1 Introduction
Several studies have shown that schizophrenia stems from neurodevelopmental abnormalities that occur during the process of neurogenesis, neuronal migration, cell differentiation, synaptogenesis and myelination [1–5]. The ventral hippocampus, which sends prominent glutamatergic projections to the prefrontal cortex and thalamus, sites involved in the structural and functional alterations seen in schizophrenia [6–9], is a key component in the pathophysiology of this disease. In order to understand the underlying pathophysiology and pharmacological aspects of schizophrenia, various animal models have been developed. An animal model based on the neonatal lesion of the hippocampus shows a number of behavioral abnormalities related to schizophrenia such as deficit in latent inhibition [10,11], impaired social behavior [12] and hyperlocomotion in an open-field test [13]. These behavioral changes are consistent with enhanced activity of the nucleus accumbens/striatal dopaminergic system (DA) and prefrontal cortical dysfunction.
Cognitive impairment is a central manifestation of the schizophrenic illness [14]. Adult patients with schizophrenia suffer from abnormalities in attention and information processing. One type of behavioral process that has been examined in this context is latent inhibition (LI), which refers to delayed conditioning to a stimulus that has repeatedly been presented without reinforcement [15–17]. Disruption of LI, which corresponds to the failure of schizophrenics to ignore irrelevant stimuli, has received increasing attention as a viable animal model [18–23]. At present, it is not clearly understood whether the stage, the duration of illness, or the treatment by neuroleptics is responsible for these discrepancies. LI is similar in humans and animals and can be viewed as reflecting analogous processes across species [24].
The pharmacology of LI in nonhuman subjects has been investigated with aversively motivated LI procedures such as conditioned emotional response, conditioned avoidance response, conditioned taste aversion, conditioned eyeblink, and conditioned freezing (for a review, see [25]). It has been suggested that the dopaminergic system is involved in the expression of LI (difficulty of learning an association involving a pre-exposure (PE) stimulus) rather than in the acquisition of LI (the cognitive processes by which the stimulus loses its associative capacity) [26]. Some experiments have demonstrated that haloperidol could facilitate LI if it is injected before both the PE and the conditioned phase [26,27]. Thus, the timing of drug administration is a critical factor, which still needs to be clarified. Several studies have been done on the timing of drug administration by comparing the effects of dopamine manipulations during the PE phase, the conditioning phase, or both [27,28]. These studies agree that altered LI could be observed only when DA neurotransmission is manipulated during the conditioning phase [28].
The present study has two major objectives: (1) to evaluate the long-term effects of the nVH lesion by lidocaine injection in rats at postnatal day 7 and validate if this models aspects of schizophrenia, and (2) to investigate the effect of haloperidol injection at the pre-exposure phase of LI to assess dopaminergic involvement in LI and whether dopamine is a contributing factor in the expression (learning) or in the acquisition of LI (the cognitive process).
2 Material and methods
2.1 Animals
The experiments involved 20 Sprague-Dawley rats, maintained in the central animal care facility under constant temperature conditions (20 ± 2 °C), 12 h/12 h light/dark cycle and food and water available ad libitum. All procedures were conducted in conformity with approved institutional protocols and with the provisions for animal care and use prescribed in the Scientific Procedures on Living Animals ACT 1986 (European Council directive: 86/609 EEC). All efforts were made to minimize animal suffering.
2.2 Surgery
At postnatal day 7 (PND7), pups were randomly separated into two groups: (1) a control group given normal saline injection (0.3 μL) and (2) a lesioned group given 1% lidocaine (Laprophan®; 0.4 μg/3 μL) injection in the ventral hippocampus. Hypothermic anesthesia was induced in the pups by placing them on ice for about 6–7 min and then secured on a Styrofoam platform mounted on a stereotaxic frame (David Kopf Instruments). An incision was made in the skin overlying the skull and 0.3 μL of Lidocaine or vehicle (0.9% NaCl) was injected bilaterally into the ventral hippocampus (AP, 2.8 mm; ML, 3.5 mm; and VD, 5.0 mm relative to bregma as defined by Lipska et al. [3]). The micosyringe was withdrawn 3 min after injection and the skin incision closed. The pups were warmed and returned to their mothers. After the weaning, the animals were housed in new cages by group and gender. Behavioral testing started on PND 56.
2.3 Validation of nVH lesion model for schizophrenia like behavior
Prior to the LI test, animals were screened for schizophrenia-related behavior and hippocampal lesioning. All behavioral testing was performed from 9 am to 12 pm and video recording done using a video camera (JVC). The animals were gently handled for 2 min/day and individually familiarized with the testing room and the test arenas for 5 min/day one week prior to testing.
After each test, debris (bedding, faeces, etc.) were removed from the test apparatus, and the interior surfaces cleaned thoroughly with 70% ethanol, then water, and wiped dry. An interval of 5 min was used between tests to ensure complete drying and dissipation of any residual odor of alcohol.
Only rats showing behavioral changes related to schizophrenic-like criteria and specific lesions to the ventral hippocampus were included in this study. Behavioral assessment (described below) was done using the open field test (to assess locomotor activity and emotional reactivity), the social interactions test, the marbles burying test (to assess anxiety and to verify the hippocampal lesion) and the conditioned avoidance response (to assess latent inhibition alteration). In rats that showed the behavioral phenotype related to schizophrenia-like symptoms, the effect haloperidol injection on LI test was investigated using the conditioned taste aversion paradigm.
2.3.1 Open field test in novel environment (PND56)
This test has been regularly used to assess locomotor activity and emotional reactivity in rodents (for a review, see Wilson et al., [29]). During two sessions of 3 min each, locomotor activity of 16 animals from the two groups (lesioned and control) was examined. The apparatus consisted of an open box of 100 × 100 × 40 cm, with the floor divided into a grid of 5 × 5 squares. The total area was 200 cm2. The apparatus was illuminated with a 70–W bulb from a height of 40 cm.
2.3.2 Social interactions test (PND70)
Twenty-four hours before the test, control (n = 8) and lesioned (n = 8) animals were housed individually in new cages with food and water available ad libitum. The cages were maintained in close proximity to facilitate auditory and olfactory contact. The social interaction test was performed in an open field apparatus (100 × 100 × 40 cm), with lighting conditions similar to the animal facility conditions. Two animals from the same group (lesioned or control) were introduced into the apparatus and social behavior was measured by counting of the total number and the total duration of contacts between the animals in a test session of 10 min.
2.3.3 Marble burying (PND 70)
This test was used to assess the level of anxiety and for behavioral verification of nVH lesioning [30–32]. The cage was filled approximately to a depth of 5 cm with wood chip bedding, lightly tamped down to make a flat even surface. The bedding substrate can be reused if it was flattened and tamped down again between trials since the re-use of bedding does not affect the burying/digging performance of subsequent rats [33]. Twenty-one glass marbles (orange and white) were placed in a regular pattern on the surface, evenly spaced out 4 cm apart. The test consisted in placing one animal in each cage for 30 min and counting the number of marbles buried (to 2/3 their depth) with bedding.
2.4 Latent inhibition
2.4.1 Conditioned avoidance response (PND 75)
This test was conducted according to the protocol described by Greksh et al. (1999) [11] to assess whether nVH lesioning caused a disruption of latent inhibition (LI). Haloperidol effect on LI was not evaluated using this test since several studies have showed that DA manipulations during the pre-exposure in a conditioned avoidance response test are without effect on LI (for a review, see [25]).
The shuttle box apparatus (BIOSEB, LE 916) was divided into two compartments of the same dimensions (25 × 29 × 38 cm) separated by a 5-cm hurdle. The conditioned stimulus (CS) was a sound produced by a buzzer and the unconditioned stimulus (US) was an electric foot shock of 0.2–0.4 mA, delivered through stainless steel rods covering the floor. The acquisition and retention of a conditioned reaction in a two way shuttle box were tested in control and lesioned animals, with and without pre-exposure to the conditioned stimulus. The groups consisted of (1) control – pre-exposed, (2) control – not pre-exposed, (3) lesioned – pre-exposed, and (4) lesioned – not pre-exposed.
This test consists of three sessions. (i) In the pre-exposure session, the animals perceive a sound (conditioned stimulus) delivered 60 times at intervals of 30, 60, and 90 s. (ii) The training session consists of 80 trials started immediately after pre-exposure. After application of the conditioned stimulus, the animals have to move to the alternate compartment of the shuttle box to avoid the unconditioned stimulus (shock). The conditioned stimulus–unconditioned stimulus interval was 1 s. The stimuli are switched off when the rat has moved to the goal compartment. Each trial is limited to 30s. (iii) The retention session is performed 24 h after the training session, in order to evaluate learning performance and memory. This relearning test was carried out in the same manner as the training session, but without pre-exposure to the sound.
2.4.2 Conditioned taste aversion (CTA)
Once the schizophrenia phenotype of this animal model was validated, the effect of single administration of haloperidol during the pre-exposure phase on LI using the conditioned taste aversion paradigm was assessed. The CTA test was performed according to the protocol described in Bethus et al. [56] to evaluate the effect of intraperitoneal (IP) injection of 0.1 mg/kg of haloperidol (Haldol® 2 mg/mL, Jansen-Cilag) prior to the pre-exposure session. For the CTA conditioning, the aversion was induced by a single IP injection of 127 mg/kg of lithium chloride (LiCl) (0.5 M, Aeros organics). The conditioned stimulus in CTA was a 5% sucrose solution, which was prepared as needed and stored at 4 °C.
2.4.3 Habituation and base line
The day before starting the habituation period, rats were water deprived, receiving water for only 30 min/24 hr in their home cage. This phase lasted 6 days.
2.4.4 Pre-exposure phase
This phase consisted of 3 sessions of pre-exposure (one session per day) during which rats had access either to sucrose 5% or to water for 30 min. Forty-five minutes before starting each session, the animals were injected IP with either haloperidol or vehicle. Throughout this session, lesioned and control animals were randomized into 4 groups according to their exposure and treatment status: (1) Group 1 (PE-HAL): control animals pre-exposed to sucrose 5% and given an IP injection of haloperidol before each pre-exposure session (n = 5), (2) group 2 (PE-VEH): control animals pre-exposed to sucrose 5% and given normal saline injection before each pre-exposure session (n = 5), (3) group 3 (NPE-HAL): control animals not pre-exposed to sucrose 5%; access to water only during 30 min, and injected with haloperidol before every pre-exposure session (n = 5), (4) group 4 (NPE-VEH): control animals not pre-exposed to sucrose 5%, access to water only during 30 min and then injected with normal saline before every pre-exposure session (n = 5), group 5 (PEL-HAL): lesioned animals pre-exposed to sucrose 5% and received an IP injection of haloperidol before each pre-exposure session (n = 5), (6) group 6 (PEL-VEH): lesioned animals pre-exposed to sucrose 5% and given a normal saline injection before each pre-exposure session (n = 5), (7). group 7 (NPEL-HAL): lesioned animals not pre-exposed to sucrose 5%; access to water only during 30 min, and then injected with haloperidol before every pre-exposure session (n = 5), and (8) group 8 (NPEL-VEH): lesioned animals not pre-exposed to sucrose 5%, access to water only during 30 min and then injected with normal saline before every pre-exposure session (n = 5).
2.4.5 Conditioning phase
Twenty-four hours after the pre-exposure session, animals of different groups had access only to sucrose 5% for 30 min, followed by IP injection of LiCl. The rat must learn to associate the gastrointestinal discomfort induced by the injection of LiCl with the consumption of sucrose.
2.4.6 Test day
After one day of recovery between the conditioning phase and the test day with only tap water available for 30 min in the usual drinking bottles, the animal had to choose for 30 minutes between a drinking bottle containing water and another containing 5% sucrose. This test was to assess the strength of the association between the CS and the US. At the end of each session, the volume of water and/or sucrose consumed was measured to evaluate the effectiveness of the treatments within the different groups.
2.5 Histological procedure
Nissl staining technique was used to verify the lesion site. After completion of all behavioral experiments, the animals were anesthetized with pentobarbital (40 mg/kg) and transcardially perfused with saline and 4% paraformaldehyde solutions. Brains were carefully removed, and stored in 4% paraformaldehyde solution for 24 h, then cryoprotected in 30% sucrose for at least 48 h before sectioning with the cryomicrotome (Leica Microsystems, Germany) at 40 μm. Sections were mounted on gelatine-coated slides and stained with Cresyl violet and examined under a photonic microscope (Leitz). Paxinos and Watson [34] atlas was used for neuroanatomical orientation. The lesion was considered successful if the ventral portion of the hippocampus was bilaterally was lesioned without affecting the dorsal part.
2.6 Statistical analysis
Statistical analysis was performed using SigmaPlot 11.0 software. Inter-group comparison was performed using one-way analysis of variance (ANOVA). For the open field, results were evaluated using the repeated measurement one-way ANOVA test (lesion as factor), and for the CAR test, two-way ANOVA test was performed with the lesion and the pre-exposure as factors. For the CTA paradigm, the data was evaluated by three-way ANOVA test, with lesion, pre-exposure and drug treatment as factors. All these tests were followed by post hoc testing with the Holm–Sidak method for pairwise multiple comparisons. The significance threshold was set at P < 0.05.
3 Result
3.1 Lesion site verification
Histological examination of the Nissl rat brains sections, using the atlas of Paxinos and Watson [34] to verify lesions site, showed that all lesioned rats had extensive lesions within the ventral hippocampus, while most of the dorsal part was spared (Fig. 1). The ventral hippocampus region was consistently shrunk and the lesion site was almost devoid of neurons. Animals with an incomplete bilateral destruction of the ventral hippocampus, a unilaterally destroyed ventral hippocampus, or lesions in the dorsal part of the hippocampus were excluded from further analysis of the behavioral data. In control animals, the hippocampus is morphologically intact.
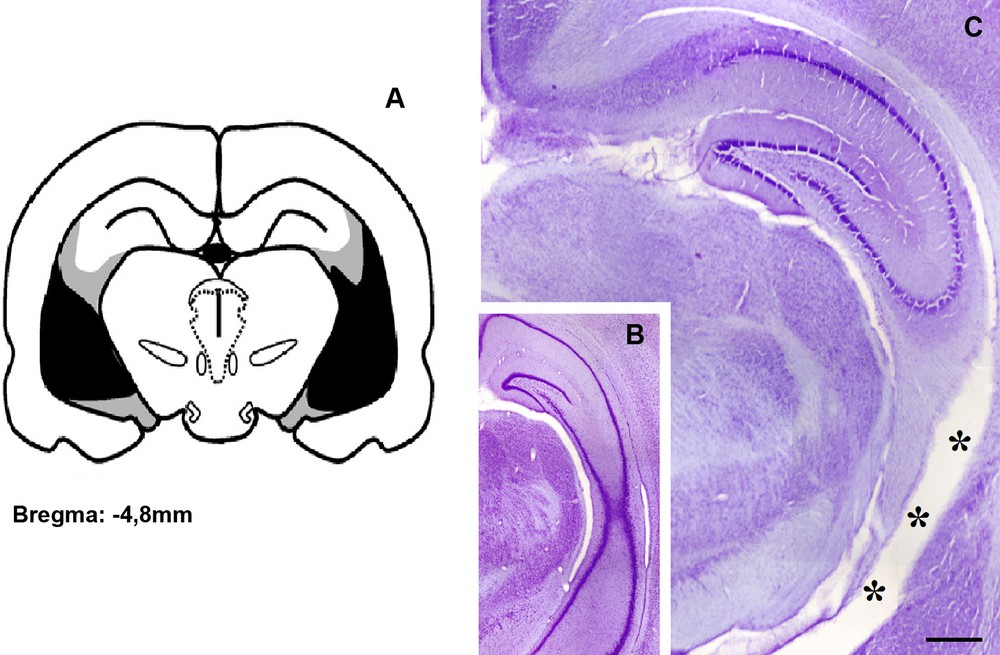
(Colour online.) Histological evaluation of the ventral hippocampal lesion induced by neonatal injection of lidocaine. (A) Drawing showing the extension of the ventral hippocampal damage observed in adulthood. Gray and dark areas indicate maximal and minimal extents of damage, respectively. (B) Coronal Nissl-stained sections at approximately –4.8 mm from the bregma showing the ventral hippocampus of a control rat, and (C) a typical neonatal ventral hippocampal lesion, characterized by thinning of the structure likely by cell loss (stars) and enlarged ventricles, whereas the dorsal part of the brain structure remains morphologically intact. Bar = 100 μm.
3.2 Open field test
The results showed that the distance travelled was affected by nVH lesion. It was increased in lesioned animals compared to the controls (Fig. 2). The quantitative data analysis showed that during the first trial, locomotor activity was significantly higher (90 ± 3.32 cm) compared to the second trial (6.17 ± 2.06 cm) in control group (F (2,17) = 163.10; P < 0.001), as well as in lesioned group (108.17 ± 10.35 cm vs. 62.83 ± 3.16 cm) (F (1,11) = 11.31; P < 0.05).
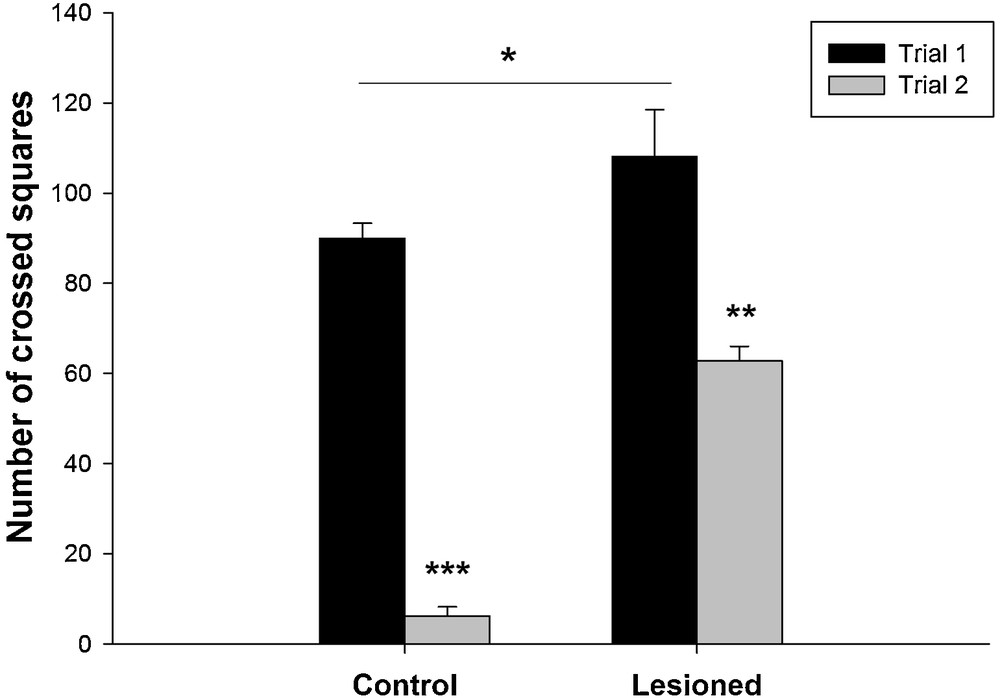
Changes in locomotor activity in control rats and after a neonatal bilateral administration of lidocaine into the ventral hippocampus during the two trials of the Open Field Test. Data presented as means ± SEM (***: P < 0.001; **: P < 0.01; *: P < 0.05).
The post hoc analysis showed that in the first session, lesioned animals had increased locomotor activity (108.17 ± 10.35 cm) compared to the control (90 ± 3.32 cm) (t = 3.36; P < 0.05). Also during the second session the distance travelled was significantly higher in lesioned rats (62.83 ± 3.16 cm) compared to the control (6.17 ± 2.06) (t = 17.7; P < 0.05) (Fig. 2).
3.3 Social interactions test
For social interactions, two parameters were measured: the total number of contacts between two animals from the same group, and the total duration of active social interaction. With regards to the total number of contacts, the behavioral analysis showed that control animals carried out the majority of contacts within the first 6 minutes, whereas lesioned animals spent the majority of their time exploring the apparatus and started interacting by the end of the trial. The analysis of the number of contacts made by each group showed controls (150 ± 2.18) interacted more than lesioned rats (39.38 ± 1.80) (F (1,13) = 1572; P < 0.001) (Fig. 3A). The active social interaction duration was less in lesioned animals (192.29 ± 6.55s) compared to the controls (435.5 ± 17.31 s) (F (1,13) = 178,04; P < 0.001) (Fig. 3B).
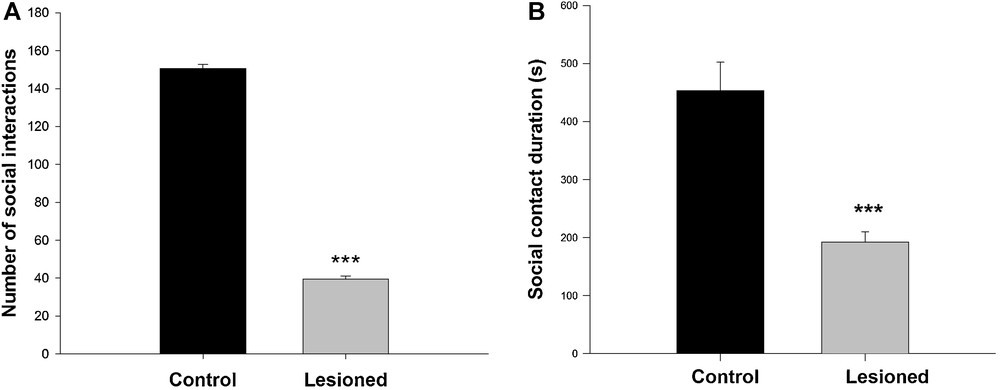
Social interaction responses recorded during a 10-min direct interaction between a rat and another one belonging to the same group, in controls and after neonatal administration of lidocaine into the ventral hippocampus. A. Number of contacts; B. social contact duration. Data are expressed as means ± SEM (***P < 0.001).
3.4 The marble burying test
In this test, the control group was more active and explored more of the cage compared to the lesioned group. After exploration, animals began the burying behavior. The control group buried 75% of marbles, which was significantly greater than those buried by the lesioned group (34.35%; H = 11.725 with 1dF; P < 0.001) (Fig. 4).
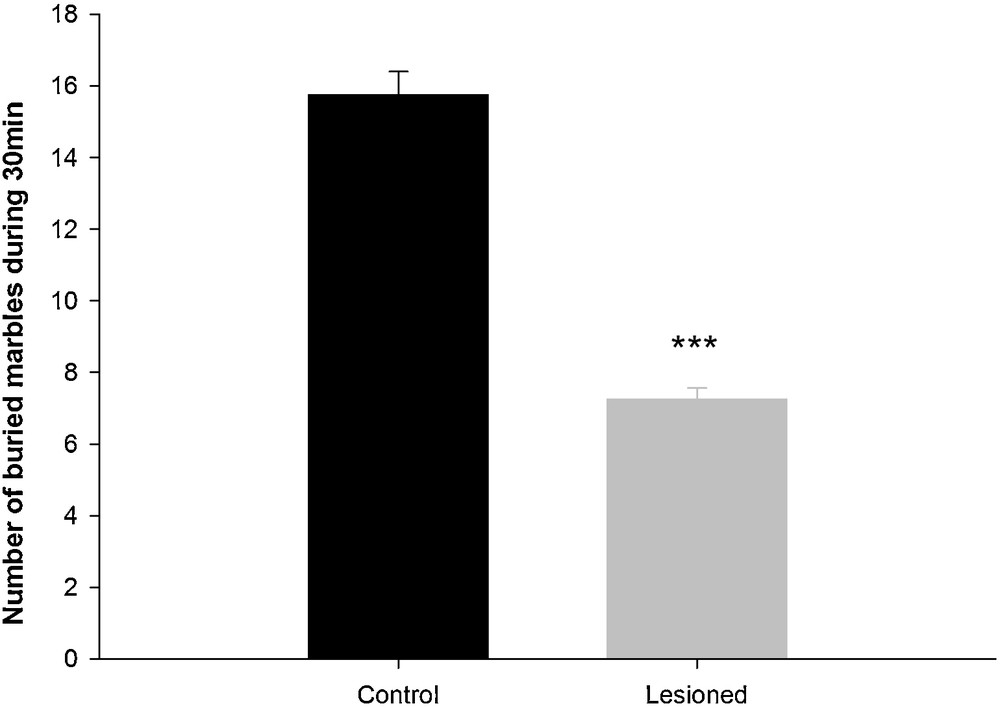
Comparison of the numbers of buried marbles in the marble burying test during a 30-min session between control and lesioned animals after a neonatal bilateral administration of lidocaine into the ventral hippocampus. Data presented as means ± SEM (***: P < 0.001).
3.5 Assessment of latent inhibition in conditioned avoidance response test
During the habituation phase to the new environment (10 min), control animals spent more time grooming and rarely moved to the other compartment (1.9 ± 0.28 times). In contrast, the lesioned group visited and explored regularly the two compartments with an average of 13.1 ± 0.43 times. During this habituation phase, increased movement to the center and edges of the compartment was only observed in lesioned rats.
During the conditioning phase, the pre-exposed animals showed a significant latent inhibition compared to the 3 other groups.
Statistical analysis between all lesioned and all control animals showed that the lesioned animals showed increased number of conditioned reactions (F (2,19) = 11.22; P < 0.001). When comparing between the different pre-exposed and non-pre-exposed groups, the results showed that the pre-exposure to CS also affected the number of conditioned reactions; (F (2.19) = 4.137; P < 0.05).
Post hoc analysis showed that the PEL group learned more quickly the association compared to the PEC group ((32 ± 1.37 vs 11.4 ± 0.6) (t = 2.34; P < 0.05)) and the NPEL made more conditioned reactions compared to the NPEC ((31 ± 0.95 vs. 27 ± 2.45) (t = 0.37; P < 0.05)). Thus, a significant interaction between the lesion and pre-exposure status was found (F (2,19) = 3.93, P < 0.05) (Fig. 5A).
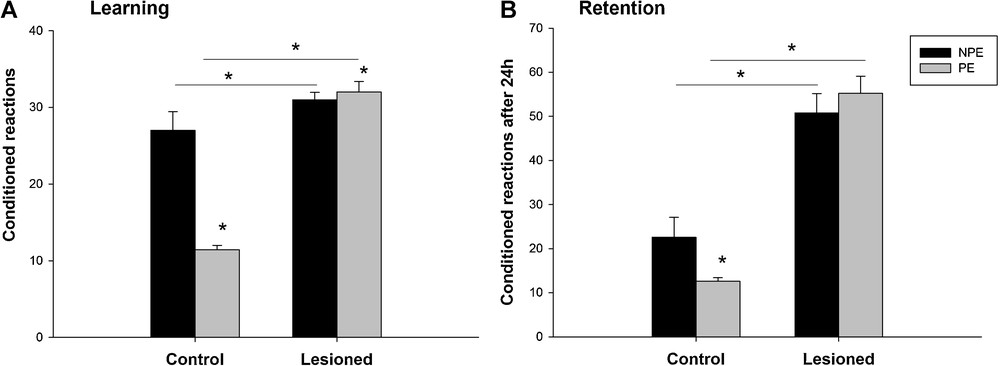
Learning (A) and retention (B) in an active avoidance test (Shuttle box) with and without pre-exposure to the conditioned stimuli. Results obtained from 80 trials in control animals and after a neonatal bilateral administration of lidocaine into the ventral hippocampus. PE: pre-exposed group; NPE: non-pre-exposed group. Data presented as means ± SEM (*P < 0.05).
Statistical analysis of the retention session showed that only in the lesioned animals the number of conditioned associations was affected (F (2.19) = 40.75; P < 0.001) and that the pre-exposure status had no effects (F (2.19) = 1.81; P = 0.19) (Fig. 5B).
3.6 The effect of haloperidol injection on LI in conditioned taste aversion test
For this test, two parameters were analyzed: sucrose consumption and total liquid consumption. This allows us to ascertain whether the sucrose consumption changes were compensated by water consumption or were due to a general decrease in the total liquid consumption.
When animals had only access to water before the conditioning phase (phase of habituation and pre-exposure for the two groups: NPEL-VEH and NPEL-HAL), the total consumption of liquid did not change from one group to another. During the habituation phase, the NPEL-HAL group consumed 5 ± 0.07 mL, which was not statistically different to the volume consumed by the NPEL-VEH (5.83 ± 0.48 mL; H = 3.938 with 1 degree of freedom; P = 0.056; Fig. 6). The same observation was made for the consumption by the PEL-HAL group (5.60 ± 0.56 mL) in comparison to the PEL-VEH group (5.20 ± 0.57 mL (F(1.8) = 1.050, P = 0.335). During the pre-exposure session (P1, P2 and P3), the consumption increased compared to the other days when pre-exposed animals had only access to water (PEL-HAL: 7.47 ± 0.49 mL; PEL-VEH: 7.21 ± 0.59 mL; F (1.5) = 0.113; P = 0.7). The NPEL-HAL group consumed 6.29 ± 0.15 mL and the PEL-HAL consumed 7.47 ± 0.49 mL (F(1.4) = 5.07, P = 0.087), the NPEL-VEH group and the PEL-VEH consumed 6.20 ± 0.66 mL and 7.21 ± 0.6 mL, respectively (F(1.4) = 2.809, P = 0.169) (Fig. 6A).
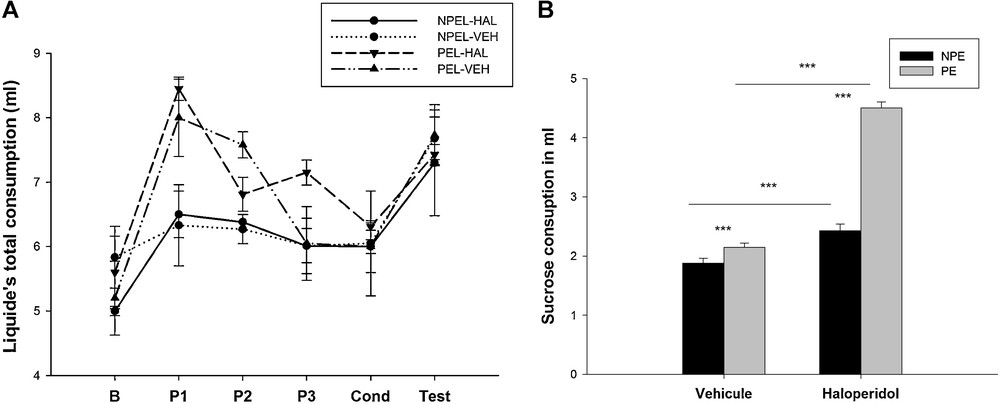
Total liquid consumption (sucrose and water) (A) and sucrose consumption (B) during every session (in mL), in control animals and after a neonatal bilateral administration of lidocaine into the ventral hippocampus. B: base line, P1–P3: pre-exposure session from day 1 to day 3. Cond: conditioning phase, Test: test day. PEL: pre-exposed lesioned group; NPEL: non-pre-exposed lesioned group; PE: pre-exposed control group; NPE: non-pre-exposed control group. Data presented as means ± SEM (***: P < 0.001).
During the test day, only sucrose consumption was evaluated. Three-way ANOVA analysis of the results was performed considering lesion, pre-exposure and haloperidol as main factors. This analysis showed a significant effect of lesion (F (1.32) = 53.683, P < 0.001), pre-exposure (F (1.32) = 20.541, P < 0.001) and haloperidol (F (1.32) = 134.461, P < 0.001) on aversive behavior (Fig. 6B).
The interactions between factors were highly significant: the effect of the lesion by pre-exposure (F (1.32) = 17.094, P < 0.001); between the effect of lesion by haloperidol (F (1.32) = 145.003, P < 0.001) and between the effect of pre-exposure by haloperidol (F (1.32) = 58.586, P < 0.001). The interactions between the three factors: lesion, pre-exposure, and haloperidol were also highly significant (F (1.32) = 74.486, P < 0.001).
4 Discussion
The present study had two main aims: first to validate lidocaine-based nVH lesion as a model of schizophrenia and second to determine the effect of a single injection of haloperidol (HAL) prior to the pre-exposure phase on latent inhibition (LI). Our results show that nVH lesion caused a significant reduction in social interactions, an increase in stress and anxiety and increased locomotor activity, as well as disruption of latent inhibition. Also, administration of HAL during the pre-exposure session showed facilitation of LI.
Lidocaine, a local anesthetic, when administered at a high dose, can cause neuronal damage by as yet unknown mechanism [35]. Blas-Valdivia [36] found that a bilateral injection of lidocaine into the ventral hippocampus of neonates caused a mild reduction of neurons and some structural alterations such as chromatin condensation, nucleus loss, and cell shrinkage. These structural abnormalities could underlie the neuronal atrophy observed in our study. Gold et al. (1998) [37] has shown that application of lidocaine to dorsal root ganglia neurons at concentrations greater than 10 mM causes neuronal death, and a 4-min application of 30 mM is sufficient to induce neuronal death.
The neurosurgical procedures used in the current study have been described previously (Lipska et al.) [4]. As the behavioral and cognitive effects were only present in the lesioned group and not in the sham operated controls, it is unlikely that the observed effects were due to the surgical procedure or the anesthesia used.
The analysis of the two sessions of the open field test for “emotional reactivity/exploration” showed differences in animal's behavior between the control and lesioned groups. Locomotor activity in the periphery of the apparatus, and the total locomotion decreased over the two sessions for the two groups. However, locomotor activity of lesioned animals remained higher during the second session, while immobility time increased only slightly compared to the controls. In general, these results are in accordance with the data reported in the literature concerning the open field test [38] the heuristic model of schizophrenia [3,12].
Given that locomotor activity is considered as an index of emotional reactivity in rodents [30], the increased locomotor activity in lesioned animals could be related to the alteration of dopaminergic afferents in the prefrontal cortex, indicating a high level of anxiety [37]. This alteration may lead to an overactive mesolimbic system, as suggested by Carter and Paycock [39]. Increase of dopamine levels in the nucleus accumbens has been shown to increase locomotor activity in animals. This nucleus is directly linked to the amygdala, from which it receives signals involved in emotional processing, and sends signals to areas involved in movement, such as the globus pallidus and the lateral nucleus of the amygdala [1]. Based on this, we hypothesise that the increased anxiety reported in this test could be explained by a diminution of the influence of the prefrontal cortex to the amygdala.
The marble burying test also showed that hippocampal damage significantly reduced the number of buried marbles, which is consistent with other studies on mice [33]. This test has been validated as an isomorphic model of anxiety [31]. The results of social interaction test showed that animals from the control group interact more than animals from the lesioned group, suggesting that the hippocampus is involved in anxiety and asocial attitude. Our results are consistent with those obtained in Sprague–Dawley lesioned rats by ibotenic acid [5] and by lidocaine [36]. This social deficit is attributable to both the time the rats spend during the social contacts and the number of contacts; i, e. each social encounter of lesioned rats, on average, is of shorter duration compared to controls. Several studies have shown the involvement of the hippocampus in the different sequences of social behavior (initiation, arrest, prosecution) [40]. Indeed, studies have shown that social behavior is sensitive to manipulations of dopamine and glutamate receptor agonists in D1 and D2 and an NMDA receptor antagonist (phencyclidine).
The disruption of latent inhibition has received increasing attention in animal models of schizophrenia [41]. Here, we tested the alteration of this paradigm after neonatal lesion of the ventral hippocampus. The weakening of the subsequent performance in pre-exposure CS position has been demonstrated repeatedly in two-way avoidance learning [42]. This paradigm requires some effort and involves locomotion, but which does not allow the animal to learn that there is a place of refuge. Thus, the most effective intrinsic index is absent and the animal must use external cues or changes in stimuli such as the interruption of the CS. The important feature of this index is that it does not indicate the end of a danger (associated with the shock), but it indicates the beginning of a safety signal (see [43]).
Our results clearly showed an impairment of latent inhibition in the lesioned group compared to the control. The pre-exposure to repeated NS induces selection and classification of the stimulus as irrelevant information in the control group, unlike the lesioned animals that, despite pre-exposure to-be-conditioned stimulus, classed it as relevant information [44]. These results are in agreement with those obtained by Caine et al. [45], Grecksch et al. [11], and Killcross et al. [46]. Several studies have shown that latent inhibition depends on the integrity of the hippocampal formation [47,48]. Indeed, neural activity in the hippocampus, amygdala and prefrontal cortex controls LI via their projections to the nucleus accumbens.
During the retention trial, the results showed that the lesioned group retained the association more readily than controls, supporting the conclusion that only latent inhibition was disrupted by nVH lesion but not acquisition or memory storage. Our control animals showed an increase in LI, because even if the session was performed without pre-exposure, the same degree of acquisition delay was observed. The passage of time may have important effects on LI. Several studies have postulated that the insignificance of the US is sensitive to context and time, i.e., the approach accommodates a loss of interference that occurs when either a context switch [49] or a retention interval [50] occurs between pre-exposure and learning session. There are also implications for the effects of time following the learning session, i.e., an increase in LI over time [51,52]. According to the same reasoning, we assumed that the disruption of latent inhibition increased over the time and that the association was very strong.
Several studies have investigated the pharmacology of LI in animal models. Studies on glutamatergic activity in the hippocampus have shown that infusion of NMDA receptor antagonists reduces LI [53], whereas studies on the manipulation of dopaminergic activity have provided a potential link between dopaminergic hyperactivity and cognitive dysfunction observed in schizophrenia [47]. Thus, an increase of dopamine by systemic injection of amphetamine impairs latent inhibition [46,54]. Further studies have shown that both the disruption and enhancement of LI by dopaminergic drugs is modulated by the mesolimbic DA system. For example, infusions of amphetamine in the nucleus accumbens disrupt LI, whereas haloperidol microinjected into the shell cells enhances LI [29,54]. A more recent study by Nelson et al. [55] has provided novel insights into DA mechanisms in LI. Their results indicated that in isolation D1 receptors do not play a role in the modulation of LI by the DA. Nonetheless, the demonstration that antagonism of D1 receptors restored normal LI in amphetamine-treated rats suggests that the activity of D1 receptors, likely through interactive effects with D2 receptors, contributes to the dopaminergic modulation of behavioral processes underlying LI [55].
In the present work, the effect of a single injection of haloperidol (HAL) during the pre-exposure phase of LI using the taste aversion conditioning paradigm was examined. The haloperidol dose (0.1 mg/kg) was selected on the basis of previous published reports [13,56] and that dose of 1 mg/kg is the maximum dose that can be used without effect on motor/locomotor and social behaviors after a single injection. Beker and Gresksch [57] have demonstrated that only subchronic (over 10 days) HAL administration could have an effect on social behavior. Here, we found that haloperidol administration significantly increased the volume of sucrose consumed by the lesioned group compared to the control. This result is similar to that obtained by Bethus et al. [57]. Conversely, others studies have demonstrated that administration of neuroleptics such as haloperidol during the conditioning phase facilitates LI and assumed that this effect was due to the increase in DA during the conditioning phase, but did not consider possible effects of DA during the phase of pre-exposure [26,47,58].
Several studies have examined the effects of injection of DA agonists during the pre-exposure phase, suggesting that increased systemic levels of DA during pre-exposure phase has no effect on LI [16,29]. However, all experiments to validate LI as a model to test antipsychotics have shown that many of these drugs are effective only when they are injected before the conditioning phase, but not before the pre-exposure [59–61].
Consistently with this view, data summarized by Lubow [62] show that LI is reduced when the context is changed between the pre-exposure and conditioning phases. It is further often suggested that one type of context shift that can result in reduced LI is that of the perceived internal state, usually termed “state-dependency”. It is unquestionable that our procedure involved such internal state shifts between the pre-exposure and the conditioning phases. Since many studies have shown that the dose we used can serve as a discriminative stimulus in drug discrimination studies [63,64], we should stress that HAL does have the potential for producing a perceived internal state. In order to explain our results, we propose that there is a different dopaminergic mechanism rather than a state-dependant effect. Another explanation is based on the number of pre-exposure sessions used. All of the reports that showed that pharmacological manipulation of DA during pre-exposure is ineffective in disruption of LI were based on the paradigm in which the pre-exposure was confined to a single pre-exposure session while the stimulus was still novel. In this study, by separating the session to 3 daily pre-exposure sessions, memory consolidation effects could be involved.
When HAL injection is given before each phase of PE, there was potentiation of LI. These results support the role of DA transmission in the acquisition of LI, as proposed by Schmajuk et al. [65]. The results of this study suggest that the dopamininergic system is involved in the expression of LI (learning of an association involving a pre-exposed stimulus) rather than the acquisition of LI (the cognitive process by which the stimulus loses its associative ability) [27].
In this study, we have demonstrated that nVH lesions caused behavioral changes related to schizophrenia, such as hyperlocomotion, anxiety, and LI disruption and lends support to the theory that exclusive administration of HAL to pre-exposure phase facilitates LI.
Disclosure of interest
The authors declare that they have no conflicts of interest concerning this article.
Acknowledgments
We thank Dr Howard M Cooper, INSERM 846, Department of Chronobiology, Bron, France, and Nilesh B. Patel, Department of Medical Physiology College of Health Science, University of Nairobi Kenya, for their critical reading of the manuscript. We also thank M. A. Regragui for his assistance for animal care.