1 The olive tree: origins, dissemination and cultivation
Six Olea europaea subspecies with distinct geographic areas are distinguished [1,2] (Fig. 1):
- • subsp. europaea present in the Mediterranean Basin, with two botanical varieties: O. europaea subsp. europaea var. europaea for the cultivated forms, and O. e. subsp. e. var. sylvestris for wild and spontaneous trees (usually named oleasters) [2];
- • subsp. laperrinei in the Saharan mountains;
- • subsp. cuspidata from southern Africa to southern Egypt and from Arabia to China;
- • subsp. guanchica in the Canary Islands;
- • subsp. maroccana in southern Morocco;
- • subsp. cerasiformis in Madeira.
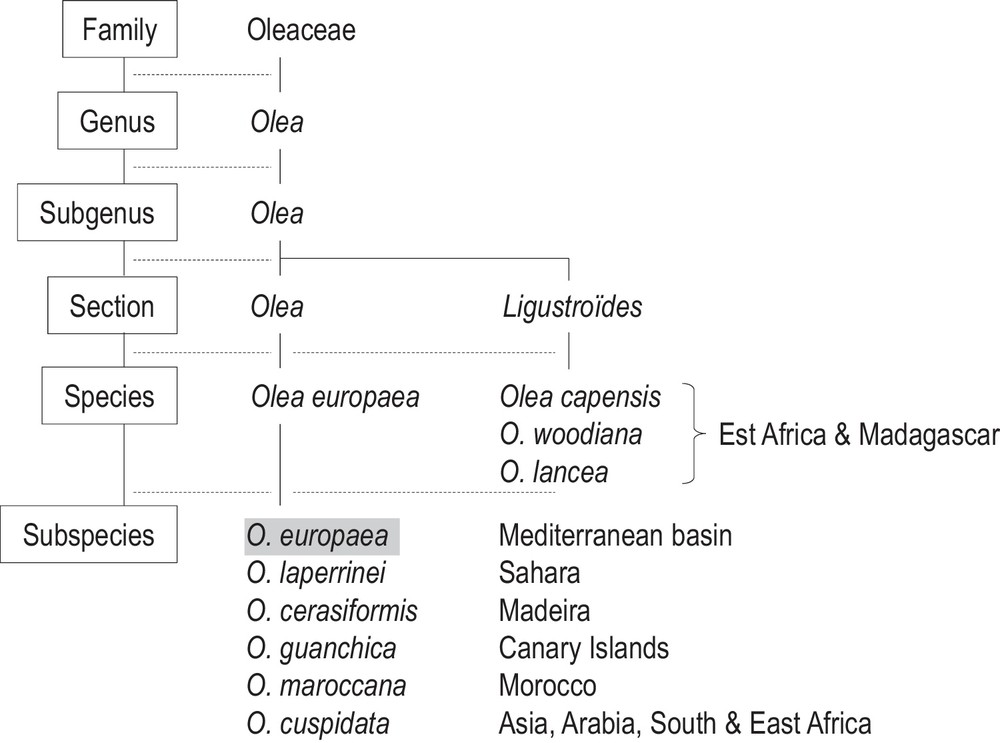
Simplified diagram of the Olea genus (Oleaceae) and biogeography of taxa [11].
The Mediterranean olive (Olea europaea L. subsp. europaea; Oleaceae) is one of the first domesticated tree species [3,4]. Its origins and domestication history are still highly debated, but archaeological and molecular data sustain that the first cultivars originated from the Levant, very likely 6000 years ago, in a region presently located at the border between southwestern Turkey and northwestern Syria [5,6]. Genetic studies indicated also that olive domestication is a long and on-going process and cultivars have originated from multiple source populations, as for grape and figs [7–9]. It is currently established that western wild olives secondarily contributed to the cultivated gene pool, while most of present cultivars remain mainly related to eastern wild olives [10].
The genetic diversity of oleaster is somewhat greater than that of the cultivated olive [6,10,11]. This diversity is highly structured between the East and the West of the Mediterranean Basin [6,12,13]. The analysis of plastid DNA polymorphisms allowed the identification of 48 distinct profiles delineating three diverging lineages. Lineage E1 is currently distributed over the whole Basin, whereas lineages E2 and E3 exclusively occur in western and central Mediterranean areas [6,14].
The olive tree has long been considered as the most typical Mediterranean tree. It has been widely disseminated through conquests and exchanges that accompanied the expansion of the Mediterranean civilizations (Phoenicians, Greeks, Romans, and Arabs) [15]. Populations of wild olives are restricted to a few isolated areas of native Mediterranean forests, where pollen/stones may be spread by natural factors (wind and birds) [13]. Most other wild-looking forms of olive may include feral forms (either cultivated olive abandoned and becoming wild or olive from stones of cultivated olive spread by birds). The olive dissemination was accompanied by a secondary diversification that has led to a high genetic variability that reflects a wide range of morphological (tree vigour, fruit shape, yield, oil content, etc.) and physiological (adaptation traits to environmental stresses, particularly those related to climate and soil constraints) characteristics that can explain its large distribution in the Mediterranean Basin [16,17]. This diversity can also result from a series of events related to human practices made to meet microclimate and terroir requirements and to satisfy consumer taste [12].
More than 2000 Mediterranean varieties have been recognised based on fruit, pit, and leaf shapes and colours, tree architecture and phenology (i.e. flowering time) [18–21]. Olive cultivars are propagated vegetatively by cutting or grafting. Most of the modern varieties display a maternal lineage (E1) that is distributed all around the whole Mediterranean area [6,14,22]. However, the genetic diversity of cultivated populations exhibits a complex patchy pattern [23,24].
For cultural (traditions, landscapes) and economic (oil and olives) reasons, this tree is regarded as one of the most cultivated plant in the world, ranking the 24th among 35 species [25]. It is now distributed in the five continents. It grows extensively in Mediterranean climate regions in Australia, South Africa, North and South America and covers about 11 Mha, with 98% located in the Mediterranean Basin [26,27]. Eighty percent of the cultivated olive surface is located in northern Mediterranean countries (Spain, Italy, Greece, Turkey), 17% in the Middle East (Jordan, Syria, Iraq, Iran) and in North Africa (Morocco, Algeria, Tunisia, Egypt), and only 2% is located in North (USA) and South (Mexico, Argentina, Peru) America [28,29] (Fig. 2).

World distribution of Olea europaea L. producing areas [26].
Olive diversity may also reflect a significant diversity of associated pests, some of them being specific. Major olive diseases are due to insects (e.g., the fruit fly Bactrocera oleae, the black scale Saissetia oleae, the moth Prays oleae, the borer Palpita unionalis), and to air-borne (e.g., the peacock spot Spilocaea oleaginea, the knot Pseudomonas syringae) or soil-borne (e.g., verticillium wilt Verticillium dahliae and plant-parasitic nematodes) parasites and pathogens.
2 Plant-parasitic nematodes associated with olive trees
2.1 General considerations
Nematodes are roundworms of the phylum Nematoda, Metazoa having unsegmented, cylindrical bodies with a non-ciliated tough outer cuticle. The group includes free-living forms and disease-causing parasites. Plant-parasitic nematodes (PPN) are soil-borne microscopic worms that mainly feed on plant-root cells using a spear-like structure, called a stylet. They introduce it in cells to inject digestion secretions and suck cell contents [30].
Thereby, these parasites cause significant economic damages to many types of crops as cereals, vegetables, tubers, fruit, and floral crops [31]. Annual losses caused by PPN are estimated from 8.8 to 14.6% of the world crop production (100–157 billion USD/year) [32,33]. In Europe, economic losses are estimated to 10% of the grain production and lead to reduce by 20 to 30% Mediterranean citrus production [34].
Olive trees are hosts of these parasites. But very few information is available about PPN communities associated with olive [27]. The first record was from the USA, where root-knot nematodes Meloidogyne spp. were detected [35]. Since then, some researches on olive PPN were developed in several parts of the world, mainly in Italy [36–38], Chile [39], Jordan [40], Libya [41], Spain [27,42], Portugal [43], Egypt [44–46], Iran [47,48], Turkey [49,50], Argentina [51], and Syria [52].
Most of these studies have focused on PPN description without studying their potential effects on the crop [53]. However, studies focused on taxa that represent a real threat to olive tree, particularly in the Mediterranean Basin, such as root-knot nematodes Meloidogyne [54–57], lesion nematodes Pratylenchus [58], spiral nematodes Helicotylenchus [59,60], reniform nematodes Rotylenchulus [61,62], cyst nematodes Heterodera [63,64], and dagger nematodes Xiphinema [60].
Other studies focused on assessing the impact of these parasites in nurseries, especially in Spain [27] and Iran [48].
2.2 Diversity of plant-parasitic nematodes associated with olive trees
Previous reviews updated knowledge about nematode diversity associated with olive tree [53,65–68].
Moreover, the analysis of all literature sources let to record 153 species (some as still unidentified) belonging to 56 genera related to the PPN orders Aphelenchida (4 genera and 3 species), Dorylaimida (5 genera and 36 species) and Tylenchida (48 genera and 114 species) (Table 1). Most of the species have been detected on other crops [28]. They belong to three trophic groups: plant feeders, fungal feeders (this group usually feeds on fungi but may alternatively feeds on plants, e.g. Aphelenchida nematodes) and root-hair feeders. Considering their adaptive strategies defined by colonizer/persister values (= cp-values) ranking from 1 (colonizers/r strategy) to 5 (persisters/K strategy) [69], most of these species show high reproduction rates and rapid adaptation capacities to environmental changes (cp-3).
Plant-parasitic nematode taxa associated with olive tree.
Order | Family | Species | Country | Reference |
Aphelenchida | Aphelenchidae | Aphelenchus avenae Bastian 1865 | Greece Irana Jordan Spain |
[103]
[48] [40] [42] |
Aphelenchus sp. Bastian 1865 | Iran Italy |
[47]
[36] |
||
Paraphelenchus pseudoparietinus Micoletzky 1921 | Spain | [42] | ||
Aphelenchoididae | Aphelenchoides sp. Fischer 1984 | Egypt Italy Jordan Spain Syria |
[46]
[36] [40] [42] [52] |
|
Aprutides guidettii Scognamiglio 1974 | Spain | [42] | ||
Dorylaimida | Longidoridae | Longidorus africanus Merny 1966 | Egypt | [108] |
L. belloi Andres and Arias 1988 | Portugal | [79] | ||
L. closelongatus Stoyanov 1964 | Greece | [167] | ||
L. cretensis Tzortzakakis et al., 2001 | Greece | [168] | ||
L. elongatus De Man 1876 | Egypt | [46] | ||
L. macrosoma Hooper 1961 | Spain | [42] | ||
L. siddiqii Aboul-Eid 1970 | Jordan | [94] | ||
L. vinearum Bravo and Roca 1995 | Portugal | [79] | ||
Longidorus sp. Micoletzky 1922 | Egypt Italy Spaina Syria |
[60]
[36] [27] [52] |
||
Paralongidorus sp. Siddiqi et al., 1963 | Italy | [110] | ||
Xiphinema aequum [112] | Italy | [112] | ||
X. barense Lamberti et al., 1986 | Italy | [113] | ||
X. californicum Lamberti and Bleve-Zacheo 1979 | USA | [114] | ||
X. diversicaudatum Micoletzky, 1927 | Portugal | [115] | ||
X. elongatum Schuurmans Stekhoven and Teunissen, 1938 | Egypt | [60] | ||
X. index Thorne and Allen 1950 | Greece Italy Jordan |
[169]
[121] [40] |
||
X. ingens Luc and Dalmasso 1964 | Italy Jordan |
[116]
[94] |
||
X. italiae Meyl 1953 | Italy | [78] | ||
X. lusitanicum Sturhan, 1983 | Portugal | [79] | ||
X. macroacanthum Lamberti et al., 1990 | Italy | [117] | ||
X. nuragicum Lamberti et al., 1992 | Spain | [17] | ||
X. pachtaicum Tulaganov 1938 | Italy Jordan Portugal Spain Spaina |
[68]
[94] [79] [17] [27] |
||
X. sahelense Dalmasso, 1969 | Spain | [118] | ||
X. turcicum Luc and Dalmasso, 1964 | Spain | [42] | ||
X. vuittenezi Luc et al., 1964 | Spain | [118] | ||
Xiphinema sp. Cobb, 1913 | Chile Egypt Iran Israel Italy Jordan Spain |
[120]
[46] [47] [170] [36] [171] [172] |
||
Trichodoridae | Paratrichodorus alleni Siddiqi, 1974 | Italy | [142] | |
P. minor Colbran 1956 | Spaina | [27] | ||
P. teres Hooper 1962 | Spaina | [27] | ||
Paratrichodorus sp. Siddiqi 1974 | Jordan | [40] | ||
Trichodorus aequalis Allen 1957 | Spain | [42] | ||
T. andalusicus Decraemer et al., 2012 | Spainb | [140] | ||
T. iliplaensis Decraemer et al., 2012 | Spain | [140] | ||
T. giennensis Decraemer et al., 1993 | Spaina | [27] | ||
T. lusitanicus Siddiqi, 1974 | Portugal | [143] | ||
T. parasilvestris Decraemer et al., 2012 | Spain | [140] | ||
T. primitivus De Man 1880 | Portugal | [143] | ||
T. silvestris Decraemer et al., 2012 | Spainb | [140] | ||
T. taylori Mancine 1980 | Italy | [141] | ||
T. variopapillatus Hooper, 1972 | Italy | [142] | ||
Trichodorus sp. Cobb 1913 | Chile Italy |
[120]
[36] |
||
Tylenchida | Anguinidae | Ditylenchus anchiliposomus Tarjan 1958 | Spain | [42] |
D. dipsaci Kuhn 1857 | Jordan Spain |
[40]
[173] |
||
D. virtudesae Tobar-Jimenez 1964 | Spain | [172] | ||
Ditylenchus sp. Filipjev 1936 | Italy Jordan Spain |
[36]
[171] [42] |
||
Criconematidae | Criconema annuliferum De Man 1921 | Spain | [42] | |
C. princeps Andrássy 1962 | Portugal | [68] | ||
Criconema sp. Hofmänner and Menzel 1914 | Greece | [169] | ||
Criconemella informis Micoletzky 1922 | Spain | [42] | ||
C. sphaerocephala Taylor 1936 | Spain | [42] | ||
C. xenoplax Raski 1952 | Jordan Spain |
[40]
[27] |
||
Criconemella sp. De Grisse and Loof 1965 | Spain | [42] | ||
Criconemoides informis Micoletzky 1922 | Jordan Spaina |
[94]
[27] |
||
Criconemoides sp. Taylor 1936 | Chile Egypt Greece Italy Jordan |
[120]
[60] [103] [36] [171] |
||
Crossonema multisquamatum Kirjanova 1948 | Zimbabwe | [174] | ||
Hemicriconemoides gaddi Loos 1949 | Turkey | [49] | ||
Mesocriconema curvatum Raski 1952 | Spain | [68] | ||
M. onoense Luc 1959 | Brazil | [175] | ||
M. sicula Loof and De Grisse 1989 | Italy | [176] | ||
M. sphaerocephalum Taylor 1936 | Spain | [68] | ||
M. xenoplax Raski 1952 | Spaina | [27] | ||
Neolobocriconema olearum Hashim 1984 | Jordan | [177] | ||
Nothocriconema princeps De Grisse and Loof 1965 | Portugal | [142] | ||
Ogma civellae Steiner 1949 | Zimbabwe | [174] | ||
O. rhomobosquamatum Mehta and Raski 1971 | Spain | [42] | ||
O. seymouri Wu 1965 | Jordan Italy Portugal Spain |
[40]
[148] [146] [68] |
||
Ogma sp. Mehta and Raski 1971 | Italy | [110] | ||
Dolichodoridae | Dolichodorus heterocephalus Cobb 1911 | Italy | [178] | |
Dolichodorus sp. Cobb 1915 | Italy | [110] | ||
Trophurus sp. Loof 1956 | Italy | [36] | ||
Hemicycliophoridae | Hemicycliophora sturhani Loof 1984 | Turkey | [49] | |
Hemicycliophora sp. De Man 1921 | Chile Spaina |
[120]
[27] |
||
Heteroderidae | Heterodera avenae Filipjev 1934 | Spaina | [27] | |
H. mediterranea Vovlas et al., 1981 | Italy Spain |
[63]
[64] |
||
Heterodera sp. Filipjev and Schuurmans Stekhoven 1941 | Italy | [36] | ||
Heteroderinae Filipjev and Schuurmans Stekhoven 1941 | Spain | [42] | ||
Hoplolaimidae | Aorolaimus perscitus Doucet 1980 | Spain | [147] | |
Helicotylenchus digonicus Perry 1959 | Cyprus Greece Jordan Spain Spaina Turkey |
[179]
[103] [180] [17] [27] [49] |
||
H. dihystera Cobb 1893 | Brazil Cyprus Egypt Italy Jordan Spaina Spain Zimbabwe |
[181]
[179] [108] [111] [180] [27] [182] [109] |
||
H. erythrinae Zimmermann 1904 | Italy | [59] | ||
H. minzi Sher 1966 | Jordan | [40] | ||
H. neopaxili Inserra et al., 1979 | Italy | [70] | ||
H. oleae Inserra et al., 1979 | Italy | [70] | ||
H. pseudorobustus Steiner 1914 | Greece Irana Italy Jordan Spaina Spain |
[103]
[48] [68] [94] [27] [27] |
||
H. tunisiensis Siddiqi 1963 | Israel Jordan Portugal Spain Turkey |
[109]
[94] [146] [173] [49] |
||
H. vulgaris Yuen 1964 | Jordan Portugal Spaina Spain Turkey |
[40]
[146] [27] [173] [49] |
||
Helicotylenchus sp. Steiner 1945 | Algeria Chile Egypt Greece Iran Italy Jordan Syria |
[104]
[120] [46] [103] [47] [36] [171] [52] |
||
Hoplolaimus aorolaimoides Siddiqi 1972 | Portugal | [146] | ||
H. galeatus Cobb 1913 | Turkey | [49] | ||
Hoplolaimus sp. Daday 1905 | Egypt Syria |
[60]
[52] |
||
Plesiorotylenchus striaticeps Vovlas et al., 1993 | Turkey | [49] | ||
Rotylenchus buxophilus Golden 1956 | Turkey | [49] | ||
R. cypriensis Antoniou 1980 | Jordan Turkey |
[40]
[49] |
||
R. robustus De Man 1876 | Portugal | [142] | ||
Rotylenchus sp. Filipjev 1936 | Cyprus Greece Italy Spaina |
[179]
[103] [36] [27] |
||
Hoplolaiminae Filipjev 1934 | Spain | [42] | ||
Meloidogynidae | Meloidogyne acrita Chitwood 1949 | China | [89] | |
M. arenaria Neal 1889 | Chile China Spaina |
[39]
[89] [27] |
||
M. baetica Castillo et al. 2003 | Spainb | [90] | ||
M. hapla Chitwood 1949 | Chile Israel Portugal |
[39]
[88] [96] |
||
M. incognita Kofoid and White 1919 | Argentina Brazil Chile China Egypt India Israel Italy Jordan Lebanon Lybia Portugal Spaina |
[68]
[181] [39] [89] [45] [183] [88] [184] [185] [186] [41] [187] [27] |
||
M. javanica Treub 1885 | Argentina Chile China Egypt Greece Irana Israel Italy Jordan Lybia Pakistan Portugal Spaina |
[51]
[39] [89] [60] [103] [48] [87] [184] [94] [41] [68] [68] [27] |
||
M. lusitanica Abrantes and Santos 1991 | Portugal Spaina |
[43]
[27] |
||
Meloidogyne sp. Goeldi 1887 | Chile Cyprus Iran Israel Italy Jordan Portugal Syria USA |
[120]
[179] [47] [87] [59] [171] [188] [52] [35] |
||
Paratylenchidae | Gracilacus peratica Raski 1962 | Italy Portugal Spain |
[36]
[146] [68] |
|
G. teres Raski 1976 | Spain | [147] | ||
Gracilacus sp. Raski 1962 | Italy Spain |
[37]
[42] |
||
Paratylenchus arculatus Luc and de Guiran 1962 | Spaina Turkey |
[27]
[49] |
||
P. baldacii Raski1975 | Spain | [42] | ||
P. ciccaronei Raski 1975 | Spain | [17] | ||
P. microdorus Andrassy 1959 | Spain | [173] | ||
P. nanus Cobb 1923 | Spain | [173] | ||
P. sheri Raski 1973 | Spain | [173] | ||
P. vandenbrandei De Grisse 1962 | Italy | [95] | ||
Paratylenchus sp. Filipjev 1936 | Italy Jordan Syria |
[36]
[171] [52] |
||
Pratylenchidae | Pratylenchoides erzurumensis Yuksel 1977 | Turkey | [49] | |
P. ritteri Sher 1970 | Spaina Iran |
[27]
[190] |
||
Pratylenchoides sp. Winslow 1958 | Jordan | [171] | ||
Pratylenchus coffeae Zimmerman 1898 | Australia Jordan |
[101]
[40] |
||
P. crenatus Loof 1960 | Italy | [190] | ||
P. fallax Seinhorst 1968 | Spaina | [27] | ||
P. mediterraneus Corbett 1983 | Turkey | [49] | ||
P. musicola Cobb 1919 | USA | [102] | ||
P. neglectus Rensch 1924 | Greece Italy Iran Jordan Spain Spaina |
[103]
[95] [189] [40] [173] [27] |
||
P. penetrans Cobb 1917 | Australia Italy Jordan Spain Turkey |
[191]
[95] [40] [42] [68] |
||
P. thornei Sher and Allen 1953 | Irana Jordan Spaina |
[48]
[94] [27] |
||
P. vulnus Allen and Johnson 1951 | Algeria Australia Italy Spaina USA |
[104]
[191] [58] [27] [102] |
||
P. zeae Graham 1951 | Turkey | [49] | ||
Pratylenchus sp. Thorne 1949 | Algeria Chile Egypt Greece Iran Italy Jordan Spain Syria |
[104]
[120] [46] [103] [47] [36] [171] [42] [52] |
||
Radopholus sp. Thorne 1949 | Greece | [103] | ||
Zygotylenchus guevarai Tobar-Jimenez 1963 | Spain Spaina Turkey |
[42]
[27] [49] |
||
Psilenchidae | Psilenchus hilarulus De Man 1921 | Irana | [48] | |
P. iranicus Kheiri 1970 | Jordan Spain |
[40]
[42] |
||
Psilenchus sp. De Man 1921 | Greece Iran |
[169]
[47] |
||
Rotylenchulidae | Rotylenchulus macrodoratus Dasgupta et al., 1968 | Greece Italy |
[133]
[131] |
|
R. macrosoma Dasgupta et al., 1968 | Israel Jordan Spainb Turkey |
[134]
[40] [62] [49] |
||
R. reniformis Linford and Oliveira 1940 | Egypt Greece |
[192]
[103] |
||
Rotylenchulus sp. Linford and Oliveira 1940 | Algeria Syria |
[104]
[52] |
||
Telotylenchidae | Amplimerlinius amplus Siddiqi 1976 | Portugal | [193] | |
A. dubius Steiner 1914 | Turkey | [49] | ||
A. macrurus Goodey 1932 | Jordan | [94] | ||
A. paraglobigerus Castillo et al., 1990 | Spaina | [27] | ||
Amplimerlinius sp. Siddiqi 1976 | Spain | [42] | ||
Bitylenchus goffarti Sturhan 1966 | Turkey | [49] | ||
Merlinius brevidens Allen 1955 | Cyprus Greece Irana Jordan Spain |
[179]
[103] [48] [94] [42] |
||
M. microdorus Geraert 1966 | Jordan Spain |
[40]
[173] |
||
M. nothus Allen 1955 | Jordan | [40] | ||
Quinisulcius acutus Allen 1955 | Turkey | [49] | ||
Paratrophurus loofi Arias 1970 | Spain | [42] | ||
Scutylenchus lenorus Brown 1956 | Turkey | [49] | ||
Telotylenchus sp. Siddiqi 1960 | Greece | [103] | ||
Trophurus sp. | Italy | [36] | ||
Tylenchorhynchus aduncus De Guiran 1967 | Spaina | [27] | ||
T. clarus Allen 1955 | Jordan Spaina |
[94]
[27] |
||
T. claytoni Steiner 1937 | Cyprus Turkey |
[68]
[49] |
||
T. cylindricus Cobb 1913 | Turkey | [49] | ||
T. dubius Buetschli 1873 | Greece Spain Spaina |
[103]
[42] [27] |
||
T. goffarti Sturhan 1966 | Jordan | [94] | ||
T. huesingi Paetzold 1958 | Spaina | [27] | ||
T. mamillatus Tobar-Jimenez 1966 | Spaina | [27] | ||
T. penniseti Gupta and Uma 1980 | Turkey | [49] | ||
T. striatus Allen 1955 | Greece | [103] | ||
T. tenuis Micoletzky 1922 | Jordan | [194] | ||
T. tritici Golden et al., 1987 | Turkey | [49] | ||
Tylenchorhynchus sp. Cobb 1913 | Algeria Chile Egypt Greece Iran Italy Jordan Spaina Syria |
[104]
[120] [45] [103] [47] [36] [171] [27] [52] |
||
Tylenchidae | Aglenchus agricola De Man 1884 | Spain | [42] | |
Basiria duplexa Hagemeyer and Allen 1952 | Spain | [42] | ||
Basiria sp. Siddiqi 1959 | Jordan | [40] | ||
Boleodorus thylactus Thorne 1941 | Irana | [48] | ||
Boleodorus sp. Thorne 1941 | Iran Jordan Spain |
[47]
[40] [42] |
||
Coslenchus cancellatus Cobb 1925 | Spain | [42] | ||
C. costatus De Man 1921 | Greece | [103] | ||
C. lateralis Andrassy 1982 | Spain | [42] | ||
Coslenchus sp. Siddiqi 1978 | Spain | [42] | ||
Discotylenchus discretus Siddiqi 1980 | Jordan | [40] | ||
Filenchus discretus Siddiqi 1979 | Spain | [42] | ||
F. filiformis Buetschli 1873 | Greece | [103] | ||
F. sandneri Wasilewska 1965 | Spain | [17] | ||
Filenchus sp. Andrássy 1954 | Jordan Spain |
[40]
[17] |
||
Irantylenchus sp. Kheiri 1972 | Irana | [48] | ||
Neopsilenchus magnidens Thorne 1949 | Spain | [42] | ||
Tylenchus arcuatus Siddiqi 1963 | Spain | [42] | ||
T. davainei Bastian 1865 | Spain | [17] | ||
Tylenchus sp. Bastian 1865 | Chile Greece Iran Italy Jordan Spain |
[120]
[103] [47] [36] [94] [17] |
||
Tylenchulidae | Trophotylenchulus saltensis Hashim 1983 | Jordan | [40] | |
Tylenchulus semipenetrans Cobb 1913 | Australia Chile Egypt Greece Italy USA |
[101]
[68] [60] [169] [38] [195] |
||
Tylenchulus sp. Cobb 1913 | Chile Italy Syria |
[120]
[110] [52] |
a In nurseries.
b On wild olive.
While many species such as Helicotylenchus digonicus, Meloidogyne javanica, M. incognita, Pratylenchus vulnus are able to parasite a wide range of cultivated and wild plants, others, such as H. oleae, H. neopaxilli, M. baetica, M. lusitanica, Rotylenchulus macrosoma, are specific to olive tree [28].
Considering the species richness encountered in each genus, the genera Xiphinema, Tylenchorhynchus, Pratylenchus, Helicotylenchus, Longidorus, Meloidogyne and Paratylenchus are the most diversified with 16, 13, 12, 10, 9, and 8 species respectively, while 41.4% of the genera are monospecific (Fig. 3).

Species diversity in each plant-parasitic nematode genus.
2.3 Distribution of plant-parasitic nematodes
Plant-parasitic nematodes were encountered in most of the olive-producing countries. But 81% of the genera were scattered in less than 25% of the countries (Fig. 4). Twenty-one genera were detected in only one country. The dominant genera (Criconemoides, Helicotylenchus, Longidorus, Meloidogyne, Pratylenchus, Rotylenchulus, Rotylenchus, Tylenchorhynchus, Tylenchulus, Tylenchus, and Xiphinema) are each present in at least six countries (Fig. 5), especially around the Mediterranean Basin. Root-knot nematodes Meloidogyne spp. are distributed in 19 countries. That could explain the economic importance of this group that can represent a real threat for olive.

Frequency of the plant-parasitic nematode genera detected (% of countries).
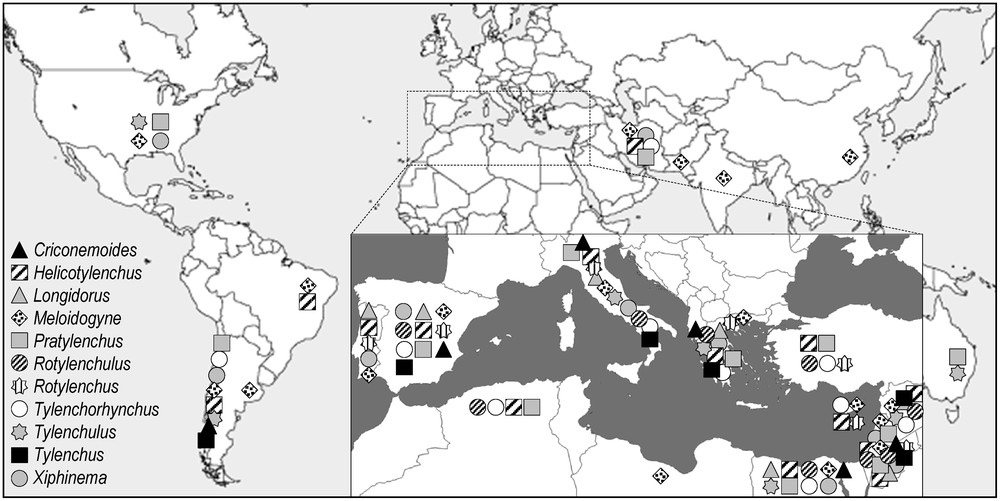
World and Mediterranean distribution of the most frequent plant-parasitic nematode genera associated with olive tree (i.e. present in more than 25% of countries).
Modelling the number of genera recorded in each country (Fig. 6A), the highest diversities were recorded in Spain (38 genera), in Jordan (28), in Italy (24), in Greece (20), and in Turkey (16). Ten to twelve genera were detected in Chile, Egypt, Iran, Portugal, and Syria. Only one to five genera were recorded in the other countries. However, these data can in no way reflect the true diversity of the PPN associated with olive trees in each country. Indeed, modelling the research effort by the number of publications as an indicator (Fig. 6B), the diversity found in each country is close to this indicator. Moreover, considering the Mediterranean Basin only, PPN diversity is totally unknown in France, in Morocco, in Adriatic countries, and in most of the Mediterranean islands.
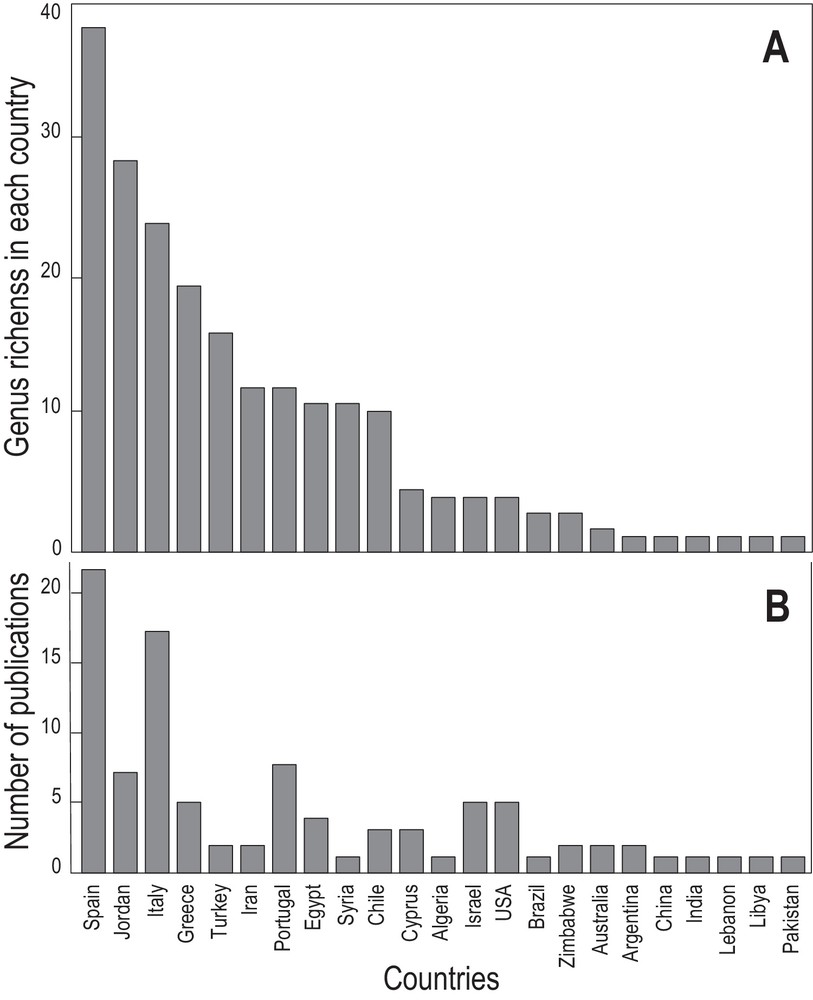
Genus richness of plant-parasitic nematode recorded in each Mediterranean country (A) and associated publications (B).
The most common nematode genera detected in Jordan were [40]: Filenchus, Helicotylenchus, Merlinius, Pratylenchus, and Xiphinema. A more recent study conducted in Iran revealed other frequent genera, such as Aphelenchus, Boleodorus, Meloidogyne, Psilenchus, Tylenchus, and Tylenchorhynchus [47].
The spatial distribution of PPN species in different fields sampled in Spain is homogeneous [42]. However, this author noticed seasonal fluctuations according to local climate. High-level PPN populations were observed in autumn, low levels in summer and intermediate levels in winter and spring. He hypothesized that microclimatic soil variations could modify root tissue plasticity, which consequently could change the aptitude of nematodes to penetrate roots [70].
Nematode occurrence usually differs in nurseries: the most common species detected in southern Spain are M. incognita, M. javanica, M. arenaria, Mesocriconema xenoplax, Pratylenchus penetrans, and P. vulnus [27]. In contrast, the most common nematodes detected in nurseries in northern Iran are [48]: Aphelenchus avenae, M. javanica, Irantylenchus sp. (a new genus reported for the first time as associated with olive), Pratylenchus thornei, Helicotylenchus pseudorobustus, Boleodorus thylactus, Psilenchus hilarulus, and Merlinius brevidens. But in nurseries, the occurrence of PPN is directly related to the origin of the cutting substrates that are often not sterilized, and to the susceptibility of olive cultivars (e.g., P. vulnus is more frequent on cv. Manzanilla than on other cultivars [27]).
2.4 Impacts of plant-parasitic nematodes on olive trees
Host–parasite interactions between PPN and olive trees have been scarcely studied. However, controlled experiments showed that root-knot nematodes Meloidogyne (e.g., M. arenaria, M. incognita, and M. javanica) significantly reduce tree growth [55–57,71] and may be responsible for 5 to 10% crop losses [33], while these damages are often difficult to assign to PPN [28]. Indeed, the symptoms due to PPN parasitism are not clear, because
- • the hardiness of this plant is able to hide them;
- • population levels are usually low in the rhizosphere [68]. In any case, this is commonly observed in traditional olive cropping systems.
Three species of fruit crop infecting nematodes that could damage olive (Longidorus diadecturus, Xiphinema americanum, X. californicum) are absent in Europe [68]. These species have been declared as quarantine pests in Europe (EPPO/OEPP). Heterodera mediterranea and X. italiae are also quarantine pests in Chile.
The nematode parasitism impacts directly the plant growth and yield. Basically, root functions are disturbed by histological alterations of root tissues. Physiological disorders limit water and mineral absorption, leading to major deficits [66]. These phenomena are less prominent on wild olive trees that seems more tolerant to PPN infection [28].
The impact of nematodes is much more significant in nurseries where irrigation conditions favour the development of roots and therefore PPN multiplication [27,28,68]. Humidification of the substrates moderates the temperature, two factors that support the development of nematodes [72]. Moreover, roots of olive seedlings are more susceptible to PPN than older plants [27]. Thus, infestation levels with Meloidogyne arenaria, M. incognita, M. javanica, Mesocriconema xenoplax, Pratylenchus penetrans and P. vulnus are significantly higher in nurseries than in orchards [27,48]. But although the same genera are known for their harmfulness on other fruit trees such as apple and pear, the damaging threshold of PPN is poorly evaluated on olive [55,58,73].
Indirect root damages are also related to nematode infection, whose penetration opens pathways to other soil-borne pathogens (bacteria, fungi) [67,74]. The best example is the association of PPN, such as M. incognita and P. vulnus, with the fungal pathogen Verticillium dahliae (Verticillium wilt). The presence of nematodes enhances the symptoms induced by the fungus [75–77]. Experiments have demonstrated a positive correlation between the number of root galls induced by M. incognita and the extent of root rots and vascular discoloration, and the M. incognita/V. dahliae combination reduced rhyzogenesis [76]. When the three parasites M. incognita/P. vulnus/V. dahliae were associated, the reproduction of each of two nematode species was affected by the presence of the other species and/or by the fungus.
Similarly, damages induced by Heterodera mediterranea could be intensified by the presence of V. dahliae [64]. The M. javanica/Fusarium sp. complex would also be responsible for the “Seca” disease that induces drying of young olive trees in nurseries [51].
Virus transmission by some PPN species is also a major risk to the olive tree. Thus, the olive tree is a natural host to Nepoviruses such as the “Arabis Mosaic Nepovirus” (ArMV), the “Strawberry Latent Ringspot Sadwavirus” (SLRSV) and the “Cherry Leaf Roll Virus” (CLRV) transmitted by Xiphinema diversicaudatum and X. vuittenezi [78,79]. These viruses do not produce symptoms on olive [80], except SLRSV that is responsible for leaf and fruit distortion on the variety Ascolana tenera [81].
Biochemical reactions in olive tree roots are diverse, depending on the nematode species that induce specific physiological mechanisms, as for lesion nematodes as P. vulnus [58,82], for root-knot nematodes as M. javanica [55,56], or for dagger nematodes as Xiphinema index [83]. The secondary metabolism would be involved in plant reactions, which would confer resistance (i.e. lower infestation and reproduction) on some varieties [84].
3 The dominant plant-parasitic nematodes associated with olive trees
3.1 Meloidogyne spp. (root-knot nematodes)
Root-knot nematodes (RKN) are sedentary endoparasites. Ninety-seven species have been described [85]. They have developed reproduction and parasitism strategies that allow them to infect thousands of plant species. Most RKN species induce galls that can invade the whole root system. They are widespread all over the world [66,74,86].
These nematodes have been firstly reported on olive trees in the United States [35]. Thereafter, several Meloidogyne species were detected: M. javanica [87], M. incognita, and M. hapla [88], M. arenaria [89], M. lusitanica [43], and M. baetica [90] recently. These nematodes occur on wild and cultivated olive and also in nurseries (except for M. baetica [27] (Table 1). Among these species, M. baetica was detected on wild olive in southern Spain, and could infect olive orchards. Experiments have shown that this species does not reproduce on tomato (on which it induces small galls without any egg mass) or on legumes. That would confirm its specificity to olive, which led to call it “Mediterranean olive root-knot nematode” [90]. This species was also detected on Pistacia lentiscus and Aristolochia baetica [91].
RKN are very well adapted to a wide range of temperatures, which explains their worldwide distribution (Table 1 and Fig. 7). However, M. incognita and M. javanica are the most widespread species in the world [53,92] especially in the Mediterranean Basin [93].

World and Mediterranean distribution of root-knot nematode species (Meloidogyne spp.) associated with olive tree.
But RKN are at least the less frequent species in olive orchards: they were detected in less than 4% of the samples in Jordan, mainly in irrigated areas and nurseries [40,94], in 2% of the samples in southern Italy (the main production area of the country) and in less than 1% in the other producing areas of the country [95]. That supports other observations made elsewhere in the world [54,55,60,92].
In spite of their scarce distribution, RKN are considered as major pests on olive [53]. Thus, M. javanica is the main cause of “Seca” syndrome in new olive orchards in Argentina [51]. This species is responsible for olive decay in nurseries [40]. RKN reduce plant growth [27,43,55,56]. Pathogenicity tests showed that M. arenaria, M. incognita and M. javanica grow as well on rootstocks [56,57] and on plants cv. Picual and cv. Arbequina, which are widely distributed in the Mediterranean Basin [71]. Controlled experiments showed that six months after inoculation with 5000 or 10,000 M. javanica juveniles per plant, plant growth was significantly reduced: 28% [60] and 45–52%, according to the cultivar [55], respectively. The stem diameter is the most sensitive parameter to infestation [57,71]. Olive trees infected by Meloidogyne spp. show yellowing of the highest branches, and then defoliation [68]. These symptoms are observed everywhere, as well as in China [89], Portugal [96], and Chile [39].
Histological observations of roots infected with M. incognita [97], with M. baetica [90,91], or with M. javanica and M. lusitanica [92] indicate different sizes and different localisations of the galls they induce. M. javanica galls are usually located on the root tip, but some galls are also distributed along roots. Clusters of several galls were also observed and no secondary roots seen near them [92]. M. lusitanica galls are usually distributed as individual galls along roots. M. baetica induces small galls, frequently located along the taproot and very rarely on secondary roots, without the development of egg masses [90].
3.2 Pratylenchus spp. (lesion nematodes)
Lesion nematodes (LN) are migratory endoparasites. They are worldwide distributed, and are able to infect a wide range of plants. They are involved with other soil-borne pathogens (e.g., fungi) in parasitic complexes. LN are the most damaging nematodes with RKN [98–100].
Several Pratylenchus species have been detected on olive (Table 1 and Fig. 5): P. coffeae [101], P. crenatus [95], P. mediterraneus and P. musicola and P. vulnus [102], P. neglectus [103], P. penetrans [95], P. thornei [104] and P. zeae [49]. Some of them (P. neglectus, P. penetrans, P. thornei, P. vulnus and P. fallax) have been also observed in nurseries [27,48].
Among these species, P. vulnus and P. penetrans are considered the most frequent and pathogenic on olive [58,71].
Infection with LN induces large necrotic areas on roots, which may cover all the root system. This may rot the cortical parenchyma [66,102] and reduce both number and size of infected roots [105]. Other symptoms such as leaf chlorosis, internode shortening and defoliation can be observed [106].
Controlled inoculations revealed that most of the LN species do not reproduce on olive, leading one to minimize the impact of these nematodes on this crop [71]. Field observations pointed that LN reproduce primarily in weeds without injuring olive trees [107]. Nevertheless, P. vulnus is the most pathogenic species [58]. It is responsible for tree decline [53,106]. Significant damages attributed to this species have been recorded in several olive orchards in Italy [38,95,106], in the USA [102], and in Algeria [104]. Inoculations with P. vulnus performed on several olive cultivars (Arbequina, Ascolana, Manzanilla, Picual) confirmed its strong pathogenic potential [58,71]. Competitions between RKN and LN have been recorded on olive. Reproduction of P. vulnus populations was reduced with root-knot nematode co-infection [76].
3.3 Helicotylenchus spp. (spiral nematodes)
Spiral nematodes (SN) are migratory ectoparasites. They are also widely distributed in the world.
Several Helicotylenchus species were detected in the olive rhizosphere such as H. digonicus [103], H. erythrinae [59], H. dihystera [108], H. minzi [40], H. neopaxili and H. oleae [70], H. pseudorobustus [103], H. tunisiensis [109], and H. vulgaris [40]. Some species, such as H. digonicus, H. pseudorobustus and H. vulgaris were also detected in nurseries [27,48]. These species have been observed in different olive-producing areas in the world (Table 1 and Fig. 5).
H. digonicus is dominant in many olive orchards [17,42]. For example, it occurs in more than 45% of the orchards in Jordan [40]. Nevertheless, H. oleae is widely distributed in Italy [70], where H. digonicus was not detected [42]. Other studies also demonstrate the dominance of other spiral nematodes, as Rotylenchus spp. in Italy [37,110,111].
Pathogenicity processes caused by SN are still unclear [53]. However, some few studies indicate that H. dihystera, H. erythrinae or H. oleae induces brown lesions on roots and chlorosis of leaf tips, leading to root-mass and plant-growth reduction [59,60,66,70]. Growth delay was observed on plants infected by H. digonicus [93]. A 78% plant-growth reduction was measured on plants inoculated with 1000 H. dihystera six months before [60]. Some species (e.g., H. digonicus and H. oleae) have a semi-endoparasitic behaviour (i.e. introduction of the anterior body part into roots) [40,70]. H. oleae penetrates up to the root epidermis and to the cortical parenchyma. It induces cell wall necroses, feed from place to place, leaving cavities in the cortex.
3.4 Xiphinema spp. (dagger nematodes)
Dagger nematodes (DN) are ectoparasites, frequently found in the olive rhizosphere [66]. Different species have been recorded (Table 1 and Fig. 5): Xiphinema aequum [112], X. barense [113], X. californicum (X. americanum) [114], X. diversicaudatum [115], X. elongatum [60], X. index [40], X. ingens [116], X. italiae [78], X. lusitanicum [79], X. macroacanthum [117], X. nuragicum [17] and X. pachtaicum [94], X. sahelense and X. vuittenezi [118], and X. turcicum [42]. Despite the low population densities, X. pachtaicum is the most common DN species on olive [40,94].
X. diversicaudatum and X. pachtaicum show different distribution areas [115]. X. diversicaudatum could be considered as an Atlantic species, distributed in both cultivated and uncultivated habitats, but is more abundant in uncultivated ones with weak environmental stresses. X. pachtaicum is rather a Mediterranean species, associated also with other fruit trees (apple and peach). This would explain why X. diversicaudatum has been reported on olive in central and northern Spain [115], but not in the South [119].
These nematodes are considered as major pathogens on olive trees in Chile [120]. In the USA, they are responsible for 5 to 10% of the damages, which corresponds to an estimated $ 38.9 million loss [33]. X. pachtaicum could be a real nuisance in olive orchards [42]. DNs affect plant growth initiated by root necrosis [60,121].
A 65% plant-growth reduction was observed on plants inoculated with 500 X. elongatum six months before [60]. However, significant development of X. californicum populations did not affect plant growth [114]. In fact, DNs are usually difficult to rear in controlled conditions because of their susceptibility to moisture and temperature variations [122]. That could explain failures in pathogenicity experiments [53].
3.5 Tylenchulus spp. (citrus nematodes)
Usually known as citrus parasites, T. semipenetrans was reported on olive in California, Chile and Italy [123] (Table 1 and Fig. 5). Studying several life history traits associated with infection and reproduction on specific host plants, six biotypes were identified: four in California [124], one in Florida [125], and one in Italy [126]. This diversity was revised and reduced to four biotypes [127]:
- • the “Poncirus biotype” that reproduces on Poncirus trifoliate and grapevine but not on olive (= California biotype C3);
- • the “Citrus biotype” that reproduces on Citrus spp., grapevine and olive (= California biotype C1)—both previous C2 and C4 California biotypes were included in this biotype group, since they cannot reproduce on P. trifoliate; but they were detected on olives in Italy;
- • the “Mediterranean biotype” is very close to the “Citrus biotype” for its host range except olive—this biotype has been found in all olive-producing Mediterranean countries, in South Africa and possibly in India;
- • the “Grass biotype” was reported in Florida only, where it reproduces only on Andropogon rhizomatus, but this biotype corresponds to the new species T. graminis that only parasites monocots [128].
The limits of these biotypes are rather unclear. C1 and C2 reproduce on grapevine and olive, whereas C3 affects grapevine but not olive, and C4 reproduces only on olive [124]. Furthermore, C1 does not reproduce on P. trifoliate and olive [126], while other studies showed that it is the only biotype able to reproduce on olive [127]. Moreover, T. semipenetrans was found in olive roots in northern Italy where citrus is not cultivated [127]. C1 has not been reported in Spain [129] either in orchards or in nurseries [27].
Histological disturbances induced by these nematodes in olive roots are similar to those observed on citrus [127]. However, life history traits vary from one variety to another. C1 is more aggressive and reproduces faster on cvs. Manzanilla and Ascolana than on other cultivars, and much more than on Citrus sinensis, delaying plant growth [130]. Similarly, C1 populations significantly reduced cv. Frangivento root growth, even no reproduction was observed one year after infection [126].
3.6 Rotylenchulus spp. (reniform nematodes)
Reniform nematodes (RN) are semi-endoparasites that infect herbaceous and woody plants, especially in tropical and subtropical regions. Three RN species were detected on olive (Table 1 and Fig. 5): R. macrodoratus [131], R. macrosoma [62,132] and R. reniformis [103,133]. These species were registered in Algeria [104], Greece [103], Israel [134], and Italy [95,131,135], but their pathogenicity on olive remains poorly documented [53].
Non-mature females penetrate the roots and remain in the epidermis on feeding sites [61,136,137]. Mature female produce a gelatinous matrix, and lays eggs within it [62].
Distribution of R. macrodoratus is restricted to Mediterranean countries, especially to France, Greece, Italy, and Malta. It is the most common RN species in Italy, where it has been detected in about 20% of the samples in the South and 22% somewhere else [38,95].
R. macrosoma is more abundant on wild olive in southern Spain [62], but it has not been detected in nurseries in the same region [27]. It is able to reproduce on common olive cultivars (Picual, Arbequina). Potential damages may occur in irrigated sandy soils for 0.5–1 nematode/cm3 population levels, but infected plants show no symptoms [62].
Feeder cell disturbances are different according to RN species: R. macrodoratus and R. reniformis induce extended mononucleated cells [131], whereas R. macrosoma induces syncytia [62]. High infection with R. reniformis leads to weak tree growth [103].
3.7 Heterodera spp. (cyst nematodes)
Cyst nematodes (CN) are sedentary endoparasites. Females lay eggs inside them which remain as a persistence structure, and/or in a yellow gelatinous matrix. When they die, their cuticle hardens, forming a cyst that may persist in the soil for several years in the absence of host plant [66], and hatch when soil conditions are favourable. CN are widespread and economically important nematodes. Very few species infect woody plants [138]. They were not recorded as olive parasites until H. mediterranea was described on cultivated olive (cv Manzanilla) in Spain [64]. More recently, H. avenae was detected in olive nurseries on grasses associated with substrates [27].
H. mediterranea differs from other CN species by its semi-endoparasitic behaviour which would be explained by the woody structure of olive roots [64]. Identical observations have been reported on pistachio tree [63].
Infected olive trees do not exhibit any symptoms on leaves, even in heavily infested soils. However, necrosis and root distortion were observed near root penetration sites [64], suggesting that H. mediterranea could contribute to plant decline in young orchards and nurseries [64,66,139], where high densities occur [66].
3.8 Other plant-parasitic nematodes
Trichodoridae nematodes are also frequently detected on olive trees. They are polyphagous root ectoparasites and are distributed worldwide. They cause damage to a wide range of crops and natural vegetation by direct feeding on roots, causing stunted roots. Some species (13%) are known as Tobraviruses vectors, resulting in important yield losses [140]. Three species of Paratrichodorus and 10 species of Trichodorus were recorded on olive trees, especially in Italy [141,142], Portugal [143], and Spain [27,42,140] (Table 1).
Nematodes belonging to the Telotylenchidae family are not major crop parasites, although they are mostly associated with several crops. The genus Tylenchorhynchus seems to be the most diverse, with 13 species recorded in different olive-producing areas, mainly in the Mediterranean Basin (Table 1 and Fig. 5).
Gracilacus peratica (family Paratylenchidae) is an ectoparasite detected on olives in Italy [36,95,144,145], Spain [42], and Portugal [146] (Table 1). Juveniles, young females and males were detected only in soil, while mature females were observed in roots. Cell walls become thick and lignified [145]. Pathogenicity processes on olive are not known [53]. G. teres was also detected on olive in Spain [147].
Ogma spp. nematodes are sedentary ectoparasites. O. rhombosquamatum has been reported in different orchards in Spain [42], Italy [38,148], Jordan [40], and Portugal [146] (Table 1). Nematode infection induces cortical cell wall thickening and cortex and epidermis necrosis.
4 Management of plant-parasitic nematodes on olive
4.1 Exclusion of PPN infection
Given that PPN are parasites living in the soil and/or in roots, olive tree protection relies first on the use of healthy plant material transplanted in a soil free of these parasites. That is why the first step in avoiding PPN on olive starts in nurseries where the best conditions for PPN multiplication are met, and from where they could be widely disseminated in orchards [53]. In that way, most infestations by RKN and LN nematodes in olive orchards originate from unsanitized plant material produced in uncertified nurseries [149]. Chemical disinfection of substrates is a suitable approach for production of nematode-free nursery stock [68]. Although laws have been established by the European Union for healthy olive seedlings certification [150], their application is unfortunately still limited [68]. Cultural practices such as fertilization, weed control with herbicides, could lead to increased populations of NPP [17]. On the other hand, intensive production systems (high-yield varieties, high-density plantations, permanent grassing, irrigation, fertilization, etc.) induce environments suitable for the development of soil-borne diseases caused directly or indirectly (e.g., verticillium wilt) by PPN [68]. These cropping systems could lead to PPN communities dominated by the most damaging species to olive tree.
4.2 Plant resistance
The use of resistant cultivars is an effective alternative to chemical control of PPN [151], but their productivity in olive fruits and oil can be a limiting factor to use them [53]. Some cultivars are resistant or tolerant to some species of Meloidogyne, Pratylenchus, and Tylenchulus.
Olive varieties can be distinguished as susceptible (nematode reproduction and plant disturbance), tolerant (nematode reproduction without plant disturbance) and resistant (no nematode reproduction) to RKN. Cvs. Manzanillo and Ascolano are very susceptible to M. javanica [54]. But they are resistant to M. arenaria and M. hapla [55]. In nurseries, Arbequina, Cornicabra, Hojiblanca, Manzanilla and Picual are the most susceptible cultivars to M. arenaria, M. incognita, and M. javanica [27].
Olive cultivars show also various reactions towards P. vulnus. This species does not reproduce on cv. Verdalion [82] while cvs. Ascolana and Manzanilla are very susceptible [55]. However, cv. Ascolana is more tolerant than cv. Manzanilla [53]. The host–parasite interaction would also depend on the diversity of both P. vulnus geographical strains and host plants on which they were detected [152].
Several olive cultivars are susceptible to X. index except cvs. FS17 and DA12I that exhibited resistance [121].
Comparing CN reproduction traits, such as soil (juveniles and cysts) and root (juveniles) infestation levels and reproduction rates on different olive cultivars, cv. Arbequina appeared as the most susceptible cultivar to H. mediterranea [68].
These resistant varieties can be also used as stocks for susceptible cultivars that have yield interests.
In that way, resistance screenings were developed, and in vitro tests have proved to be valid alternatives to traditional resistance screenings trials [151].
4.3 Soil solarisation
This method was successfully used to control soil-borne parasites: fungi [153,154], bacteria [155], and PPN [156]. It can be an efficient method especially in the Mediterranean Basin, where climatic conditions are optimal with high summer temperatures. In nurseries, soil solarisation can therefore be used for controlling RKN in substrates [157], affecting 95% of M. incognita eggs up to a 40-cm depth after a 10-day application. However, the gelatinous egg mass matrix protects them against several environmental stresses. Then, Meloidogyne spp. is sometimes heat-tolerant and difficult to control by soil solarisation [158]. This method may also be useful to control other PPN, e.g., P. vulnus and P. penetrans, and other soil-borne pathogens as Verticillium dahliae [157].
4.4 Biological control
4.4.1 Organic residues
The addition of forest residues provides organic matter that could contribute to PPN suppression, by increasing soil microflora [159]. Composted dry cork, especially in nurseries, may be effective for M. incognita suppression, due to the toxicity of released products (e.g., ammonia, phenolic compounds) [160].
4.4.2 Biofumigation
Some plants (e.g., Brassica oleracea, B. nigera, B. juncea, Raphanus sativum, Crambe abyssinica, Sorghum spp.) are able to release volatile biocides after ploughing in soil that could have toxic effects on PPN. Such soil biofumigations are ecological alternatives to chemical fumigation against PPN and other soil-borne pathogens [161]. Trials to assess the effect of Sorghum sudanense on the suppression of M. incognita showed a significant reduction in final nematode populations 30 to 60 days after treatment [68]. Brassicaceae species showed suppressive effects on M. incognita parasitizing tomato plants by reducing root galls and nematode reproduction [162]. The association of these plants with olive trees could be a way to reduce PPN populations.
4.4.3 Microbial control
Soil inoculation with mycorrhizal fungi can be a useful practice to reduce RKN populations. An early inoculation of olive stocks cvs. Arbequina and Picual with Glomus intraradices, G. mosseae and G. viscosum enhanced plant-growth and controlled M. incognita race 1 and M. javanica populations by inhibiting nematode reproduction and reducing root galling [163]. Other microbial antagonists to PPN (such as nematophagous fungi and Pasteuria penetrans) can be also used for nematode suppression.
5 Prospects: better knowledge on PPN biodiversity for better management
This review clearly shows that the diversity of the PPN communities strongly differ across countries. Considering olive protection, PPN control strategies appear to be developed in each Mediterranean producing country according to its own ecological and agronomical constraints, and the PPN diversity encountered.
As seen above, most of the researches emphasized studies targeting some PPN species. Because resistance and biocontrol are specific to emblematic species and because PPN are everywhere found in communities (i.e. species mixtures), these control strategies may induce changes in nematode communities, but do not necessarily decrease their overall pathogenicity. Consequently, such biological strategies may be unsustainable from an ecological point of view [164], and they must focus on community rearrangements instead of reducing the most pathogenic species.
Considering olive tree history and diversity of associated PPN around the Mediterranean Basin, local PPN communities could be originating from historical mixtures set up with native (before olive introduction) and invasive (with rootstocks from oleasters) communities. Olive domestication in the past, and breeding and cropping now could explain the present distribution of PPN. Consequently, management strategies must take into account the spatial distribution of the PPN diversity all around the Mediterranean Basin (regional scale) and in each olive-producing country (local scale). A special focus on PPN communities associated with wild olive would give information about trade-offs between PPN communities and olive trees in non-disturbed situations. Moreover, investigation about co-adaptation between PPN and olive tree (wild and varieties) would provide new insights into assembling mechanisms between PPN and olive trees. In this way, a large survey with high sampling efforts is initiated [165] in order to evaluate as precisely as possible the diversity of PPN communities parasitizing wild and cultivated olive, and to target the historical (evolution) and environmental (cropping systems, olive varieties, soil factors) filters involved in community patterns. As a confirmation, interesting effects of olive genotypes on PPN community patterns were observed [17]. Such observations could lead to the development of new management strategies in a diversity-conservation approach.
Acknowledgements
This work was part of the PESTOLIVE project: Contribution of olive history for the management of soil-borne parasites in the Mediterranean Basin (KBBE 219262), funded by the ARIMNet action (www.arimnet.net) [166] supported by EU and non-EU Mediterranean countries. The authors thank Dr. G. Besnard (CNRS, Université Paul-Sabatier, Toulouse, France) for his critical reading of this manuscript.