1 Introduction
The development of powerful bioindicators has provided a basis for setting up various monitoring systems throughout the world, starting in 1975 with Mussel Watch [1], focused on monitoring the concentration of pollutants in marine waters by means of this bio-accumulator organism. More recently, the European Water Framework Directive (WFD), adopted in 2002 [2], makes use of a panel of bioindicators to assess the ecological status of a body of water [4,4]. Among the Biological Quality Elements adopted, the seagrass P. oceanica (L.) Delile was used for the Mediterranean; several integrative indicators have been developed along these lines: BiPo [5], POMI [6] and PREI [7], applied in different eco-regions.
Because of their ecological roles, their sensitivity to disturbances and their very extensive geographical range, seagrasses often constitute excellent biological indicators [3,8]. Several networks based on these species have thus been developed over the past decades in order to investigate the general state of the ecosystem [9,10]. The first network was the Posidonia Monitoring Network for the Provence-Alpes-Côte d’Azur region (PMN), founded in 1984 [11]; this initiative was extended in 1988 in the Euro-Mediterranean region with Cost 647 (Spain, France, Italy and Greece; [12]). In 2001, the first worldwide network, SeagrassNet, was set up in the United States [13]; it now includes 122 sites in 33 countries (two in the Mediterranean, one at Calvi in Corsica; http://www.seagrassnet.org/).
The development of monitoring networks in the Mediterranean has been strengthened by the adoption by the contracting parties of the Barcelona Convention, the Action Plan for the Conservation of Marine Vegetation in the Mediterranean Sea [14] and the guidelines for the Standardization of Mapping and Monitoring Methods for Mediterranean Seagrasses [15]. For this purpose, many Mediterranean countries have set up monitoring networks for seagrasses [10,16–18]. These networks have generally a two-fold purpose: (i) to monitor the state of conservation of the P. oceanica meadows, and (ii) to use the Posidonia oceanica meadows as an indicator of the quality of the environment.
The regression of seagrass meadows is a worldwide phenomenon observed over several decades, though the amplitude of this regression varies depending on the species and geographical zones under consideration [19]. In the Mediterranean Sea, P. oceanica is subject to natural and anthropogenic pressures and even if the decline of meadows seems in general to be relatively limited (between 0% and 10% throughout the 20th century; [20–22]), more significant rates of decline (up to 5%–8% per year) have been observed locally in sectors subject to strong anthropogenic pressures [23–25].
However, new pressures, indirectly or directly linked to global change, could be the cause of significant regressions, notably the introduction of exotic species, the rise of Sea-Surface Temperature (SST) and of the sea level [26–32]. However, in the absence of sufficiently long and precise series (baseline) regarding the distribution and vitality of the meadow, it is often difficult to confirm these hypotheses and/or to assess with precision the extent of the regression observed.
The aim of the present work is to monitor the distribution and the vitality of the P. oceanica meadow at 15 sites along 1000 km of coastline (Corsica Island, north western Mediterranean basin) in order to assess the dynamic of the meadow and to determine the possible causes of the changes observed (direct human impact, global change). Developed between 2004 and 2007, this monitoring network is based on the PMN method but one site is also monitored using the SeagrassNet protocol in order to inter-calibrate the two monitoring methods.
2 Material and methods
The 15 sites taken into account correspond to sites with no or with low identified human pressure (significant distance to city/harbor, low exploitation of living resources without trawling, no urban or industrial discharge, Marine Protected Areas) and sites exposed to significant human pressure; the Stareso site is also monitored on the basis of the SeagrassNet network (Fig. 1).
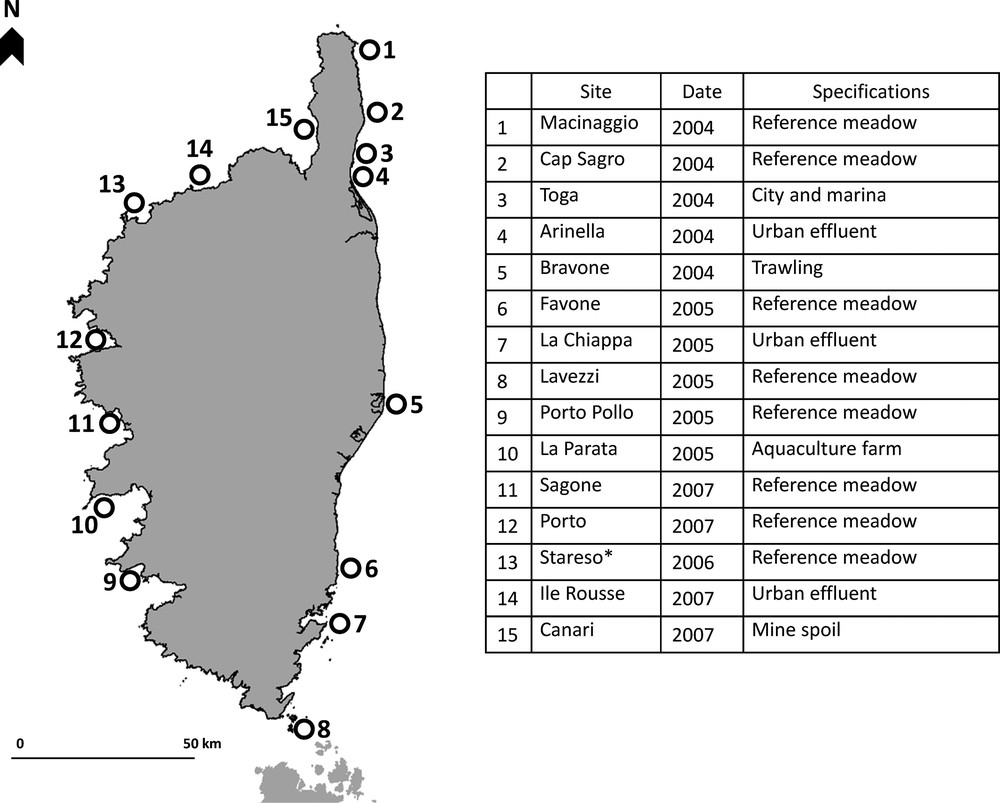
Location, environmental specifications and dates of setting up of the 15 sites of Posidonia Monitoring Network (PMN) along the coast of Corsica. *: SeagrassNet and PMN site (common site).
For two depths, specific measurements were taken at each site for the PMN (an intermediate depth between 14 and 16 m depth and the lower limit) and for three depths for the SeagrassNet, the upper limit (8 m), an intermediate depth (25 m) and the lower limit (37 m).
The PMN sites were set up between 2004 and 2007 (measurements recorded between May and July) and there were follow-up visits to each site in 2013 during the same period (between May and July, and ± 8 days in relation to the original measurement dates); the SeagrassNet system was initiated in October 2006 with follow-ups in October 2009 and October 2012.
The PMN procedure consisted in setting up eleven fixed markers every five meters, along the edge of the lower limit of the meadow; the precise position of each marker was then recorded with a compass [11]. A detailed map of the position of the meadow along the limit was established on the basis of these compass bearings and of vertical photographs taken and then assembled; horizontal photographs of the meadow above its limit were also taken at the level of each marker to compare the changes in the location of the limit over time ([33]; Fig. 1).
Several descriptors were also measured, at the intermediate depth and the lower limit, in order to assess the vitality of the meadow (Table 1) and the quality of the water body in which it was growing (BiPo index [5]; Table 2). The interpretation of the measurements is based on the standardized scales validated by the Barcelona Convention [15] and the Ecological Quality Ratio calculated in the BiPo index [5].
Assessment of the vitality of the meadow, based on several relevant descriptors, according to [14] adapted.
Location | Descriptors (Metric) |
High (5) |
Good (4) |
Normal (3) |
Poor (2) |
Bad (1) |
Lower limit | Lower limit type | Progressive (P) | Sharp high cover (S+) | Sharp low cover (S−) | Sparse (Sp) |
Regressive (R) |
Lower limit | Lower limit depth (m) | > 34.2 | 34.2–30.4 | 30.4–26.6 | 26.6–22.8 | < 22.8 |
Lower limit | Cover (%) | > 35 | 35–25 | 25–15 | 15–5 | < 5 |
Lower limit | Plagiotropic rhizomes (%) | > 70 | 70–30 | < 30 | – | – |
Lower limit | Leaf production (leaf number·a−1) | ≥ 8.0 | 7.9–7.0 | 6.9–6.0 | 5.9–5.0 | < 4.9 |
Lower limit | Rhizome elongation (mm·a−1) | ≥ 8.0 | 7.9–6.0 | 5.9–4.0 | 3.9–2.0 | < 1.9 |
15 m | Leaf surface (cm2·shoot−1) | > 362 | 361–292 | 291–221 | 220–150 | < 150 |
15 m | Density (shoot·m−2) | > 339 | 339–239 | 238–172 | < 172 | Na |
Assessment of water body quality (EQR) based on the BiPo index according to [4]. The lower limit types have been modified according to [14] (See Table 1 for abbreviations). X corresponds to the value measured for each descriptor; for instance a lower limit observed at a depth of 30 m corresponds to an EQR of 0.7375 ((((30−25)/6) × 0.225) + 0.55).
Location | Descriptors (Metric) |
High (1.000–0.775) |
Good (0.774–0.550) |
Normal (0.549–0.325) |
Poor (0.324–0.100) |
Bad (< 0.100) |
|
Lower limit | Lower limit depth (m) | Values EQR |
> 31 (((X–31)/7 × 0.225) + 0.775) |
25–31 ((((X–25)/6) × 0.225) + 0.55) |
19–25 ((((X–19)/6) × 0.225) + 0.325) |
< 19 (((X/19) × 0.225) + 0.1) |
Na 0.05 |
Lower limit | Lower limit type | Values EQR |
P 1.00 |
S+ 0.89 |
S− 0.66 |
Sp 0.44 |
R 0.21 |
15 m | Density (shoot·m−2) |
Values EQR |
> 339 (((X–339)/260 × 0.225) + 0.775) |
239–339 (((X–239)/100 × 0.225) + 0.55) |
172–238 (((X–172)/67 × 0.225) + 0.325) |
< 172 (((X/172) × 0.225) + 0.1) |
Na 0.05 |
15 m | Leaf length (mm) | Values EQR |
> 812 ((((X–812)/143) × 0.225) + 0.775) |
651–812 ((((X–651)/161) × 0.225) + 0.55) |
481–651 ((((X–481)/170) × 0.225) + 0.325) |
< 481 (((X/481) × 0.225) + 0.1) |
Na 0.05 |
The SeagrassNet protocol requires three permanent transects of 50 m length parallel to the coastline, at the three depths defined by the protocol [33]. The in situ measurements (cover, density) and the sampling (P. oceanica shoots) are carried out at predefined distances along each transect (12 measurements and samples per transect). Density is measured in 25 cm × 25 cm quadrats and cover is estimated in 40 cm × 40 cm quadrats by means of video images. The shoots collected are divided into three parts: leaf, rhizome (maximum length of 10 cm) and sheaths. The length of the longest leaf determines the canopy height. Each shoot part is then dried and weighed in order to measure the biomass.
3 Results
Of the 165 PMN markers, placed along the lower limit, only three were not recovered in 2013, or less than 2%; these were replaced on the basis of the compass bearings taken when they were initially installed.
The meadow had regressed above 73 markers, it had remained stable for 63 and it had progressed for 29; while the regressive dynamic is high (44%), the meadow had progressed or remained stable in the case of more than half of the markers (Table 3). With regard to the sites, at six sites the meadow had regressed, eight had remained stable and one had progressed. The highest regressions observed also concerned both the sites with the highest human pressure impact (Arinella and La Parata) and the reference sites (Cap Sagro and Porto Pollo). The only progression was recorded at the Canari site.
Patterns of change in the location of P. oceanica lower limit above the marker (PMN). For each site, synthesis is based on the mean evolution of the 11 markers (position of the median).
Site | Progression | Stability | Regression | Synthesis |
Macinaggio (ref) | 0 | 8 | 3 | Stability |
Cap Sagro (ref) | 0 | 1 | 10 | Regression |
Toga | 2 | 4 | 5 | Stability |
Arinella | 0 | 0 | 11 | Regression |
Bravone | 1 | 8 | 2 | Stability |
Favone (ref) | 0 | 11 | 0 | Stability |
La Chiappa | 0 | 7 | 4 | Stability |
Lavezzi (ref) | 3 | 2 | 6 | Regression |
Porto Pollo (ref) | 0 | 0 | 11 | Regression |
La Parata | 0 | 0 | 11 | Regression |
Sagone (ref) | 3 | 5 | 3 | Stability |
Porto (ref) | 5 | 6 | 0 | Stability |
Stareso (ref) | 1 | 4 | 6 | Regression |
Île Rousse | 4 | 6 | 1 | Stability |
Canari | 10 | 1 | 0 | Progression |
Total | 29 | 63 | 73 | Stability |
The typology of the lower limit remained the same for 8 sites out of 15; in contrast, for the 7 other sites, the differences observed always reflect a degradation of the limits, in particular for the sites of Cap Sagro, Arinella and Porto Pollo, where the lower limit declined from ‘sharp high cover limit’ to ‘regressive limit’ (Table 4).
Patterns of change in the lower limit type and meadow density between the setting up (2004–2007) and the monitoring phase (2013). Mean ± standard deviation (95%).
Type of limit | Density (nb shoot·m−2) | |||
Site | Setting up | Monitoring phase | Setting up | Monitoring phase |
Macinaggio (ref) | Progressive | Progressive | 150 ± 31 | 69 ± 10a |
Cap Sagro (ref) | Sharp high cover | Regressive | 134 ± 23 | 55 ± 11a |
Toga | Regressive | Regressive | 261 ± 33 | 142 ± 28a |
Arinella | Sharp high cover | Regressive | 130 ± 21 | 41 ± 7a |
Bravone | Regressive | Regressive | 121 ± 22 | 83 ± 11a |
Favone (ref) | Sparse | Sparse | 79 ± 19 | 53 ± 11a |
La Chiappa | Sharp low cover | Regressive | 112 ± 34 | 79 ± 13 |
Lavezzi (ref) | Progressive | Progressive | 127 ± 17 | 134 ± 17 |
Porto Pollo (ref) | Sharp high cover | Regressive | 75 ± 10 | 48 ± 8a |
La Parata | Sparse | Regressive | 80 ± 14 | 43 ± 11a |
Sagone (ref) | Progressive | Sharp low cover | 181 ± 42 | 138 ± 25 |
Porto (ref) | Sharp low cover | Sharp low cover | 122 ± 43 | 110 ± 21 |
Stareso (ref) | Sharp high cover | Sharp low cover | 67 ± 12 | 50 ± 10a |
Île Rousse | Regressive | Regressive | 42 ± 8 | 62 ± 10a |
Canari | Sharp high cover | Sharp high cover | 128 ± 25 | 242 ± 26a |
a Significant difference (Student t test, p value > 0.05).
A similarly regressive dynamic was found for the density recorded above the markers (Table 4). During the study period, 11 sites out of 15 showed a significant change in this descriptor: nine sites presented a decline in the mean density whereas two presented an increase. The most significant decline concerned the site of Arinella, which dropped from 130 ± 21 to 41 ± 7 shoots per square meter (confidence level 95%). Over the whole set of sites, the mean density declined from 121 ± 27 to 90 ± 28 shoots per m2, or a decline of 25%.
The mean vitality of the meadow, calculated on the basis of all the eight descriptors measured at the lower limit and at intermediate depth, remained ‘good’, even if a decline in the mean vitality index was recorded over time (setting up: 3.8 ± 0.2; monitoring phase: 3.5 ± 0.2) and the number of sites ranked as ‘good’ declined over course of the study period (Tables 5 and 6). This decline in vitality was observed both at the reference sites (50%) and at the high human pressure impact sites (53%).
Vitality of P. oceanica meadow at the time of the setting up of the PMN (2004–2007). Mean ± standard deviation (95%).
Setting up | Lower limit type | Lower limit depth (m) |
Cover (%) |
Plagiotropic rhizomes (%) | Leaf production (number·y−1) | Rhizome elongation (mm·y−1) | Density (shoot·m−2) |
Leaf surfacea (cm2·shoot−1) | Synthesis (Mean metric values) |
Macinaggio (ref) | P | 38.0 ± 0.1 | 21.8 ± 4.2 | 20.6 ± 9.9 | 7.1 ± 0.2 | 6.6 ± 1.6 | 243.8 ± 11.6 | 371 | 4.1 (Good) |
Cap Sagro (ref) | S + | 33.0 ± 0.3 | 13.2 ± 5.2 | 12.4 ± 9.0 | 6.3 ± 0.3 | 6.5 ± 1.5 | 270.8 ± 19.0 | 412 | 3.6 (Good) |
Toga | R | 24.2 ± 0.4 | 19.1 ± 7.1 | 8.6 ± 2.9 | 7.0 ± 0.4 | 5.5 ± 0.9 | 230.7 ± 16.2 | 348 | 2.9 (Normal) |
Arinella | S + | 26.9 ± 0.3 | 54.5 ± 11.9 | 30.9 ± 5.6 | 8.1 ± 0.1 | 5.1 ± 0.4 | 246.4 ± 10.7 | 314 | 4.0 (Good) |
Bravone | R | 36.1 ± 0.1 | 40.9 ± 7.7 | 24.1 ± 2.9 | 7.6 ± 0.4 | 8.5 ± 1.8 | 315.1 ± 17.2 | 302 | 3.9 (Good) |
Favone (ref) | Sp | 36.9 ± 0.3 | 7.7 ± 2.2 | 80.0 ± 5.3 | 6.2 ± 0.6 | 4.4 ± 1.7 | 325.0 ± 17.4 | 316 | 3.5 (Good) |
La Chiappa | S– | 35.3 ± 0.1 | 17.7 ± 5.0 | 9.3 ± 6.2 | 7.4 ± 0.6 | 9.0 ± 1.9 | 304.0 ± 13.6 | 292 | 3.9 (Good) |
Lavezzi (ref) | P | 30.3 ± 0.1 | 66.3 ± 16.7 | 17.7 ± 7.0 | 8.2 ± 0.7 | 6.6 ± 1.2 | 169.3 ± 17.2 | 345 | 3.9 (Good) |
Porto Pollo (ref) | S + | 32.2 ± 0.2 | 32.0 ± 5.6 | 94.5 ± 3.1 | 7.2 ± 0.5 | 4.3 ± 1.4 | 256.3 ± 14.7 | 303 | 4.0 (Good) |
La Parata | Sp | 35.3 ± 0.2 | 10.1 ± 4.3 | 95.5 ± 3.1 | 8.7 ± 0.8 | 6.3 ± 1.7 | 176.1 ± 7.7 | 300 | 3.8 (Good) |
Sagone (ref) | P | 33.2 ± 0.1 | 26.5 ± 12.8 | 75.5 ± 14.5 | 8.2 ± 0.4 | 6.1 ± 1.2 | 472.4 ± 21.8 | 226 | 4.4 (Good) |
Porto (ref) | S– | 36.5 ± 0.2 | 20.1 ± 8.1 | 96.2 ± 5.4 | 6.5 ± 0.4 | 4.2 ± 0.7 | 338.4 ± 16.8 | 310 | 3.8 (Good) |
Stareso (ref) | S + | 38.6 ± 0.3 | 27.6 ± 10.2 | 77.3 ± 15.0 | 7.3 ± 0.7 | 4.5 ± 1.2 | 310.4 ± 27.9 | 367 | 4.3 (Good) |
Île Rousse | R | 35.8 ± 0.2 | 33.6 ± 15.5 | 56.6 ± 17.0 | 6.5 ± 0.3 | 5.4 ± 1.0 | 208.3 ± 11.9 | 181 | 3.1 (Normal) |
Canari | S + | 27.4 ± 0.8 | 87.0 ± 6.7 | 11.7 ± 7.4 | 8.2 ± 0.5 | 7.1 ± 1.4 | 280.2 ± 14.7 | 245 | 3.9 (Good) |
a Only mean data are available.
Vitality of P. oceanica meadow during the monitoring phase (2013). Mean ± standard deviation (95%).
Control phase | Lower limit type | Lower limit depth (m) |
Cover (%) |
Plagiotropic rhizomes (%) |
Leaf production (number·y−1) | Rhizome elongation (mm·y−1) | Density (shoot·m−2) |
Leaf surface (cm2·shoot−1) | Synthesis (Mean metric values) |
Macinaggio (ref) | P | 38.0 ± 0.1 | 14.2 ± 9.2 | 78.5 ± 12.6 | 7.2 ± 0.7 | 5.2 ± 1.7 | 368.8 ± 22.0 | 307.2 ± 37.7 | 4.1 (Good) |
Cap Sagro (ref) | R | 33.0 ± 0.3 | 5.7 ± 3.7 | 90.9 ± 12.8 | 6.9 ± 0.5 | 4.0 ± 1.0 | 307.6 ± 32.2 | 304.4 ± 29.8 | 3.1 (Normal) |
Toga | R | 24.3 ± 0.4 | 41.2 ± 13.2 | 21.3 ± 13.7 | 7.0 ± 0.8 | 6.2 ± 1.7 | 268.4 ± 29.3 | 253.7 ± 37.5 | 3.3 (Normal) |
Arinella | R | 26.9 ± 0.3 | 6.8 ± 4.5 | 52.1 ± 19.0 | 7.6 ± 0.6 | 4.3 ± 0.8 | 260.4 ± 33.0 | 352.1 ± 27.9 | 3.1 (Normal) |
Bravone | R | 36.1 ± 0.1 | 28.3 ± 12.1 | 65.1 ± 12.5 | 7.0 ± 0.6 | 4.7 ± 1.1 | 308.4 ± 30.6 | 171.5 ± 21.6 | 3.4 (Normal) |
Favone (ref) | Sp | 36.9 ± 0.2 | 7.6 ± 2.6 | 81.4 ± 8.5 | 7.0 ± 0.8 | 6.2 ± 1.7 | 322.8 ± 49.7 | 188.7 ± 29.6 | 3.5 (Good) |
La Chiappa | R | 35.3 ± 0.1 | 12.5 ± 5.9 | 83.4 ± 17.2 | 6.9 ± 0.6 | 4.2 ± 0.9 | 318.0 ± 32.0 | 195.3 ± 37.8 | 3.1 (Normal) |
Lavezzi (ref) | P | 30.3 ± 0.1 | 44.0 ± 18.4 | 67.2 ± 17.4 | 8.1 ± 0.7 | 5.2 ± 1.3 | 241.6 ± 32.3 | 312.1 ± 62.4 | 4.1 (Good) |
Porto Pollo (ref) | R | 32.2 ± 0.2 | 4.8 ± 2.2 | 79.3 ± 13.6 | 7.6 ± 0.7 | 4.0 ± 1.1 | 351.2 ± 46.2 | 215.6 ± 33.1 | 3.1 (Normal) |
La Parata | R | 35.3 ± 0.2 | 1.0 ± 0.9 | 92.5 ± 10.2 | 7.9 ± 0.7 | 3.5 ± 0.8 | 211.6 ± 25.0 | 244.2 ± 36.0 | 3.0 (Normal) |
Sagone (ref) | S– | 33.2 ± 0.1 | 35.2 ± 16.0 | 67.7 ± 11.6 | 7.8 ± 0.6 | 4.9 ± 0.9 | 448.0 ± 32.3 | 106.5 ± 21.2 | 3.6 (Good) |
Porto (ref) | S– | 36.5 ± 1.3 | 23.5 ± 9.5 | 85.8 ± 15.7 | 7.0 ± 0.6 | 4.9 ± 1.4 | 298.4 ± 47.3 | 288.1 ± 38.7 | 3.8 (Good) |
Stareso (ref) | S– | 38.6 ± 0.3 | 19.2 ± 4.6 | 94.5 ± 8.0 | 7.0 ± 0.7 | 3.7 ± 0.7 | 240.8 ± 20.5 | 276.0 ± 28.3 | 3.6 (Good) |
Île Rousse | R | 35.8 ± 0.2 | 22.6 ± 12.7 | 81.5 ± 14.1 | 7.1 ± 0.7 | 3.8 ± 1.1 | 258.4 ± 25.0 | 139.5 ± 20.9 | 3.1 (Normal) |
Canari | S + | 27.4 ± 0.8 | 97.5 ± 3.5 | 28.5 ± 11.3 | 8.1 ± 0.6 | 6.3 ± 1.5 | 376.8 ± 38.1 | 387.5 ± 78.3 | 4.3 (Good) |
The quality of the water body (BiPo Index) declined over the course of the study period (setting up: 0.74 ± 0.06; monitoring phase: 0.67 ± 0.07) but also remained ‘good’ at the scale of the Corsican coasts as a whole (Tables 7 and 8). This decline was observed both for the reference sites (75%) and for the high human pressure impact sites (80%).
Water body quality (BiPo index) at PMN sites during setting up (2004–2007). Mean ± standard deviation (95%).
Setting up | Lower limit depth (m) | Lower limit type | Density (shoot·m−2) |
Leaf length (cm) | EQR |
Macinaggio (ref) | 38.0 ± 0.1 | P | 243.8±11.6 | 978.1 ± 28.5 | 0.89 (High) |
Cap Sagro (ref) | 33.0 ± 0.3 | S + | 270.8±19.0 | 989.7 ± 36.7 | 0.84 (High) |
Toga | 24.2 ± 0.4 | R | 230.7±16.2 | 853.8 ± 40.7 | 0.52 (Normal) |
Arinella | 26.9 ± 0.3 | S + | 246.4±10.7 | 913.9 ± 36.5 | 0.75 (Good) |
Bravone | 36.1 ± 0.1 | R | 315.1±17.2 | 830.8 ± 44.7 | 0.67 (Good) |
Favone (ref) | 36.9 ± 0.3 | Sp | 325.0±17.4 | 903.0 ± 30.0 | 0.77 (Good) |
La Chiappa | 35.3 ± 0.1 | S– | 304.0±13.6 | 839.3 ± 28.2 | 0.77 (Good) |
Lavezzi (ref) | 30.3 ± 0.1 | P | 169.3±17.2 | 914.7 ± 32.2 | 0.76 (Good) |
Porto Pollo (ref) | 32.2 ± 0.2 | S + | 256.3±14.7 | 730.0 ± 23.4 | 0.74 (Good) |
La Parata | 35.3 ± 0.2 | Sp | 176.1±7.7 | 732.1 ± 39.1 | 0.59 (Good) |
Sagone (ref) | 33.2 ± 0.1 | P | 472.4±21.8 | 576.7 ± 31.2 | 0.80 (High) |
Porto (ref) | 36.5 ± 0.2 | S– | 338.4±16.8 | 785.8 ± 59.4 | 0.87 (High) |
Stareso (ref) | 38.6 ± 0.3 | S + | 310.4±27.9 | 887.1 ± 18.9 | 0.87 (High) |
Île Rousse | 35.8 ± 0.2 | R | 208.3±11.9 | 810.7 ± 40.8 | 0.59 (Good) |
Canari | 27.4 ± 0.8 | S + | 280.2±14.7 | 690.8 ± 46.6 | 0.69 (Good) |
Water body quality (BiPo index) at PMN sites during the control phase (2013). Mean ± standard deviation (95%).
Control phase | Lower limit depth (m) | Lower limit type | Density (shoot·m−2) |
Leaf length (cm) | EQR |
Macinaggio (ref) | 38.0 ± 0.1 | P | 368.8 ± 22.0 | 964.5 ± 97.1 | 0.95 (High) |
Cap Sagro (ref) | 33.0 ± 0.3 | R | 307.6 ± 32.2 | 962.9 ± 53.4 | 0.69 (Good) |
Toga | 24.3 ± 0.4 | R | 268.4 ± 29.3 | 714.8 ± 74.7 | 0.49 (Normal) |
Arinella | 26.9 ± 0.3 | R | 260.4 ± 33.0 | 972.0 ± 53.4 | 0.61 (Good) |
Bravone | 36.1 ± 0.1 | R | 308.4 ± 30.6 | 612.8 ± 61.0 | 0.59 (Good) |
Favone (ref) | 36.9 ± 0.2 | Sp | 322.8 ± 49.7 | 592.2 ± 85.5 | 0.66 (Good) |
La Chiappa | 35.3 ± 0.1 | R | 318.0 ± 32.0 | 675.0 ± 122.7 | 0.61 (Good) |
Lavezzi (ref) | 30.3 ± 0.1 | P | 241.6 ± 32.3 | 990.5 ± 93.1 | 0.82 (High) |
Porto Pollo (ref) | 32.2 ± 0.2 | R | 351.2 ± 46.2 | 683.2 ± 69.5 | 0.60 (Good) |
La Parata | 35.3 ± 0.2 | R | 211.6 ± 25.0 | 715.8 ± 72.9 | 0.55 (Good) |
Sagone (ref) | 33.2 ± 0.1 | S– | 448.0 ± 32.3 | 410.7 ± 47.0 | 0.65 (Good) |
Porto (ref) | 36.5 ± 1.3 | S– | 298.4 ± 47.3 | 903.1 ± 103.8 | 0.80 (High) |
Stareso (ref) | 38.6 ± 0.3 | S– | 240.8 ± 20.5 | 634.1 ± 35.2 | 0.69 (Good) |
Île Rousse | 35.8 ± 0.2 | R | 258.4 ± 25.0 | 455.6 ± 47.8 | 0.49 (Normal) |
Canari | 27.4 ± 0.8 | S + | 376.8 ± 38.1 | 982.1 ± 122.6 | 0.83 (High) |
The three transects of the SeagrassNet system, installed at the Stareso site in 2006, were recovered at each follow-up visit to the site (2009 and 2012). The meadow density declined when the depth increased for the three years studied (Fig. 2). Between 2006 and 2012, the density increased at the upper limit (8 m depth), remained stable at the intermediate depth (25 m) and declined at the lower limit (37 m). At the lower limit, it declined from 24 ± 16 to 12 ± 13 shoots per m2.

Changes in meadow density (mean shoot number per square meter ± confidence level 95%) between 2006 and 2012 at different depths, from the upper limit to the lower one.
The meadow cover also declined at the lower limit where it dropped respectively from 23.3 ± 10.0% in 2006, to 20.0 ± 9.0% in 2009 and to 19.2 ± 12.6% in 2012
4 Discussion
The introduction of the PMN for Corsica between 2004 and 2007 constituted the first stage towards achieving a reference state for the localization and the vitality of the P. oceanica meadows along the coasts of Corsica [33]. The follow-up visit to the site in 2013 made it possible to assess the effectiveness of the protocol used and to monitor changes in the state of conservation of the meadows over time.
The protocol used for the PMN for the Corsican coasts provided a basis for:
- • validating the sustainability of the structures used (99% still found after 5 to 9 years without maintenance);
- • comparing with precision the data acquired during the setting up phase (2004–2007) and at the time of the first follow-up visit (2013);
- • detecting and quantifying the alterations recorded with regard to the lower limit, the vitality of the meadow and the quality of the water body, and;
- • comparing the data collected with those resulting from another monitoring system, the SeagrassNet system, for the Stareso site.
For the PMN system, a considerable effort was made with regard to the data acquisition protocol to limit the degree of observer subjectivity and in order to dispose of reproducible raw data. These raw data could perhaps be optimized in the years to come depending on the new tools that become available and on advances in scientific knowledge. The PMN protocol is consistent with the guidelines validated by the Barcelona Convention [15], which means that it can offer a basis for comparison with other similar networks that have been developed for the Mediterranean [10,11,17].
The SeagrassNet protocol has from the outset been standardized in a very precise way [34]; its adaptation to take into account the specificities of the Mediterranean seagrass meadows [35] will allow very effective temporal comparisons (http://www.seagrassnet.org/).
The vitality of the P. oceanica meadows and the quality of the water body (BiPo index) along the Corsican coasts, respectively “Normal” and “Good”, are strongly correlated (r = 0.86 in 2013). The “High” values recorded for lower limit depth may be explained in particular by the high degree of limpidity of the waters, because of the small number of permanent coastal rivers and the limited impact of human activities. In general, the sites situated in the highly oligotrophic sectors (e.g., Cyprus [36,37]) often present high values for the BiPo index [38] whereas sites exposed to high turbidity (e.g., Catalonia [6]), even if of natural origin, present lower values ([39]; Table 9).
Assessment of the water quality on the basis of the BiPo index at different Mediterranen sites.
Country | Site | BiPo index | Reference |
Spain | Catalonia – Montroig | 0.36 | [40] |
Catalonia – Mataro | 0.55 | [40] | |
France | PMN Corsica (2013) | 0.49–0.95 mean: 0.67 |
This study |
Corsica – Punta Bianca | 0.84 | [39] | |
Corsica – Stareso | 0.82 | [39] | |
Corsica – Cages | 0.77 | [39] | |
Italy | Ischia – Lacco Ameno | 0.59 | [40] |
Ischia – Scarrupata | 0.78 | [40] | |
Cyprus | Akamas | 0.94 | [38] |
Cavo Greko | 0.90 | [38] | |
Limassol | 0.79 | [38] | |
Moulia | 0.95 | [38] | |
Nisia | 0.87 | [38] | |
Polis | 0.82 | [38] | |
Tunisia | Sidi Ali El Mekki | 0.86 | [17] |
Kerkennah island | 0.65 | [17] | |
Turkey | Gokceadaa | 0.49 | [17] |
Mersinb | 0.34 | [17] |
a Off the Dardanelles Straight.
b Southeastern limit of the range of P. oceanica.
Concerning the PMN Corsica, no clear patterns of change over time of the P. oceanica meadows can be observed (Table 10), even if the vitality index value has slightly declined by 8.6% on average. However, taking into account changes in (i) the position of the lower limit, (ii) the vitality index and (iii) the BiPo index, the median showed a pattern of stability for 9 sites (including 5 reference sites), regression for 5 sites (including 3 reference sites), and progression for 1 site (Table 10).
Indicators dashboard – environmental status and patterns of change in the location of the lower limit, the vitality of the meadow and the water body quality (BiPo) between 2004–2007 and 2013.
Site | Lower limit (Depth) Evolution |
Vitality index (Value) Evolution |
BiPo index (Value) Evolution |
Synthesis |
Macinaggio (ref) | (High) | (Good) | (High) | (High) |
Cap Sagro (ref) | (Good) | (Normal) | (Good) | (Good) |
Toga | (Normal) | (Normal) | (Normal) | (Normal) |
Arinella | (High) | (Normal) | (Good) | (Normal) |
Bravone | (High) | (Normal) | (Good) | (Good) |
Favone (ref) | (High) | (Good) | (Good) | (Good) |
La Chiappa | (High) | (Normal) | (Good) | (Good) |
Lavezzi (ref) | (Normal) | (Good) | (High) | (Good) |
Porto Pollo (ref) | (Good) | (Normal) | (Good) | (Good) |
La Parata | (High) | (Normal) | (Good) | (Good) |
Sagone (ref) | (Good) | (Good) | (Good) | (Good) |
Porto (ref) | (High) | (Good) | (High) | (High) |
Stareso (ref) | (High) | (Good) | (Good) | (Good) |
Île Rousse | (High) | (Normal) | (Normal) | (Good) |
Canari | (Normal) | (Good) | (High) | (Good) |
Synthesis | (High) | (Normal) | (Good) | (Good) |
Concerning the reference sites, other disturbing observations underlined their regression. In terms of values, 50% presented a decline of the vitality index value (Tables 5 and 6) and 75% of the BiPo index value (Tables 7 and 8). More specifically, 25% of these sites presented a regressive lower limit type, in 2013. Moreover, the number of reference sites where the pattern of change in the location of the lower limit was “regressive” was the same as that where it was “stable” [4].
The regression recorded at the level of the lower limit of the meadows cannot be imputed to local degradation of the environmental conditions, given that they were distributed along the whole coastline of the island and in view of their distance from potential sources of human pressure. In addition, a similar phenomenon was observed for the Provence-Alpes-Côte d’Azur Region PMN (between 1988 and 1999 in [12]), and more particularly at the level of the lower limit of the Côte Bleue seagrass meadows [41].
Similarly, in the “Parc national de Port-Cros” (reference meadow), [42] observed a very severe decline in the meadow density and cover at the level of the lower limit between 2002 and 2008. A similar pattern was also observed at Kerkennah Islands (Tunisia), in the southwestern basin, between 2007 and 2014 (Pergent and Langar, unpublished data). In the Western Pacific region, in sites with other seagrass species and stable human influence, the SeagrassNet also exhibited a clear decline [43].
For the common reference site, Stareso, the two monitoring systems (PMN and SeagrassNet) show the same regressive dynamic with a decline in the meadow vitality, in particular with regard to the density and cover at the lower limit.
If P. oceanica regression could be related to the rise in temperature of the seawater at shallow depth [32,44], this seems less plausible at depths where the temperature is lower and more stable [42], even if some regression has been locally observed, along the lower limit, during warm water episodes [41]. The lower limit corresponds to the depth of compensation of the species, beyond which the amount of light is insufficient to enable photosynthetic production to compensate for the losses due to respiration [45]; any reduction of this light will inevitably entail a decline in the vitality of the meadow and a regression of the position of the lower limit [46]. Two hypotheses might explain the regression observed:
- • the mean sea level rose between 1870 and 2000 by 18 cm, including 6 cm over the past 20 years [47]. Measurements taken in Corsica, at the Ajaccio tidal gauge station, show a mean increase of 2 cm over the past 10 years (SONEL network; http://www.sonel.org/-Maregraphes-.html). For the deep lower limits (below 35 m depth), this increase in the height of the water column over the past decade has resulted in a decline in the amount of light at the seabed. This reduction may result, in areas where the slope is relatively slight, in a significant regression of the position of the lower limit [21]. Thus, for a slope of 2%, an increase of 2 cm in the depth of the water column (vertical) corresponds to a linear regression of 1 m of the position of the lower limit (horizontal) of the seagrass meadow (Fig. 3);
- • the North Atlantic Oscillation (NAO) may play a role in the penetration of the light within the water column. This climatic oscillation (NAO) may entail, when it is slight, an increase in precipitation (increase in inflow from coastal rivers and cloud cover) at Mediterranean scale [48]. On the basis of the high values during the 1990s, the NAO index declined after 2002 to reach very low values in 2010 [49,50].
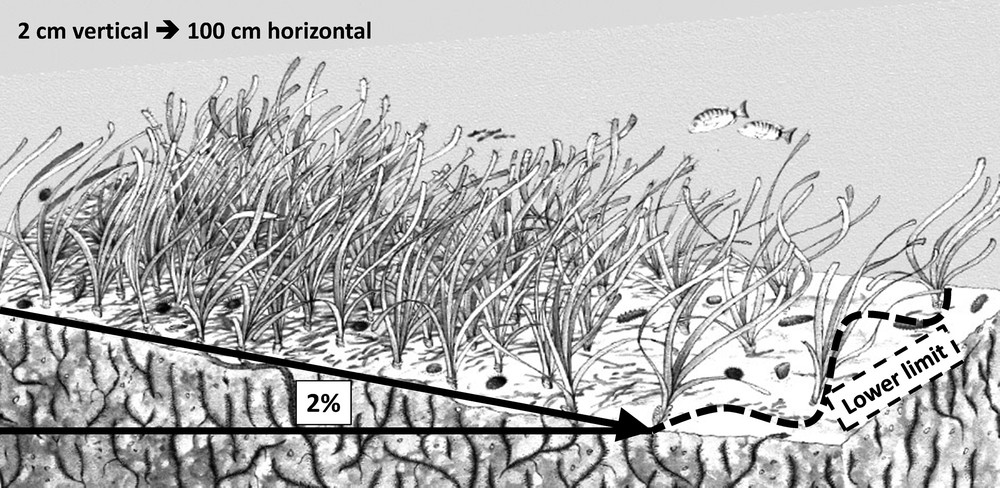
Regression of the lower limit resulting from increase of water column height and mean slope.
The sites exposed to human pressure present a more contrasting dynamic. For the sites exposed to the persistence, or even the amplification, of these pressures, the state of conservation of the meadows (vitality index) continues to decline. This is the case for the sites of Arinella (urban sewage outfall) and La Parata (discharges from an aquaculture facility). In contrast, at sites where these pressures are in decline, the state of conservation of the meadow has stabilized (Toga: shutdown of coastal management operations, Île Rousse: establishment of a sewage treatment plant) or progressed (Canari: shutdown of mining discharges at sea).
It is thus clear that the state of conservation of the P. oceanica meadow, as a bioindicator, constitutes an effective tool for the assessment of government policy regarding actions undertaken to improve the quality of the environment. The PMN put into application along the coasts of Corsica would appear to be highly sensitive to the degradations or improvements in the environmental conditions. In addition, if the regression of the lower limit of the meadow, at several reference sites, in relation with the rise in sea level, is confirmed, this species may also play the role of bioindicator for climate change in the Mediterranean. Climate change might be the cause of a significant regression of the lower limit of the P. oceanica meadows, at Mediterranean scale, during the course of the 21th century, given the forecasts for the patterns of change in sea level. [51]. For this reason, an extension of the PMN in Corsica, with the installation of six new reference sites in the “Réserve naturelle des bouches de Bonifacio” (southern coast), was undertaken in 2013. These new sites should provide the means to monitor more precisely the patterns of change in the seagrass meadow where human impact is low and could definitively confirm the impact of climate change.
Disclosure of interest
The authors declare that they have no conflicts of interest concerning this article.
Acknowledgments
This work was supported by a grant from the “Office de l’Environnement de Corse” (Corsica, France) and received support from the Global Seagrass Monitoring Network. It was carried out within the framework of the programme CHANGE of the FRES 3041, funded by the “Collectivité Territoriale de Corse” (Corsica, France). The authors thank Aurélie Blanfuné-Thibaut for statistics and Michael Paul for checking the English. The authors would also like to express their gratitude to the reviewers for their helpful remarks.