1 Introduction
Early recognition of the pathogen by the plant is essential, when the plant activates the available biochemical and structural defence to protect itself from the pathogen. Once a particular plant molecule recognizes and reacts with an elicitor derived from a pathogen, alarm signals are transduced to the host cell molecules and to nuclear genes, causing them to become activated so as to produce pathogen-inhibiting substances. Defence reactions include the reinforcement of the cell wall by callose and/or lignin deposition, the production of reactive oxygen species (ROS), hypersensitive reactions (HR), multicomponent responses involving increased expression of defence-associated genes and localized cell death at the site of infection [1,2].
A series of defence reactions are induced in potato plant cells after treatment with hyphal wall components (HWC) prepared from P. infestans, including the generation of superoxide anions (O2−), induction of HR and phytoalexins production [3]. Sanchez et al. [4] observed that HWC also cause the HR of cells of solanaceous plants. An infiltration of Phytophthora-derived oligopeptide elicitor, Pep-13, into potato leaves induces the accumulation of hydrogen peroxide, defence gene expression and the accumulation of jasmonic acid and salicylic acids [5].
A rapid and transient production of ROS, termed “oxidative burst”, is a hallmark of successful recognition of plant pathogens and the oxidative burst could have a direct effect on the pathogen or the defences caused in plant because of its reactivity [6]. ROS could also contribute to the establishment of physical barriers via oxidative cross-linking of precursors during the localized biosynthesis of lignin and suberin polymers [7]. ROS mediate the generation of phytoalexins, antibiotic compounds and secondary metabolites that arrest pathogen growth [8]. ROS are also associated with HR, a localized response at the site of pathogen attack that displays programmed cell death and that could contribute to limit the spread of the pathogens or to be a source of signals for the establishment of further defences [1]. Hypersensitive cell death occurs at the infection site with apoptosis-like features. Despite controversial debate as to the role of ROS in plant HR, they are generally regarded as causes of hypersensitive cell death [9,10].
The most rapid responses of plants to potential pathogens are the congruent production of both nitric oxide (NO) and reactive oxygen intermediates. NO has a key role in plant–pathogen interactions and participates in the early stages of HR induction [11,12]. In an investigation on tobacco cell suspension culture for study of the relations between NO generation and several defence responses induced by INF1 elicitor, cPTIO, a NO scavenger, completely suppressed the activation of a 41-kDa protein kinase and cell death by INF1, which suggests that NO plays a crucial role in the induction of hypersensitive cell death [13]. Potato tubers treated with NOC-18, a NO donor, induced the accumulation of potato phytoalexins [14]. In induced biochemical defence, phytoalexin production is regulated by NO [15].
The synthesis of phytoalexins or phytoanticipins is considered to be an important part of the plant innate immune response to a variety of pathogens [16,17]. ROS within the cell or to the neighbouring cells often generates phytoalexins [18]. Lubimin and rishitin are two of the major sesquiterpenoid phytoalexins produced by potato, and the potato pathogen Gibberella pulicaris can degrade both [19]. Eicosapentaenoic and arachidonic acids extracted from the mycelia of P. infestans elicit fungitoxic sesquiterpenes in the potato [20]. Substances from germinating spores of P. infestans also elicit the accumulation of phytoalexin rishitin in potato tuber slices [21].
In the present study, authors used a liposoluble MEM elicitor derived from P. infestans with the aim of examining the effect of the MEM elicitor to ascertain the early reactions and to evaluate the induced resistant reactions in potato plants and potato suspension-cultured cells. This study also concerns defence strategy in potato – P. infestans interactions and defence activities in potato in response to the MEM elicitor.
2 Materials and methods
2.1 Plant materials
Potato suspension-cultured cells, leaves and tubers of cultivar Sayaka, which has resistant genes R1 and R3, were used. Potato suspension-cultured cells are very sensitive to early responses to MEM elicitor, whereas potato leaves were used to observe elicitor responses in more natural conditions. On the other hand, potato tubers gave the best results for phytoalexin production (unpublished data by Monjil and Kawakita). Potato plants were grown at 23 °C and 70% humidity under a 16-h photoperiod in environmentally controlled growth cabinets and the leaves were used for the experiments. Potato suspension-cultured cells (cv. Sayaka) were prepared from callus obtained from potato tuber discs in callus-inducing solid medium [22]. Potato suspension-cultured cells were subcultured every week and used for experiments 4–5 days after subculturing.
2.2 Pathogenic isolate Phytophthora infestans
The pathogenic isolate [P. infestans (Mont.) De Bary], race 1.2.3.4, was used as a mycelium culture and was maintained at the Plant Pathology laboratory of the Graduate School of Bioagricultural Sciences, Nagoya University, Japan. P. infestans strains, used as inocula for liquid culture, were grown on rye-seed extract agar medium in a test tube at 18 °C in the dark for two weeks. Growing of mycelia for methanol extraction was done in rye liquid nutrient [22]. For spray experiment, zoosporangia suspensions from the P. infestans isolates were prepared following the method reported by Monjil et al. [23]. Briefly, P. infestans isolates were subcultured on rye-media for 7–10 days. Twenty milliliters of water were added to the surface of the P. infestans colonies, which were then rubbed with a cotton swab to release zoosporangia. Zoospores from zoosporangia were taken out by keeping the water containing zoosporangia at 16 °C for 3 h. MEM-treated potato leaves were inoculated with 1-mL aliquots of P. infestans zoospores (2 × 105 zoospores/mL) and covered with lens paper to keep the suspension of zoospores on the surface of the leaves. The inoculated plants were kept at high humidity at 20 °C for one day after inoculation, and then moved to a growth room at 23 °C.
2.3 Preparation of MEM elicitor from P. infestans
MEM elicitor from P. infestans was prepared following the method described by Monjil et al. [24]. Frozen mycelia were ground by a mortar and pestle under a freezing temperature using liquid nitrogen. The ground mycelia were suspended in methanol at the rate of 10 mL of methanol per 1 g of mycelia. The mycelia suspension was finely grounded using a polytron-type homogenizer (HG30, Hitachi Koki, Japan) for 2 min. After centrifugation at 4 °C, 3000 g for 30 min, the supernatant was collected and dried by using an evaporator, and was used as an MEM elicitor. It can be noted that 1 g of mycelia from P. infestans yielded 88–90 mg of MEM elicitors.
2.4 Measurement of O2− production
The relative intensity of O2− generation in potato suspension-cultured cells was measured by counting photons from L-012-mediated chemiluminescence following the method described by Monjil et al. [22]. In plant leaves, O2− measurement was done as described by Kobayashi et al. [25]. For the detection of O2− production in leaf tissues, 0.5 mM L-012 in 10 mM MOPS-KOH (pH 7.4) was infiltrated intro potato leaves via a syringe without needle. Chemiluminescence was monitored continuously using a photon image processor equipped with a sensitive CCD camera in the dark chamber at 20 °C (Aquacosmos 2.5; Hamamatsu Photonics, Shizuoka, Japan) and quantified using the U7501 program (Hamamatsu Photonics).
2.5 H2O2 in situ detection
Leaf discs of potato plants infiltrated with elicitors were removed with a cork borer at 3, 6, 12 and 24 h after infiltration. H2O2 detection was performed following the DAB staining method described by [26]. Leaf discs were placed in 1 mg/mL 3,3′-diaminobenzidine (DAB-HCl) (Sigma, St. Louis, USA) and incubated at room temperature overnight. DAB reactions were examined in leaves cleared in boiling ethanol (96%) for 10 min. The samples were then stored in ethanol (96%) at room temperature or mounted in PBS (phosphate-buffered saline)/glycerol (50%) and kept at 4 °C for further examination. H2O2 was visualized as a reddish-brown coloration.
2.6 Detection of cell death
To detect the cell death on the plant, elicitors were infiltrated by using a syringe without needle from the opposite surface of the leaves. Cell death was measured by monitoring the death areas developed on the infiltrated leaf surface after 6, 12, 24 and 48 h of treatment with elicitors. Cell death was also checked by an electrolyte leakage method, which was adapted from the method previously described by [27]. Leaves were infiltrated by elicitors under the leaf surface, and leaf discs (1 cm in diameter) were collected from the leaf 3, 6 and 12 h after treatment. Seven leaf discs were floated on 7 mL of distilled water for 2 h at room temperature, and electrical conductivity was measured using a conductivity meter (Horiba, Kyoto, Japan).
2.7 Detection of phytoalexins
Potato phytoalexins produced in potato tubers were extracted using ethyl acetate following the method described previously [14], with a little modification. Concave holes (diameter 15 mm, depth 7 mm) were made in the parenchyma of the sliced tuber about 10–15 mm thick by using an electromotive drill. After aging at 20 °C for 24 h in dark humid chamber, 500 μL of each treatment were added to the concave hole and were incubated under the same conditions for 48 h. Phytoalexins were extracted from the collected liquid from the treated holes with equal amounts of ethyl acetate. The extract were separated on TLC plates (TLC aluminum sheet of silica gel 60, Merck, Whitehouse Station, USA), which were developed with cyclohexane:ethylacetate (1:1, v/v) and visualized by spraying with 75% sulfuric acid containing 0.5% vanillin, followed by heating at 120 °C. Phytoalexins were quantified by high-performance liquid chromatography (HPLC) analysis. Extracts were concentrated and dissolved in 200 μL of 70% MeCN in H2O. A portion (5 μL) of these solutions was analyzed by an HPLC system (Jasco Co., Tokyo) equipped with two PU-1580 pumps, a DG-2080-54 degasser, and an MD-2010 plus photodiode array detector, under the following conditions. Column: Develosil ODS-UG-5 (4.6 × 250 mm, Nomura Chemical), solvent: 50% − 50% − 90% − 100% (0 − 5 − 25 − 26 min) MeCN in H2O, flow rate: 1 mL/min, detection: UV 205 nm. HPLC data were processed by the Borwin program (Jasco Co.). The phytoalexins (oxylubimin, 10-epi-oxylubimin, rishitin, lubimin, and 10-epi-lubimin) were eluted at 5.7, 6.2, 10.3, 12.5, 13.2 min, respectively.
2.8 Measurement of NO production
NO production activity in potato suspension-cultured cells was measured by a fluorometric method using the NO indicator diaminofluorescein-2 (DAF-2 DA; Daiichi Pure Chemicals, Tokyo, Japan) [24]. In potato leaves, NO production was measured by infiltrating 200 mM of a sodium phosphate buffer at pH 7.4 and 12.5 μM DAF-2DA, using a needleless syringe; leaves were incubated for 1 h in the dark at room temperature before observation. Fluorescence from DAF-2 T, the reaction product of DAF-2DA with NO, was captured using a fluorescence stereomicroscope (MZ16FA, Leica) equipped with a CCD camera (Color 14 bit, AxioCam HRc, Carl Zeiss). The fluorescence intensity of the scanned entire field of the image captured by a CCD camera was quantified by determining the mean green channel values for the images with the histogram function of Adobe Photoshop 7.0 (Adobe, Seattle) [28]. A fluorescence image was obtained from an inoculated area per leaf, and at least three inoculated areas were analyzed as replicates for each treatment.
2.9 RNA preparation and RT-PCR
Expression of potato genes after the treatment of elicitors was analyzed by RT-PCR [29]. RT-PCR was conducted using a commercial kit (ReverTra-Plus-; Toyobo Co., Osaka, Japan). The cDNA was synthesized from total RNA (1 μg) with an oligo (dT) primer. After the cDNA synthesis reaction, PCR was performed with denaturing, annealing, and extension temperatures of 94 °C for 15 s, 55 °C for 30 s, and 72 °C for 30 s, respectively. Gene-specific primers for each sequence were as follows: StEF-1α forward primer, 5′-CATTGTGCTCATTGGCCACG-3′, StEF-1α reverse primer, 5′-CCAGTCGAGGTTGGTAGACC-3′, StrbohA forward primer, 5′-TTAGCAGCTCGAGCATTACG-3′, StrbohA reverse primer, 5′-TCCTTCATACCGATGCATTG-3′, StrbohB forward primer, 5′-ATCGAAAACACGAGGGATTC-3′, StrbohB reverse primer, 5′-GCACCAGACTTACTCCTATC-3′, StrbohC forward primer, 5′-CGGAAAATCATCACCCGCAC-3′, StrbohC reverse primer, 5′-CGGCTCCGGCACTAGTTCCG-3′, StPR1 forward primer, 5′-GTGCTAGAGTCAAGTGCAAC-3′, StPR1 reverse primer, 5′-GAATCAAAGTCTGGTTGCTC-3′, StNR5 forward primer, 5′-TACAACATTGGCATTGGCTTG-3′, StNR5 reverse primer, 5′-AACATTTTATACCTAAAGTTAACAA-3′, Sthsr203J forward primer, 5′-AAGCTGATTGGTACATGTACTATGC-3′, Sthsr203J reverse primer, 5′-TAGCTCCGATTTACTTCGCTG-3′, StPVS3 forward primer, 5′-TTGTCTGCTGCTGCTTGTGG-3′, and StPVS3 reverse primer, 5′-TCTCCATGAGTCCTTACATG-3′.
2.10 Resistance-inducing activity against pathogen infection
Potato leaves were grown and used for resistance induction study. MEMs at the concentration of 100 μg/mL were spayed into potato leaves using a hand sprayer. DMSO treatment was used as a control. After 24 h of treatment, challenge inoculation was done by P. infestans (2 × 105 zoospores/mL), and potato leaves were kept in dark and humid conditions for 18 h.
3 Results
3.1 MEM elicitor and its O2− producing activity
Our MEM elicitor contains relatively low-molecular-weight compounds because of the limited solubility of high-molecular-weight compounds or proteins in methanol. The oxidative burst (rapid and transient production of O2−) in potato is an early response induced by elicitor treatment. MEM was added to potato suspension-cultured cells and O2− producing activity was measured by using an O2− unique luminous reagent L-012 (Fig. 1). MEM induced rapid O2− generation in a dose-dependent manner (Fig. 1a). DMSO (3%) was used as a control because the MEM elicitor was dissolved in 3% DMSO. A time course experiment was done up to 6 h and indicated that O2− generation was rapidly induced by MEM, peaked at 3 h after the treatment, and slowly decreased until 6 h (Fig. 1b). In potato leaves, induction of O2− production by the MEM elicitor was observed as enhanced L-012 mediated chemiluminescence in infiltrated sites (Fig. 2a). Then, 3, 6, 12 and 24 h after the elicitor treatment, rapid O2− production was detected by the MEM elicitor within 3 h, and the maximum elicitor activity was observed 6 or 12 h after treatment; lower O2− production was observed after 24 h (Fig. 2b).

O2−–inducing activity of MEM in potato suspension-cultured cells. a: the dose dependency of MEM was measured in potato suspension-cultured cells treated with 1/10 volume of MEM to get final concentrations 1 μg/mL, 25 μg/mL, 50 μg/mL, 100 μg/mL, and 200 μg/mL, respectively. DMSO (3%) was used as a control. The O2−-producing activity was measured in a 96-well microtiter plate with L-012 using chemiluminescence plate reader Mithras LB 940; b: time course of the O2− producing activity of potato suspension-cultured cells by 100 μg/Ml MEM up to 6 h. Data are means ± standard deviation from five independent experiments.
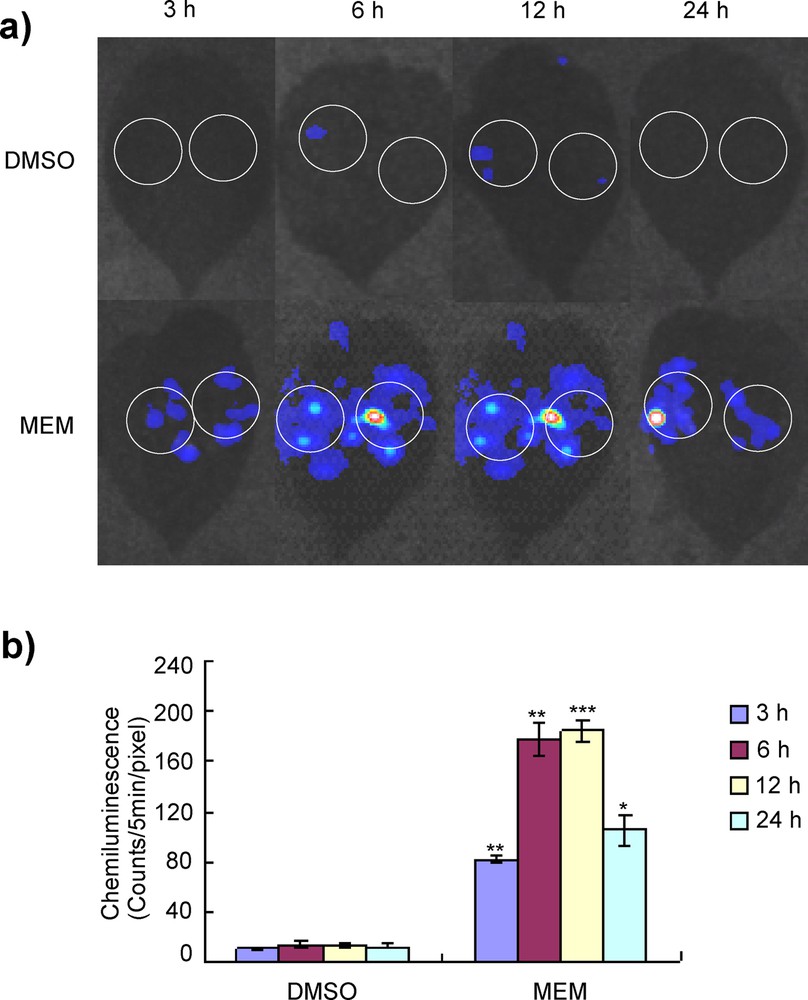
(Color online.) O2−-inducing activity of MEM in potato leaves. a: the O2−-producing activity of potato leaves was measured by treatment with MEM elicitors. MEM (0.5 mg/mL) was used for this study. DMSO (3%) was used as a control. Elicitor-treated leaf areas were infiltrated with L-012 solution 3, 6, 12 and 24 h after treatment, and were monitored using a CCD camera. White circles indicate areas infiltrated with L-012; b: chemiluminescence intensities were quantified with a photon image processor. Data are means ± standard deviation from three independent experiments. Data were subjected to Student's t-test. *P < 0.05, **P < 0.01, ***P < 0.001 versus DMSO-treated control treatment.
3.2 Hydrogen peroxide-producing activity
H2O2 generation was detected after MEM treatment and stained with DAB (Fig. 3). DAB captures H2O2 and forms a reddish-brown coloration at sites of peroxidase activity. DAB staining was observed in potato leaves at different time points, namely 3, 6, 12 and 24 h after MEM treatment. DAB staining was observed in any time point, indicating that H2O2 producing activity is induced by MEM.

(Color online.) H2O2 accumulation by MEM in potato leaves. Accumulation of H2O2 by MEM at the concentration of 0.5 mg/mL in potato leaves after incubation of leaf tissues with the dye DAB were determined after 3, 6, 12 and 24 h of treatment. DMSO (3%) was used as a control. The reddish-brown coloration indicates H2O2 accumulation. The results shown are representative of at least three separate experiments.
3.3 Phytoalexins accumulation
Phytoalexins accumulation in potato tuber was measured by treatment with 0.1, 0.5 and 1 mg/mL of MEM (Fig. 4a). DMSO (3%) was used as a control. Purified rishitin was used as a marker for phytoalexin detection in TLC. HPLC analysis showed that MEM induced several phytoalexins accumulation, particularly rishitin, oxylubimin and lubimin in potato tubers (Fig. 4b and c). 10-epi-Oxylubimin and 10-epi-lubimin are two stereoisomers that were also observed in HPLC analysis.
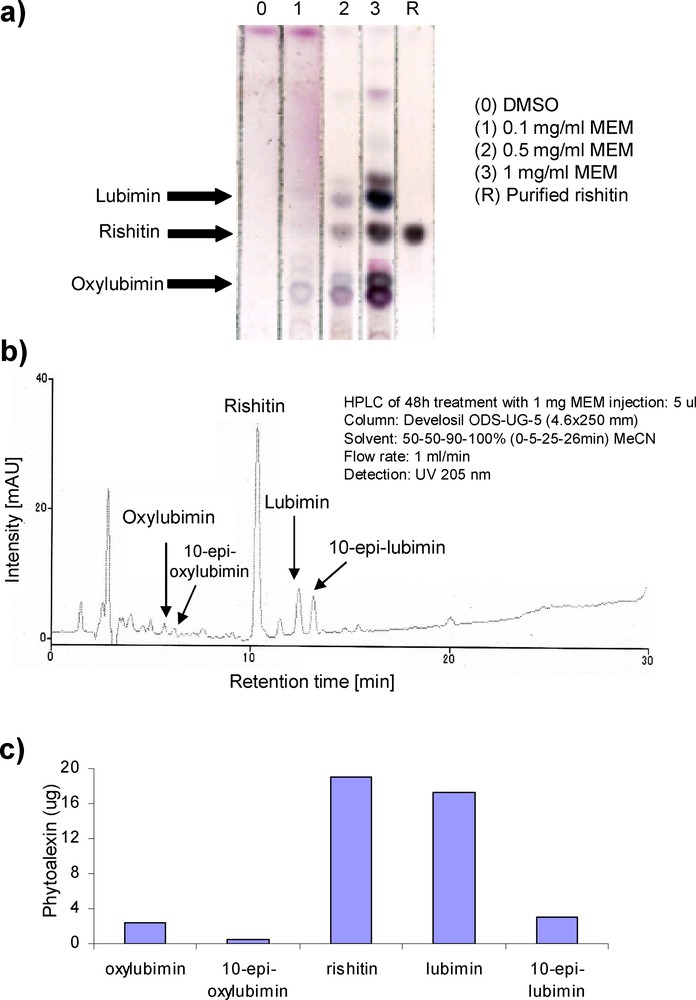
(Color online.) Production of phytoalexins by MEM in potato tubers. a: for TLC experiment, potato tubers were treated with MEM at three concentrations (0.1, 0.5 and 1 mg/mL), and phytoalexins were extracted 48 h after treatment. DMSO (3%) was used as a control. Equal load of extracted samples and 25 μg of purified rishitin were separated and developed on a TLC plate. Black arrows indicate the site of rishitin, lubimin, and oxylubimin development in a thin-layer chromatography plate; b: HPLC analysis of rishitin, lubimin and oxylubimin at 1 mg/mL concentration; c: quantification of rishitin, lubimin and oxylubimin from the HPLC peak.
3.4 Induction of cell death
HR-like cell death was examined in potato leaves. In this experiment, average-sized potato leaves (30–40 days after germination) were selected and infiltrated by elicitors by using a syringe without needle from the lower surface of the leaves. Cell death was detected by monitoring the dead areas developed on the leaf's surface 6, 12, 24 and 48 h after treatment with MEM elicitor. As a result, the dead areas were detected within 6 h after treatment with MEM. Elicitor-treated areas became water-soaked like death after 24 h and dried after 48 h (Fig. 5a). Ion leakage has been observed as an indicator of plant cell death [30]. A higher degree of ion leakage was detected in MEM elicitor-treated leaves after 6 h compared to DMSO-treated leaves (Fig. 5b).

(Color online.) Induction of cell death by MEM in potato leaves. a: potato leaves were infiltrated with 0.5 mg/mL of MEM and incubated for 48 h. Photographs were taken 6, 12, 24 and 48 h after elicitor treatment. Hypersensitive cell death was observed as a water-soaked lesion 6 h after treatment, which gradually showed increased cell death and by 24 h only dead areas remained in the treated leaf areas. DMSO (3%) was used as a control. The results shown are representative of three separate experiments; b: electrolyte leakage of dead cells of potato leaves. After treatment with elicitors by infiltration into the leaf, leaf discs were floated on distilled water and shaken for 2 h at room temperature. The electrical conductivity was measured using a conductivity meter. Data are means ± standard deviation from five replications. Data were subjected to Student's t-test. *P < 0.05, **P < 0.01 versus DMSO control treatment.
3.5 Nitric oxide-producing activity
NO generation was detected by DAF-2DA-mediated fluorescence in MEM elicitor-treated potato suspension-cultured cells (Fig. 6a). To scavenge NO responsive events in cells, a potent NO scavenger, cPTIO, was used. NO generation in suspension cells treated by MEM was eliminated by cPTIO. A similar experiment was done in potato leaves. The leaf areas were treated with the MEM elicitor and infiltrated with the DAF-2DA solution, and NO production was monitored 1 h later using fluorescence stereomicroscopy. Fluorescence was detected 6 and 12 h after elicitor treatment and eliminated by addition of cPTIO (Fig. 6b and c), indicating that the MEM elicitor induced NO in potato leaves.
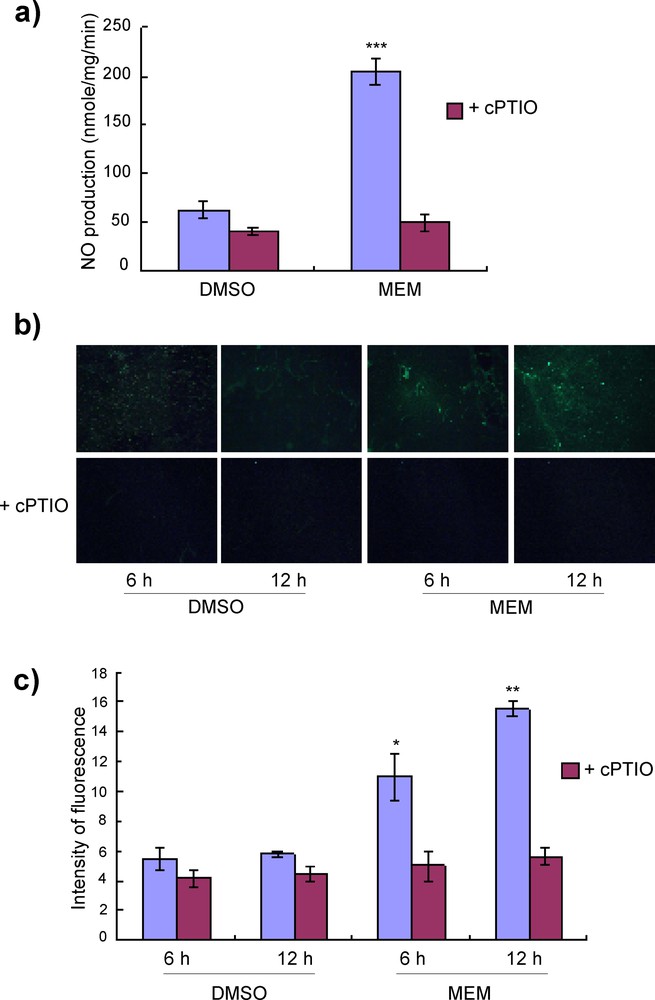
(Color online.) Induction of nitric oxide by MEM in potato. a: NO generation by the MEM elicitor at the concentration of 100 μg/mL in potato suspension-cultured cells 3 h after treatment with or without cPTIO. DMSO (3%) was used as a control. The cells were incubated with 10 μM DAF-2 DA for 1 h prior to measurement with a fluorescence spectrophotometer. The excitation and emission wavelengths for DAF-2D were 495 nm and 515 nm (band paths 3 nm and 3 nm), respectively. Each value represents the mean and standard deviation of three replicates; b: NO generation by MEM at the concentration of 0.5 mg/mL in potato leaves 6 h after treatment. The treated leaves were infiltrated with DAF-2 DA and were monitored 1 h later by fluorescence stereomicroscopy. In the case of cPTIO treatment, treated leaf areas were infiltrated with 0.5 mM cPTIO 1 h before infiltration of DAF-2DA; c: signal intensities were quantified by determining the mean channel values for the images 6 or 12 h after treatments, respectively with the histogram function. The results shown are representative of at least three separate experiments. Data were subjected to Student's t-test. *P < 0.05, **P < 0.01, ***P < 0.001 versus DMSO control treatment.
3.6 Expression of defence-related genes
To investigate whether the defence-related genes are induced by the MEM elicitor, total RNAs from MEM-treated potato suspension-cultured cells were extracted and analyzed by RT-PCR (Fig. 7). MEM elicitor expressed ROS production regulatory genes, Rbohs (respiratory burst oxidase homologue). A high expression of StrbohA and StrbohC, and weak expression of StrbohB was observed. The expression of the hypersensitive response marker gene Sthsr203J indicated that MEM generated ROS-inducing HR. StPVS3 encoding potato vetispiradiene synthase (PVS) was found highly expressed with MEM treatment. High expression of defence gene StPR1, a pathogenesis-related protein 1, was found by MEM elicitor treatment. The MEM elicitor also induced StNR5 transcript, nitrate reductase 5, a nitric oxide-producing enzyme induced by pathogen or elicitor signals.

Gene expression by MEM in potato suspension-cultured cells. Total RNAs were isolated from potato suspension-cultured cells treated for 3 h with 1/10 volume of MEM to get final concentrations of 50 μg/mL and 100 μg/mL, respectively. DMSO (3%) was used as a control. RNAs were analyzed by RT-PCR using specific primers for StrbohA, StrbohB, StrbohC, StPR1, StNR5, Sthsr203J, and StPVS3. Equal loads of cDNA were monitored by amplification of constitutively expressed StEF-1α. Three repetitions were performed.
3.7 MEM induced disease resistance in potato against P. infestans
To investigate the resistance activities during the interaction between MEM-treated potato leaves and P. infestans, MEM-treated potato leaves were inoculated with P. infestans. MEM (100 μg/mL) was sprayed in excised leaves; one day later, inoculation was done by P. infestans. Within 3 days after the inoculation of P. infestans, DMSO-treated plant leaves showed water-soaked disease symptoms on inoculated leaves (Fig. 8a), and the disease lesions almost extended over entire leaves within 6–7 days. In contrast, few spots were developed in leaves treated with the MEM elicitor (Fig. 8a). The interaction between potato leaves and P. infestans was examined using light microscopy in inoculated leaves after staining with lactophenol trypan blue, which is indicative of cell death. As a result, densely stained epidermal and mesophyll cells along with fungal structures were observed in the DMSO-treated leaves six days after inoculation (Fig. 8b). On the other hand, less stained cells were observed in MEM-treated leaves. The percentage of potato leaves with disease severities up to 6 days showed the suppression of disease in MEM-treated potato leaves (Fig. 8d). From these results, it can be concluded that MEM induces resistance in potato plants against P. infestans.

(Color online.) MEM induced resistance against Phytophthora infestans in potato. a: DMSO-treated control plant leaves and MEM potato leaves were kept in an incubator for 24 h and then were inoculated with the spores of P. infestans. Photographs were taken from the 6th day after inoculation; b: microscopic observation of potato leaves from the 6th day after inoculation with P. infestans. Inoculated leaves were stained with lactophenol trypan blue to visualize the dead plant cells. Bar = 70 μm; c: appearance of disease symptoms showing differences in severity representative of the five classifications used in D; d: plots showing percentages of potato leaves with disease symptom severities in each of the five classes represented in D, for leaves of DMSO and elicitor-treated plant leaves inoculated with P. infestans from 1 to 6 days post-inoculation. At least 10 inoculated leaves from each plant were counted.
4 Discussion
One of the most rapid defence reactions to pathogen attack is the so-called oxidative burst, which constitutes the production of ROS, primarily O2− and H2O2, at the site of the attempted invasion [31]. In the present study, it was shown that the MEM elicitor induced O2− production as well as that of H2O2 in potato (Figs. 1, 2 and 3). Doke [32] reported that O2− generation is detected in potato tissues as a resistance reaction induced by the invasion of P. infestans or treatment of hyphal wall components (HWC) from P. infestans. It had been reported that the oxidative processes were induced in cell suspensions of Solanum species by culture filtrate of P. infestans [33]. These results, in conjunction with this study, provide evidence that substantial O2− generation and peroxidation occurred in MEM-elicited potato suspension-cultured cells as well as in potato leaves.
When O2− and H2O2 levels are increased in plants in response to an attack by a pathogen, they were followed by a hypersensitive reaction that leads to the death of host cells [34]. MEM showed the induction of HR by cell death on potato leaves (Fig. 5). Kamoun et al. [35] reported that the elicitins, one of the protein elicitors from Phytophthora species, were regarded as avirulence factors, as they cause HR-like lesions when infiltrated into tobacco. It was also reported that HWC from P. infestans activates O2− generation, which is responsible for triggering hypersensitive cell death in the solanaceous host plants, tomato, sweet pepper, and tobacco [36].
There is a good correlation between the degree of host resistance and the level of phytoalexins accumulation. Rishitin and/or solavetivone are phytoalexins that were reported to inhibit the growth of different microorganisms, including P. infestans [37,38]. Rishitin was also shown to inhibit Botrytis cinerea mycelial growth in both solid and liquid media [39]. The results presented in this article showed that MEM induced the accumulation of phytoalexins, particularly rishitin, lubimin and oxylubimin in potato tubers (Fig. 4). Doke [32] reported that O2− generation is closely connected with rishitin production by HWC. ROS can mediate the generation of phytoalexins and of secondary metabolites that arrest the pathogen's growth [8]. It had been reported that N,N-dimethylsphingosine induces phytoalexins production and hypersensitive cell death of Solanaceae plants without generation of ROS [40]. Noritake et al. [14] published that the treatment of potato tuber tissues with NOC-18, a NO donor, induced the accumulation of the potato phytoalexin, rishitin. The MEM elicitor investigated in this study also induced NO generation in potato leaves and suspension-cultured cells (Fig. 6). In addition to phytoalexins production, NO induced other plant defence responses, including cell death [41]. Wilson et al. [42] reported that NO is involved in cell death and defence against biotic and abiotic stresses. Thus, it was suggested that the MEM elicitor induced phytoalexins production on potato tubers due to the activation of superoxide anion and or nitric oxide in plant cells.
In several experiments, ROS- and HR-mediated gene expression was confirmed by some researchers. The treatment of potato tubers with a HWC elicitor caused a rapid but weak transient accumulation of H2O2 (phase I), followed by a massive oxidative burst 6 to 9 h after treatment (phase II), to which the expression of StrbohA and StrbohB, respectively, contributes [43]. The transcript level of StrbohC increased drastically and continually in potato leaves after treatment with the HWC elicitor [44]. The treatment of the HWC elicitor from P. infestans or infection of P. infestans with potato tubers caused transient increases in the transcript level of PVS3 during, not only incompatible, but also compatible interactions [45]. Hsr203J encodes proteins that have homology with esterase, and has been used as a marker of HR [46,47]. Taylor et al. [48] proved that StPR1 gene is rapidly activated in potato leaves treated with fungal elicitor. It is reported that elicitor-treated NO production in potato tubers induces StNR5 gene expression [13]. In potato suspension-cultured cells, a group of defence response-related genes, namely Sthsr203J, StPVS3, StrbohA, StrbohB, StrbohC, StPR1, and StNR5 were expressed by the MEM elicitor treatment (Fig. 7). These evidences indicate that the MEM elicitor plays an important role in defence-related gene expression.
For examining the defence role of MEM-treated potato leaves, some of them were inoculated with P. infestans. A higher level of resistance in potato was stimulated by MEM against P. infestans (Fig. 8). The resistance might be systemic because spraying MEM over a potato leaf surface before challenge inoculation induced resistance along with systemic resistance, inducing StPR1 gene expression. The activation of systemic-acquired resistance (SAR) correlates with the expression of the pathogenesis-related 1 (PR1) gene [49,50]. Treatment of susceptible potato cultivars with DL-β-aminobutyric acid induced enhanced resistance against P. infestans [51,52]. PAMP-triggered defence responses in potato include the accumulation of the signalling molecules salicylic acid (SA) and jasmonic acid (JA), defence gene activation, and hypersensitive cell death [5].
All these results provide a better understanding of the mechanisms by which the MEM elicitor induced defence response in potato. It was shown that MEM from P. infestans has the potentiality to induce O2− production, hypersensitive cell death, phytoalexins, NO production and defence-related gene expression in potato. Enhanced disease resistance against P. infestans might be due to a MEM-inducing series of responses in plant cells. The authors considered that further purification of MEM is necessary to obtain the compound(s) responsible for O2− production.
Acknowledgements
The authors thank Dr. M. Mori and Dr. S. Tsuda (National Agricultural Research Center for Hokkaido Region, Japan) for providing potato tubers.