1 Introduction
In his famous book, Chance and Necessity, Jacques Monod reminded us that telling living things from non-living ones is straightforward. Even when in fossil form, the remains of life display some sort of arbitrariness, some sort of inventive property, which the standard laws of mechanical physics would not reach easily. It may therefore seem outrageously naïve to take the stance of the engineer, and design and construct from scratch a living organism. Yet, this prompted the endeavour of Synthetic Biology, which seeks to develop the building up of living contraptions, keeping biology within the realm of physics and chemistry. To be sure, for a long time it was common practice to see life in fairly standard chemical processes such as those illustrated by Stéphane Leduc in his 1912 book, La biologie synthétique (Fig. 1).
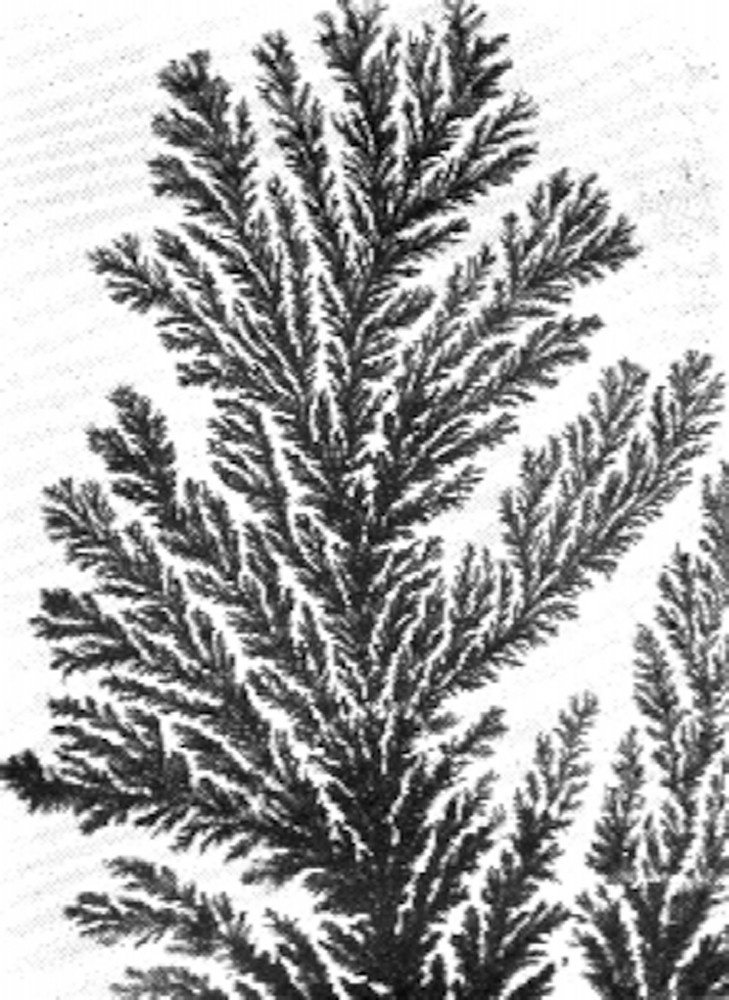
Silver sediments on a punctiform cathode, during the electrolysis of silver nitrate (Stéphane Leduc, La Biologie synthétique, http://www.peiresc.org/bs08suite.htm).
2 From nature to manufacture: combining genes to make a living organism
All the same, for the general public a synthetic drug produced by engineering is believed to carry the scary flavour of craftsmanship. The identical chemical, isolated from a plant or an animal, would carry the harmless and even beneficial flavour of Nature. What is deemed natural is somehow blessed with a spark of life. As often, this common sense has something important to tell us, something still escaping attention of most biologists. Indeed, the common view perceives the laws of physics, as they were three centuries ago, tantamount to those of mechanics, while it seems obvious that living organisms differ from mechanical constructs. This implies that the recent revolutions in physics, that I will now outline, has not reached yet the understanding of biologists nor, a fortiori, that of the general public.
Some 10,000 years ago, in parallel with the emergence of complex mechanical tools, the Neolithic revolution created agriculture, based on man-mediated selection of plants and animals. Domestication retained useful traits and directed their evolution towards organisms useful to us. First, implicitly, then explicitly in recent times, we used in this process our knowledge of heredity to speed up the efficiency of selection. Domestication became intimately connected to what was to become genetics. A dramatic change between the old recipes meant to domesticate life and the science of genetics happened after the discovery of genes by Mendel—whom we celebrate today, De Vries, Correns, von Tschermak, Johannsen, Morgan, Sturtevant, Ephrussi, L’Héritier and many others. Knowing that the characters we could observe resulted from a combinatorial action of genes—that were organised as linear sequences and subject to shuffling between varieties or even species—a first gene-driven approach resulted in a considerable speed up of the creation of new varieties for agriculture. This gene/character-driven process is still a most widely used way to domesticate plants and animals.
The process was refined by increasing human intrusion via running statistical tools that give marks to phenotypic traits and connect them to the way genes are organised in the genome. Let us notice that this procedure is obviously artificial, because someone, and not blind nature itself, selects individuals to force them into the generation of a hopeful progeny, often using manual fertilisation. Yet the practice is generally appreciated as natural in the coverage of mass media. This is likely because it combines one or more fully living organisms to get the expected progeny. The spark of life is still there. By contrast with this apparently holistic approach, genetic engineering, the most recent step in this process of gene-driven domestication of plants and animals (genetically modified organisms, GMOs), uses bits and pieces of living things (or even synthetic constructs) to produce an organism with the desired properties. Lacking knowledge about the way life develops, the general public perceives this designed fragmentation as missing an essential attribute of life, and, as a consequence, as possibly dangerous. Yes, this mistaken feeling misses a major process of gene invention in nature: the natural evolution of organisms across generations also uses bits and pieces of living things, and this at a huge scale, much larger than what man could do. Indeed, despite human ingenuity, the GMO approach falls short of the way life itself explores the infinite space of gene variation. All the same, and contradicting this flawed belief, the danger would in fact take place because the construction is alive, not inert, showing implicitly that we perceive nature as potentially dangerous.
3 Information and life
To better understand the borders of natural and synthetic life we need to dive deeper in the intricacies of the cell's functions. This will lead us into a profound question of physics—and perhaps philosophy, that of the nature of the physical currencies that build up reality. Genetics, at the core of the science of heredity, is based on an alphabetic metaphor. Genetic heredity is transmitted from generation to generation by DNA, a macromolecule that has the strange property of behaving as a textbook written with an alphabet of four letters. This creates a link between genetics and number theory that deserves further explanation, as this highlights a major feature of biology, which crept quietly into physics during the past century or so and bloomed in recent times. Alphabetic writing uses strings of symbols chosen from a narrow list (usually of the order of 20-30 symbols). It combines them sequentially to represent the phonemes that make spoken words. There is no connection between these letters and the objects they represent: four (in English), cztery (in Polish), vier (in German), quatre (in French), τɛσσɛρα (in Greek), 四 (in Chinese) represent the same concept of number theory, number 4. We find here the first clue of the existence of a new currency of reality, which obviously differs from the commonly accepted ones, matter, energy, space or time. This currency underlies what we mean by “symbol”, the fact that some entity is used to represent an abstract (yet physically real) idea. Commonly referred to as “information”, it embodies the link between the symbol and what it represents. Now, DNA is made of the chaining of four chemicals, its sequence, following an order that has something to do with the general functioning of the cell. As just pointed out, this connects the DNA sequence with some information. Briefly, the DNA contained in a cell, its genome, can be understood as standing for a book of recipes, that which tells the cell how to behave in a given environment (in particular how to produce a progeny).
Beside its genome, the bulk of the cell behaves as a computer that would read and write (some of) the DNA text (the genetic program) according to cues provided by the environment, and interprets it as actions. It chains the recipes in an order that allows the organism to maintain itself, produce a young progeny, react to environmental cues, protect itself against aggressions, etc. The program prescribes synthesis of the entities that express its meaning. In particular it codes for the synthesis of the nanomachines that make identical copies of the program. Alan Turing in the 1930s proposed the design of a most elementary machine of this type, made of a read/write head and of a mobile tape that carried a linear sequence of symbols. Using a coding table, the machine reads or writes symbols on the tape as a function of its previous readings and moves it through the head, controlling a mechanical device that allows the tape to go forward, backward, and possibly stop. Core features of this machine, shared with living cells, is that it entails physical separation between the machine proper (the read/write head and the mechanics needed to make the tape move) and the data and program, using a coding table to set the conditions under which the program is run. With this table, the genetic program is recursive: it codes for the components that make it run. Now, Turing and others showed that running a recursive program via a coding table has a remarkable property: not only does it preclude absolute predictability in the long run, but, as demonstrated by Rolf Landauer, it also allows creation of information. To be sure, this feature of the genetic program and its coding properties is at the heart of the creative properties of life [1,2]. It is the place where information combines with matter, energy, space and time within living organisms.
A sequence of symbols associated with a coding process opens up the possibility for true creation (i.e. sudden appearance of an entity which cannot be predicted from what was existing before, but only accounted for a posteriori). Recursive rewriting of the DNA text via using a coding table makes living organisms creative, evolving in a way that cannot be fully predicted because of the very way they are constructed. This explains why living organisms may behave as information traps. This way, evolution creates new functions, which, to come into being, recruit pre-existing structures. This happens without any grand design, but locally: in a system that keeps discarding unproductive entities, if something works (locally, not necessarily to the benefit of the organism) it will be kept for further use. This process is what we name natural selection. It readily explains the “tinkering” features of living organisms (fairly haphazard collection of local functions, that concur to allow the organism, with its basic element, the cell, to thrive), as stressed by François Jacob. To state this again, living organisms are those material systems that have found a way, facing an uncertain future, to create an innovative, hence unforeseeable progeny, among which some will be able to survive. Needless to say, this applies to any type of organisms, be it natural or artificial and we must now consider how this works in practice.
4 The making of genomes
The previous, fairly abstract, view of what life is leads us to a concrete question: how are genomes produced in nature? To compare nature and craft we need to have an idea of the way they are made. An organism comes from a parent organism, which comes from a parent organism… for many generations. This chain of events requires a start, likely to be fuzzy, something like 3.5 billion years ago. A great many scenarios account for the way the first cells appeared on Earth. They all have in common that they ended in gathering together just a few hundred essential functions. These functions are enabled by macromolecular structures housed in the space enclosed by the membrane of a cell. They govern the overall management of matter and energy supplies to make a progeny similar to what the parent cell was, maintain the cell for a significant span of time, while interacting with its environment. They replicate the program (make an exact copy), while reproducing the cell (making a similar copy). In this process innovation comes from the relentless variations in the cell setup (when the program is run, it produces various outcomes depending on the environment, time or random events) and from rare accidents—mutations—in the program, that are subsequently propagated in the following generations. This allows organisms to recruit information from their environment and evolve. However, if it were solely resulting from these alterations, the speed of evolution would be extremely slow, because the program's replication is quite accurate. Furthermore this would not leave much room for innovation, which must come from the context (Fig. 2).

A scenario for the origin of the first cells builds on the critical need for compartmentalization at the origin of life. In a first step, this was assumed by the surface of minerals, which selected the reactive compounds that formed primitive metabolism. Subsequently, RNA molecules replaced mineral surfaces after the discovery of nitrogen fixation and the emergence of ribonucleotides, in parallel with a machinery for the synthesis of peptides, coenzymes, and lipids. This RNA-metabolism world then developed into an RNA-genome world based on RNA as informational templates rather than substrates. Bordered by lipids, the first cells were phagocytes, Protokarya, which put together two compartments stemming from the RNA-metabolism world (the cytoplasm) and the RNA-genome world (the nucleus). The emergence of stable deoxyribonucleotides allowed the clustering together of genes into chromosomes, while phagocytosis created the opportunity for an escape based on an alternative metabolism of membrane lipids, with the Archaea, and on the emergence of a robust and phagocyte-resistant envelope, with the Bacteria. Reductive evolution allowed bacteria with a modified enveloped to be phagocytosed again as symbionts of Protokarya, leading to the final generation of the Eukarya.
Again, a solution to this challenge is manifest in scenarios of the way the first cells emerged, creating and propagating innovation. Cells used to, and often still do fuse and split continually. They also swallowed all or pieces of other cells. Furthermore, most components of cells are fairly labile, and are broken down into small molecules. This comes true for almost everything, except for some structures, such as the ligno-cellulosic material that protects plant cells and, notably, DNA, the material of the genetic program. The recent deciphering of the genome sequence of Neanderthals and of many ancient organisms shows that DNA is a remarkably stable molecule [3]. A great many systems, viruses in particular, carry over the process and spread it as fairly large pieces across cells and organisms. DNA fragments are prone to recombination events, cutting and pasting all kinds of genetic sequences in the genome texts. Thus, through horizontal gene transfer, the coding properties of DNA are propagated over many organisms in a single generation.
The techniques designed by scientists at the origin of GMOs and synthetic biology took advantage of these pre-existing systems. Yet, this developed at a minute scale as compared to what happens in nature. Water and soil are replete with viruses and sheer DNA sequences. Typically seawater contains ten to one hundred million virus-like particles (VLP) per gram in pelagic environments and ten times this number per gram in sediments. We are bathing in a DNA soup [4]. The DNA flow can involve direct DNA transformation, but specialised processes such as those mediating bacterial sexuality, or viral infection followed by viral genome insertion or retrotransposition are ubiquitous. All kinds of viruses and mobile elements have repeatedly invaded genomes. Our own genome hosts almost 10% of its DNA derived from viruses. Viruses embedded in genomes can excise imprecisely, carrying with them host adjacent genes. As a consequence, natural genomes are patchworks of genes that display a huge combinatorial association, ever experimenting novel setups, some of which will be stabilised by natural selection.
However, the haphazard combinations thus generated have no reason to be of interest for human endeavours. To domesticate life, nature had to be replaced by craft. Slowly evolving from past pursuits, the present practice of agriculture used selection acting on the expressed traits of the organism, its phenotype, or directly on gene function in the rare situation when a precise phenotype could be solely linked to a particular gene. In its early days, selection recognised a particular trait and inbred organisms that possessed the same trait. This resulted in very slow vertical transmission, especially when using the phenotype alone (one had to wait for many generations to create a really inbred strain, and, for plants, this usually required one year per generation). Furthermore, the genes of interest hitchhiked other genes, possibly deleterious, in a fairly uncontrolled way. More recently, knowledge of the way genes are organised in the genome was used to speed up the process, via statistically-relevant choices in crosses. However, it was still not possible to know exactly what global modifications of the genome text was at work. A further skill was developed with GMOs, where the modification of the genome is considerably smaller than in artificial breeding crosses, and known exactly. Instead of selecting, after the fact, the organisms of interest, it became possible to create them with the narrow properties we wished them to have. In order to isolate a plant resistant to a virus one could either crossbreed plants (in an intelligent way, using a parent organism known to have developed some kind of resistance) until we found one that resists, or we could identify genes coding for products making viruses innocuous. The former is a considerable extension of an old practice, hybridization. However, hybrids combine whole genomes, possibly and eventually creating new species, while in genetic engineering only one gene or a few genes are introduced in the organism, which will seldom alter its nature as a species. This situation is now changing with synthetic biology, where new species will be created [5]. However, because they evolved for more than 3 billion years, natural organisms are much better at producing innovations than the crippled life we designed. Indeed, understanding how nature manages information, as we have seen, synthetic biology engineers exclude the components of their constructs that are meant to innovate. They have to: would you fly in a plane that can modify its wings and engines while flying? The danger comes when they duplicate nature, not when they create artificial organisms.
5 Nature's reach
The main difference between nature and craft is that, in the former, there is no design. In natural organisms, the processes that manage construction and maintenance are in-built, not managed by an outside intelligence. This has the consequence that living organisms must both get energy and matter exploring their environment, and be able to separate between what is old and what is young within them to generate a young progeny. This latter scoring and cherry-picking function is original to life. It belongs to the family of functions linked to information that we discussed previously. It marks where nature differs the most from manufacture. Let us first return to exploration. In natural environments this entails winning competition with other organisms. This is not so with synthetic biology organisms meant to be cell factories: their environment is designed by engineers, so that they do not require means to manage competition with foreign organisms. As for the formation of a young hopeful progeny, the synthetic biology engineers must prevent cell factories to innovate as much as possible. The consequence is that natural organisms are essentially inventive whereas artificial ones are not.
The distance between natural and artificial evolution is witnessed in the historical pattern of emerging conflicts between species. After all, competition for limited resources is the basis of natural selection. Indiscriminate continual elimination of individuals (be it only because they age), makes the background of natural selection. However, this selection is organised so as to preserve all cells carrying functions that do not draw too much on their overall competence, favouring those that lead to capture or store important resources in the presence of competitors. A major consequence of this in-built property of natural organisms is that wild-type animals or plants that get to biotopes where no competition has yet evolved, either because of climate changes, pollution, or accidental introduction, cause destructive biological invasions (islands or continents such as Australia witness invasion by foreign plants and animals with dramatic consequences) (Fig. 3).
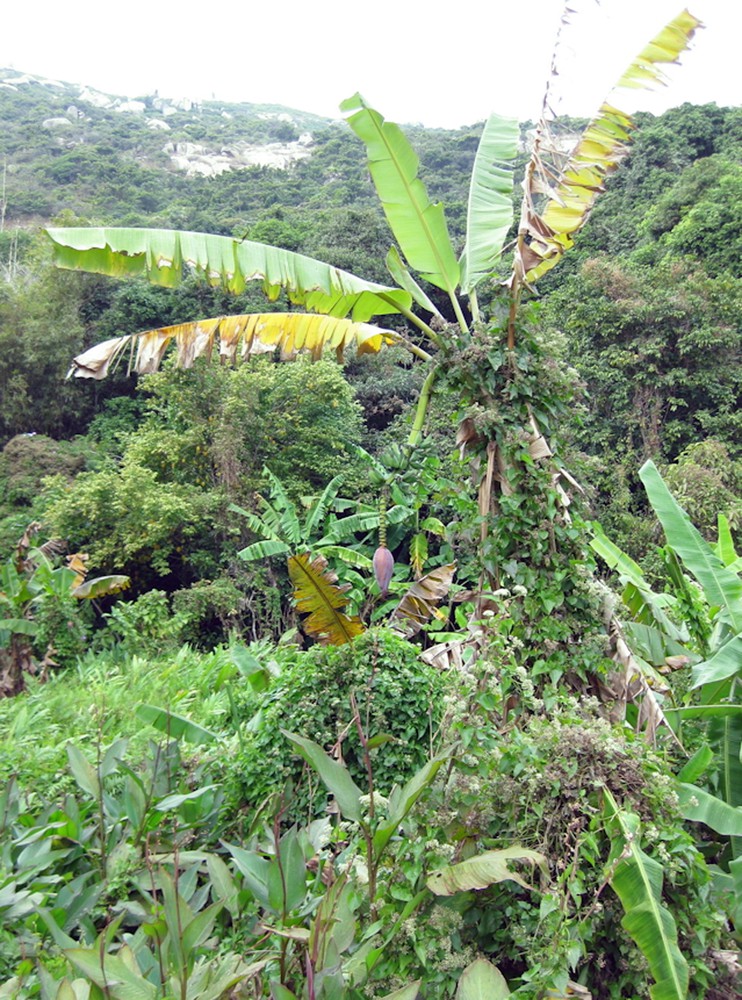
Mikania micrantha killing a banana tree (Lamma Island, Hong Kong).
Why are organisms invasive, while they are put in check in their normal environment? A cautionary example will tell. Mammalian cells harbour a variety of viruses. Over myriads of generations they became attenuated in their normal hosts where specific adaptive genome immunity was progressively built up. These viruses (see those causing many children diseases) usually cause a mild form of disease. In contrast, when shifted from their hosts to human beings, the probability that they generate very infectious agents is high because, while the entry within cells has been preserved, no adaptive immune system has yet evolved. In Archaea and Bacteria, the CRISPR system, an anti-viral immune system discovered twenty years ago and first considered anecdotal, illustrates the point. It leaves in the host genome scars of previous infections, which are subsequently used to fight further infections. Sadly, in animals, direct contact with fresh blood of related animals is dangerous because similar protecting barriers are often absent. Owing to our similarity to other Primates, a likely origin of the AIDS virus is in the butchering of monkeys or apes. However, because a pathogen requires a conserved port of entry, the danger decreases when one shifts from man to animals, which are less and less similar to us. Note that it remains significant with bats, that some think are genetically quite close to Primates [6].
A rational behaviour would be, therefore, to take care of the possibly dangerous properties of nature in an inverse proportion with the kinship, which associates us with the species of interest. Plants would be less dangerous than animals. In this respect, the fear of Genetically Modified Plants (at least when they do not carry animal genes) is unfounded. Now, monkeys and even pigs are built on a pattern very similar to that of human beings. Their organs would easily exchange with ours, triggering a keen interest for xenotransplantation, a practice that removes the species barrier. Alas, the transplanted patients would carry for years organs loaded with animal virus remnants. This would increase immensely the risk of recombination between the animal viruses and normal host endogenous viruses, the more so if the phylogenetic distance is shorter. This would create a new “experimental” field for new pathogens to emerge. The danger becomes serious when we note that xenotransplantation is perceived as positive: there is an inverse relationship between the reality of a danger and human perception of danger. If not carefully explored, such a practice, perhaps useful from the point of view of a patient, would become extremely dangerous for society as a whole. Studies are wanting in this domain. For example, do we have indications that butchering animals may be a source of diseases? The answer is positive unfortunately [7], and this would deserve thorough studies.
6 Tentative conclusions: two kinds of craftsmanship, Homo sapiens is an invasive species
Belonging to the realm of physics, the key property of life is that it is based on natural selection, a law that results in the systematic collection and subsequent transmission of novel information in the progeny of living organisms. In this context, nature is considerably more liable than craft to produce dangerous outcomes, such as what we see in constantly emerging diseases. It makes natural life in a natural environment utterly unpredictable in the long run. However this property requires that nature and craft develop in a natural environment and this situation is on the verge of a complete turnaround, because of the impact of our species on Earth. The accidental fusion of two chromosomes in one of our ancestors (note that this is a gross chromosome anomaly, that our designed modern practice of artificial human fertilisation might have prevented from producing a progeny) probably resulted in the Homo lineage, with a remarkable consequence in terms of invasiveness. Homo sapiens created a field of crafts that we did not discuss yet, crafts which aim at wholly changing its environment, the whole surface of the Earth, not at creating or modifying life directly. A first step developed with the making of tools, the control of fire, and finally with the practice of agriculture. This meant changing the environment so that plants and animals chosen by man would thrive, at the expense of wild plants and animals that were suddenly deprived of the environment that had allowed their birth, evolution and development. We also began to construct homes, cities, roads. Our fields are artificial, metamorphosing landscapes. As a consequence the human population started to increase. In turn this increase resulted in more artificial modification of the surface of our planet to the extent that, in practice, nothing on Earth remains that escaped the influence of man's craft. Nature is still inventive, but it gets less and less room to exert its inventivity. It is not the human design of living artefacts that may become dangerous, but the inanimate craft associated with our frighteningly explosive demography that destroys all natural niches where life could exert its inventivity.
Disclosure of interest
The author declares that he has no competing interest.
Acknowledgements
This work benefited from the support of AMAbiotics SAS.