maturation process, including its specific proteins secreted by the epididymal epithelium [25]. Carnitine is the most important metabolite that helps in epididymal sperm maturation, motility, and fertilizing capacity (80%) [26,27]. In our findings, the considerable decline in carnitine, sialic acid, and protein concentrations in cauda epididymal plasma is due to the toxic effect of these plants. Carnitine has been shown to have an important cellular function, for example by transferring long-chain fatty acids across the inner mitochondrial membrane for β-oxidation [28].
LH and FSH are called gonadotropins. In testis, LH binds to receptors on Leydig cells that secrete testosterone [26]. In our study, low levels of LH, FSH and testosterone hormones were observed, which suggests that these plants could be toxic, preventing the synthesis and maturation of spermatozoa [26,27,29]. Testosterone stimulates Sertoli cells to synthesize nutrients for the development of spermatozoa, androgen binding protein, and inhibin. The synergistic effect of FSH and testosterone accelerates spermatogenesis so that a large number of spermatozoa are produced in the lumen of the seminiferous tubule. An excessive number of Sertoli cells cause a high level of nutrient production in the cells to meet the requirements of nourishment from spermatozoa. In contrast to the results of this study, it decreases considerably the levels of FHS, LH and testosterone due to the toxic effect of these plants [24,30]. By means of all above findings, it is clear that our plants have some potential toxicity that hampers the process of spermiogenesis, as it had been reported in our previous studies [10,11].
Furthermore, this study was undertaken to determine the effects of B. papyrifera and B. carterii on the ultrastructure of rat epididymal sperm. The results significantly confirm that the epididymal sperm of rats exposed to B. papyrifera and B. carterii exhibit distinct ultrastructural changes, such as major damage to almost all sperm flagella in the cauda epididymal region. The primary and foremost defect is the degeneration of the midpiece mitochondria. Not surprisingly, caudal spermatozoa are totally immotile by their exposers, as described in previous studies [11,31]. There were numerous ultrastructural changes of spermatozoa such as mitochondrial spiral, outer dense fibers and axoneme that are severely affected by these plants. Based on the present findings, it may be suggested that these plants can damage spermatozoa in the epididymis. Similar changes have been evidenced in additional studies on lead acetate and gossypol [30–32].
In rats, oral administration of triptolide at a dosage of 100 μg/kg per body weight for 70 days virtually affects all cauda epididymal sperm and exhibits a complete absence of plasma membrane over the entire middle and principal piece; furthermore, premature decondensation of the nuclei and disorganization of the mitochondrial sheath with many vacuolated mitochondria were taking place, which supports our hypothesis [33].
Many studies have suggested that the main structures present in the sperm head of mammals are the nucleus, the acrosome, and the membranous envelopes [34]. The mitochondrial sheath is believed to be the source of energy for sperm motility and outer dense fibers [35]. These outer dense fibers provide added strength to protect sperm from damage by shear forces encountered during epididymal transit or ejaculation [36]. The spermatozoa of the cauda epididymis in this study revealed several abnormalities, mainly abnormal patterns of the outer dense fibers and components of axoneme are displaced on either side; furthermore, complete absence of plasma membrane and disorganization of mitochondrial sheath in several spermatozoa were noticed [35,36].
Ultrastructural observations of treated animals showed that the tail region has cytoplasmic droplets that look like a balloon. Spermatozoa ejaculated with an attached cytoplasmic droplet would be correlated with altered epididymal function and reduced sperm potency [37,38]. The presence of high percentages of spermatozoa with cytoplasmic droplets in rats exposed to B. papyrifera and B. carterii may be due to an altered epididymal milieu. Similar observations were made in studies carried out with animals treated with substances such as Carica papaya extracts, vincristine, and aflatoxin B1 [39–41]. Agnes and Akbarsha [41] showed in their studies higher percentages of cytoplasmic droplets in cauda epididymal spermatozoa of albino mouse. Cytoplasmic droplets contained electron-dense spherical inclusions, commonly called lipid inclusions, secreted from the lamellae through the spherical vesicles of the cytoplasmic droplets. The pathological and physiological changes observed in the head and tail region of spermatozoa of rats exposed to B. papyrifera and B. carterii could be due to an overall disturbance in an epididymal milieu that secretes large portions of proteins and cytoplasmic inclusions. However, these findings are based on preliminary observations such as hormone assays, biochemical compositions, sperm kinetics, and ultrastructural studies. Further, in-depth enzyme kinetics determination, as well as the masking of these sites is required to find out its target mechanisms.
Disclosure of interest
The authors declare that they have no conflicts of interest concerning this article.
Acknowledgement
The authors would like to extend their appreciation to the Deanship of Scientific Research at King Saud University for its funding of this research through the Research Group Project No. RGP-VPP-300.