1 Introduction
Many biotic and abiotic stresses hamper the production of economically important crops. Among biotic stresses, specifically, plant pathogenic fungi are the major production constraint. Rhizoctonia solani Kuhn (teleomorph: Thanetophorus cucumeris) is considered a major soil-borne plant pathogenic fungus that causes annual crop-yield losses (20–40%) worldwide [1]. Rhizoctonia solani (R. solani) is an aggressive basidiomycete necrotrophic plant pathogen having a broad host range. It forms highly resistant structures and is a major source of the infection called sclerotia (1–3 mm in diameter) that allows R. solani to survive under severe environmental conditions.
R. solani causes severe diseases in various plants, including solanaceous crops, cereals, vegetables (bean, sugar beet, lettuce), cotton, melon, ornamental plants, and forest trees [2].
Till now, 14 anastomosis groups (AG-1 to AG-13 and AG-BI12–15) of R. solani have been classified [3,4]. The transcriptomics and comparative genomics approaches are currently being exploited to understand the pathogenicity attributes of R. solani. The draft sequences of some of the anastomosis groups (AGs) of R. solani (AG1-IA AG1-IB, AG3, and AG8) are now available [2,5,6].
R. solaniis is currently controlled by various measures, including agronomic practices (crop rotation), biological agents, use of resistant cultivars and mostly of chemical agents (fungicides) [7–11]. Using resistant cultivars is considered as the most efficient approach in managing R. solani; however, developing such cultivars needs time and also new races of the pathogen appear, which overcome the resistant genes of newly developed cultivars [8].
On the other hand, the chemical agents highly pollute the environment, resulting in food security issues, high costs and also have health concerns for other organisms [12]. Even though these chemicals are easily utilizable and display high effectiveness, they cause significant pollution in reservoirs, i.e. ground and soil water reservoirs. These also accumulate as undesirable chemical residues in the food chain [13,14]. Moreover, with the excessive, persistent and abusive applications of chemical-agent-resistant strains of plants, pathogens have also emerged [15]. The advantages and disadvantages of chemical pesticides and bio-pesticides are summarized in Fig. 1.
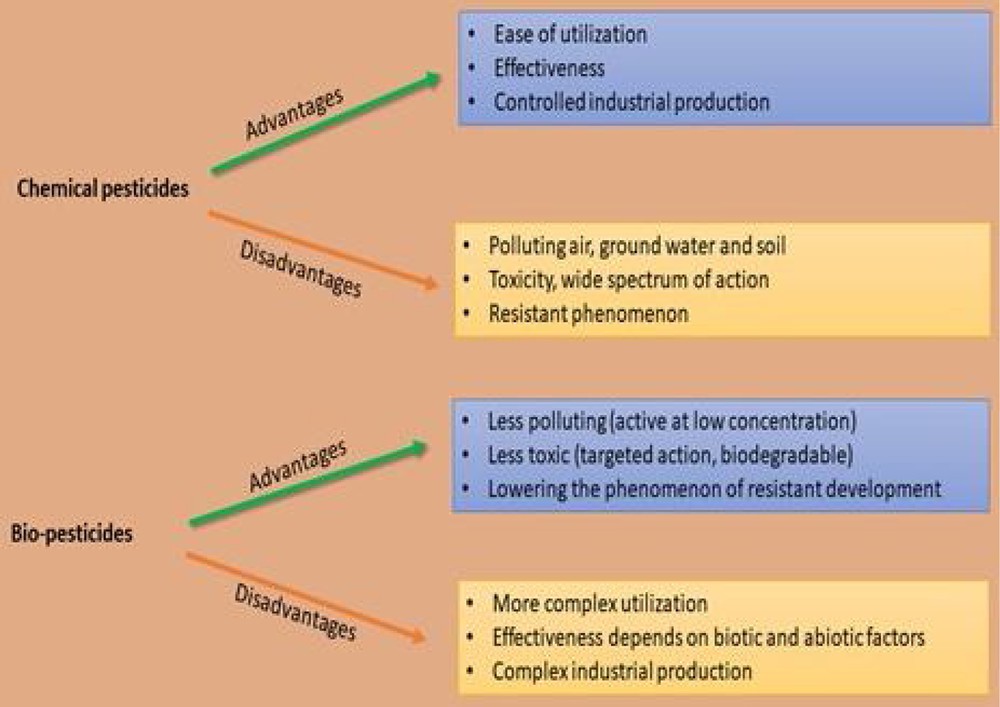
The advantages and disadvantages of chemical pesticides and bio-pesticides.
The spraying of chemical agents such as fungicides reduce the symptoms of diseases caused by R. solani, but it does not kill the sclerotia [16]. The usage of microbial biocontrol agents and of plant-growth-promoting rhizobacteria (PGPR) is considered as safer potential sustainable measures to suppress R. solani [17]. Some Bacillus spp.-based substances [1,18], active against plant pathogens, are listed in Table 1.
Bacillus spp.-based active substances against plant pathogens.
Substance | Category | List (*) | Status under reg. (EC) No 1107/2009 | Date of approval | Expiration of approval |
Bacillus amyloliquefaciens MBI 600 | FU | C | Pending | ||
Bacillus amyloliquefaciens strain FZB24 | FU | C | Pending | ||
Bacillus amyloliquefaciens sub sp. plantarum D747 | FU | C | Approved | 01/04/2015 | 31/03/2025 |
Bacillus firmus I-1582 | NE | C | Approved | 01/10/2013 | 30/09/2023 |
Bacillus pumilus QST 2808 | FU | C | Approved | 01/09/2014 | 31/08/2024 |
Bacillus subtilis str. QST 713 | BA, FU | C | Approved | 01/02/2007 | 30/04/2018 |
The application of naturally occurring beneficial microbial bio-control agents is considered as an environmentally sound option to manage diseases as well as to increase crop yield. These biocontrol agents play an essential role in the development of biopesticides [19–22]. These microbial biocontrol agents inhibit the phytopathogen growth and the development by secreting antibiotics, parasitize phytopathogens, induce systemic acquired resistance (SAR) in the host and compete with the phytopathogen for resources [23,24]. Till now, biocontrol agents such as Hypericumgramineum, Bacillus spp., Pseudomonas fluorescens, some species of Streptomyces, Ulocladiumatrum spp. and Trichoderma spp. are considered as promising biocontrol agents against phytopathogens. Among these microbial biocontrol agents, various Bacillus spp. of the Bacillus genus (members of the genus Bacillus) have become one of the attractive and effective microbial control agents that suppress R. solani both in vitro and vivo.
Bacillus spp. has become an attractive substance because it produces highly resistant structures called endospores that enable it to survive under severe environmental conditions. Bacillus spp. promotes plant growth and induces systemic resistance and becomes antagonists by secreting various types of antimicrobial compounds [25], such as lipopeptides, antibiotics (see Table 2), enzymes, and volatile organic compounds (VOCs) [25] (see Table 3) and also compete with R solani for space and nutrients [26]. Bacillus spp.-based bio-pesticides commercialized for the management of R. solani are reported in Table 4.
Antimicrobial compounds of Bacillus spp. and their corresponding functions.
Bacillus spp. strain | Antimicrobial compounds | Functions | References |
B. polymyxa VLB16 | Antifungal protein | Alter morphology of fungal hyphae | [27] |
B. amyloliquefacien LBM 5006 | Iturin-like and fengycin-like peptides | Abnormal germ tube formation and conidial germination | [28,29] |
B. amyloliquefacien GA1 | Plantazolicin | Inhibition of R. solani by the production of plant growth stimulating hormones and small molecules | [30] |
B. subtilis F-29-3 | Fengycin | R. solani is inhibited by the production of sterols, phospholipids and oleic acid | [31] |
B. subtilis B29 | Protein-B29 | Reduction of mycelial growth | [32] |
B. subtilis B-916 | Bacisubin | Inhibition of mycelial growth | [33] |
B. amyloliquefaciens WH1 | WH1fungin | Inhibition of glucans Synthase |
[34] |
Antimicrobial compounds and their functions produced by Bacillus spp. strains against R. solani.
Antibiotics | Functions |
lipopeptides | |
Surfactin | Production of biofilms, induces systemic resistance in plants |
Bacillomycin D | Direct suppression of fungi, induces systemic resistance in plants |
Fengycin | Direct suppression of fungi, induces systemic resistance in plants |
Bacillibactin | Production of siderophores |
polyketides | |
Macrolactin | Direct suppression |
Bacillaene | Direct suppression |
Difficidin | Direct suppression |
Bacilysin | Direct suppression |
Bacteriocins | |
Plantazolicin | Direct suppression |
Amylocyclicin | Direct suppression |
Volatile organic compounds | |
Acetoin/2,3-butanediol | Induced systemic resistant in plants |
Bacillus spp. based bio-pesticides commercialized for the management of R. solani.
Product name | Bio-control agent | Crop | Company | Commercialized in |
Rhapsody® | B. subtilis | Turf, forest, ornamental plants | AgraQuest Inc., USA |
USA, Canada |
Rhizo Plus® | B. subtilis FZB24 |
Several crops | ABiTEP GmbH, Germany |
Germany |
RhizoVital®42 li and RhizoVital 42TB | B. amyloliquefaciens | Vegetables, ornamental plant | ABiTEP GmbH, Germany |
Germany |
Serenade® | B. subtilis/antibiosis | Fruits, Vegetables | AgraQuest Inc., USA |
USA, Europe, South America |
Sonata® | B. pumilus | Vegetables, Fruits | AgraQuest Inc., USA |
USA, Mexico, Europe |
Sublic® | Bacillus sp. | Several crops | ELEP Biotechnologies, Italy |
Italy |
Yield Shield® | B. pumilus | Soybean | Bayer CropScience, USA |
USA |
Botrycid® | B. cepacia | Several crops | Safer Agrobiologicos Colombia |
Colombia |
Recently, Zhou et al. [35] reported a novel protein (GltB) recovered from B. subtilis Bs916 strain. When the gene responsible for this protein was mutated (gltB) through homologous recombination, it caused fragile, thin, and structurally different biofilms from that of the wild-type B. subtilis strain. Furthermore, the mutant produced a structurally different γ-polyglutamate (γ-PGA) and three lipopeptide antibiotics (LPs). When this mutant was used as a biocontrol agent against rice sheath blight disease caused by R. solani, it exhibited no biocontrol activity. This study examined the importance of GltB on biofilm formation and the biocontrol efficiency of B. subtilis Bs916 against R. solani.
Bacillus subtilis strain NCD-2 reduced the mycelia growth of Verticillium dahliae Kleb and Rhizoctonia solani in dual assays. The NCD-2 strain gene phoR gene was mutated mini-Tn10, and the mutant strain was designated as M216. The mutant failed to suppress the growth of both V. dahliae and R. solani. The mutated gene phoR gene encodes an enzyme kinase in the PhoP/PhoR two-component system. The ATPase activities, as well as growth on organic phosphate agar, were also reduced in the mutant strain of Bacillus subtilis. Furthermore, the mutant lost its phosphatidylcholine-solubilizing ability. When the cotton seedlings were treated with the mutant to manage to damp off in vivo, it was found that the mutant failed to suppress the damping off of cotton. Moreover, the colonization of the rhizosphere of cotton by the mutant also decreased as compared to the wild strains of Bacillus subtilis. In summary, the antagonistic activities of B. subtilisNCD-2 against V. dhaliae and R. solani are under the influence of a PhoR/PhoP two-component system. Further research is needed to know what secondary metabolites or antibiotics of NCD-2 strain are controlled by the PhoR/PhoP system [36]. In this review paper, we report about the bio-control attributes and mode of action and spectrum of compounds released by Bacillus spp. against R. solani as well as about the management of major diseases caused by R. solani by Bacillus spp.
2 Plant growth promoters
The bacteria found in the rhizosphere and in roots of plants have multiple functions. Some of them can cause severe disease and others enhance plant growth and control diseases. The bacteria that enhance plant growth and also protect plants from diseases are technically called plant-growth-promoting bacteria (PGPB) [37–40]. These bacteria are further categorized based on the location, i.e. some either colonize the rhizosphere or endosphere of different root parts and some colonize both. Those bacteria that are living in the rhizosphere are known as rhizobacteria and, as they are promoting the growth of plan, they are technically called plant-growth-promoting rhizobacteria (PGPR) [40]. Similarly, the bacteria found in the endosphere of plants are known as endophytes. The members of the Bacillus species are important plant-growth-promoting bacteria (PGPB) that protect plants from biotic as well as abiotic stresses [20]. Therefore, strains of Bacillus spp. have been used as bio-fertilizers as well as alternative biocontrol agents in place of chemical fertilizers and pesticides, respectively [19,20]. Similarly, some members of the Bacillus spp. family act as endophytes. These endophytes do not cause any harm to plants; however, they are also protecting the plants from other phytopathogens and environmental stresses. They enhance soil porosity for nodule formation; as a result, the growth of plants is promoted. Bacillus spp. solubilized minerals like potassium (K), zinc, and phosphorous (P) from the soil, and increased porosity. They also produce hormones like indole-3-acetic acid (IAA), hydrolytic enzymes, antibiotics, volatile organic compounds (VOCs), lipopeptides, and siderophores [26,40–46]. All these structures and compounds are associated with the biocontrol activity of Bacillus spp. against plant pathogens. Similarly, plants roots secrete nutrients and mucilage to the rhizosphere and, due to the availability of nutrient microorganisms, find a competitive environment. PGPRs such as Bacillus spp. reside within or are surrounding plant roots and assist in the growth and development of the plants [47]. As mentioned above, these PGPR (Bacillus spp.) are actually found in the soil that surrounds and interact with plant roots and inhibits plant diseases by producing reactive oxygen species (ROS), hydrogen peroxide, and volatile compounds [48,49].
Specific strains of PGPRs have initially been demonstrated against R. solani by Fatima et al. [50]. Now the application of PGPRs against phytopathogens is growing rapidly [51] and could reach five billion dollars by 2020 [52]. PGPRs suppress soil-borne pathogens like R. solani and, as a result, the growth of the plant is accelerated. Moreover, they produce antibiotics as well as they induce in the plants systemic resistance against plant pathogens. Some members of the Bacillus spp. family, acting as PGPR, increase the yield as well as they suppress soil-borne plant pathogens. Bacillus amyloliquefaciencs is a Gram-positive bacterium that is commercially available as a bio-fertilizer. Genome analysis revealed that it can also act as a biocontrol agent because 10% of the genome is involved in the production of antimicrobial metabolites [18].
An endophytic strain was isolated from the rhizosphere of rapeseeds. The objective was to check its biocontrol activity against R. solani as well as its plant-growth-promoting ability. The strain was identified as Bacillus subtilis based on 16S rDNA and BIOLOG test analysis. The strain was named 330-2 strain. The strain produced various compounds such as indole-3-acetic acid, lytic enzymes, and structures such as siderophores. It also solubilized different organic as well as inorganic compounds such as phosphates and zinc. The strain exhibited strong antagonism against various fungi, e.g., Rhizoctonia solani AG1-IA, Botrytis cinerea, Fusarium oxysporum, Alternaria alternata, Cochliobolus heterostrophus, and Nigrospora oryzae. The strain also enhanced the growth of rice and maize seedlings. Furthermore, PCR was performed, which indicated that 10–30% of the genes are responsible for production of antibiotics, transport of nutrients as well as metabolism. The genes for antibiotic production are srfAA, bae, fen, mln, and dfnI, for metabolism areas, ltA, pabA, and ggt, and, for translocation of nutrients, fhu, glpT, and gltT. In summary, the strain has a great potential for commercialization as an excellent biological control agent against banded leaf and sheath blight (BLSB), caused by R. solani [53].
As mentioned above, PGPRs are alternatives to chemical agents and can be used as bio-fertilizers and bio-pesticides. They have the potential to replace chemical fertilizers and pesticides as they are eco-friendly as well as cost-effective. Bacillus sp. strain RMB7 was found to have a broad-spectrum activity against several phytopathogens. Strain RMB7 was identified as a Bacillus spp. based on 16S rRNA gene sequencing. When the strain was grown in a medium containing tryptophan, it produced 8 mg·L−1 of indole-3-acetic acid (IAA). The strain also suppressed nine plant pathogens, including R. solani, under in vitro conditions. Moreover, through T-RFLP analysis, it was found that the strain has a significant impact on the microbial community of the rhizosphere. The researchers also reported the presence of genes that were involved in the production of two bio-active compounds such as lipopeptide surfactins as well as iturin A. It can be concluded from the study that Bacillus sp. strain RMB7 have multifaceted beneficial functions including abilities as PGPRs as well as bio-control agents [54]. Islam et al. [55] isolated 200 bacterial strains from various soil samples of different areas of South Korea. Among the isolated strains, 19 strains were found to antagonize R. solani. Among them, strain C9 was found to be the best bio-control agent against R. solani. Strain C9 was identified as Bacillus subtilis using 16S rDNA sequencing. This strain reduces the incidence of stem segment colonization of R. solani in Zoysia grass and well, as it extended the growth of the grass. Moreover, antibiotics were also produced by using 1% mannitol and Soytoe, which were found to be the best carbon and nitrogen sources. An antibiotic that was found to be a stereoisomer of acetyl butanediol was also purified from the aforementioned strain. The application of this antibiotic to R. solani resulted in the suppression of its mycelia. The results of further studies revealed that Bacillus subtilis species constitute a reservoir of antifungal compounds that can be used as biocontrol agents as well as plant-growth promoters.
Mexico is an important producer of strawberries; however, the production of strawberries is reduced due to phytopathogenic fungi such as R. solani and Fusarium verticillioides. Both these pathogens cause a strawberry disease called secadera [56]. Basurto-Cadena et al. [56] screened healthy as well as unhealthy strawberry plants to identify native bacterial strains that can be used as bio-control agents against secadera. They isolated 600 bacterial strains. Among them, eight bacterial strains were found to be R. solani and F. verticillioides antagonists. These bacteria were identified as Bacillus subtilis strains. Among the Bacillus subtilis strains, strain B. subtilis 21 exhibited strong antagonistic activities. Under greenhouse conditions, plants treated with B. subtilis 21 strain produce more leaves per plant and also the length of leaves was found to be higher than that of the leaves of the untreated control. Moreover, the presence of proteases, chitinases, zwittermicin, and bacteriocin-like antibiotics were also detected. These substances could be implicated in managing diseases caused by R. solani. Soybean seeds were primed by Bacillus spp. strain SJ-5 to induce systemic resistance against R. solani and F. oxysporum in soybean plants. Strain SJ-5 reduced the growth of R. solani and F. oxysporum by 45% and 63%, respectively. Strain SJ-5 secreted cell-wall-degrading enzymes, i.e. chitinase, protease, and β-1,3-glucanase. Moreover, its cell-free supernatant also exhibited potent antifungal activity. Higher concentrations of defense-related enzymes (lipoxygenase, phenylalanine ammonia-lyase, peroxidase, polyphenol oxidase, and β-1,3-glucanase) were detected in various parts of soybean plants by the second week after challenge inoculation. GC–MS analysis revealed the highest concentration (91.2% and 99.84%) of jasmonic acid (JA) in soybean roots then in the roots of controls (see Fig. 2). From this result, it can be concluded that Bacillus sp. strain SJ-5 promoted the growth of the plant as well as provided protection against the elicitation by R. solani of defense-related enzymes [57].
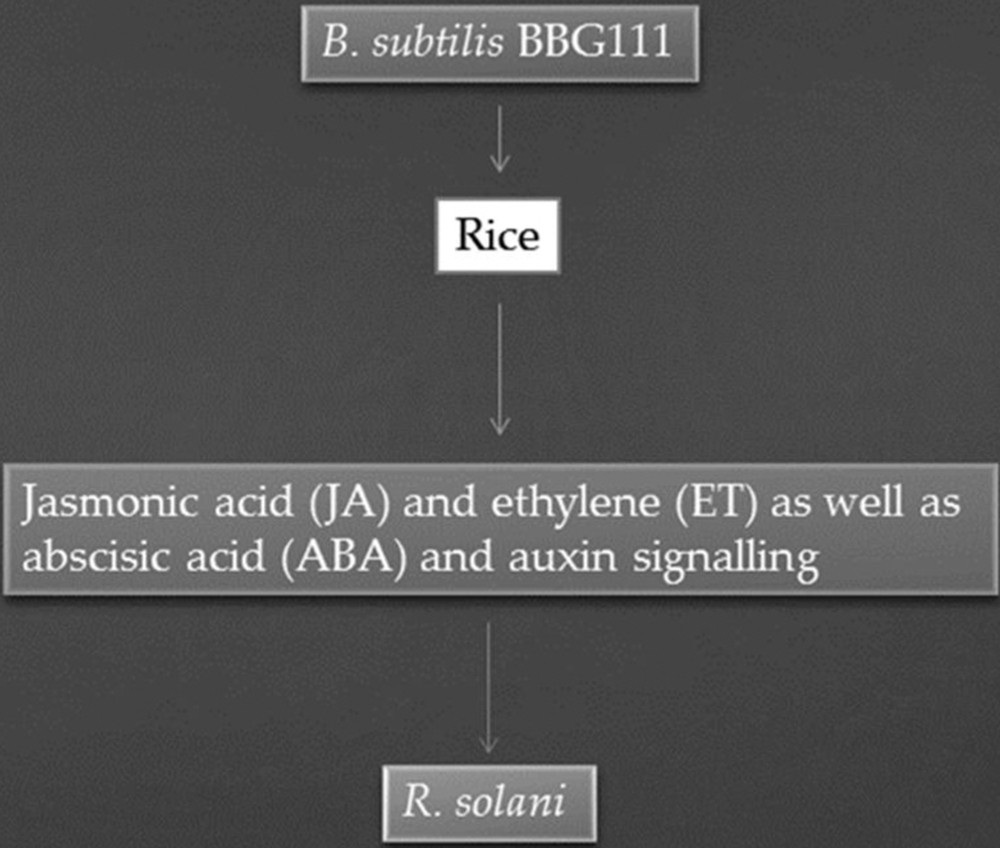
Bacillus subtilis BBG111 induce systemic resistance against R. solani.
A novel antifungal protein was recovered from a culture of Bacillus subtilis strain B29 through ion-exchange chromatography. The novel antifungal 42.3-kDa protein was named B29I. This novel protein B29I exhibited inhibitory activity against mycelial growth in Fusarium oxysporum, Rhizoctonia solani, Fusarium moniliforme, and Sclerotinia sclerotiorum [32]. Though the development of PGPR is eco-friendly and cost-effective, the selection of a single PGPR against the multiple diseases in the field is a challenge for the scientist [58]. Beneficial functions of PGPRs are shown in Table 5.
Beneficial functions of plant growth promoting bacteria (PGPB).
S. No. | Functions | References |
1 | Broad-spectrum Bio-control activities |
[58–60] |
2 | Nitrogen fixation | [61] |
3 | Solubilization of minerals | [62] |
4 | Secretion of plant Hormones |
[63] |
5 | Production of siderophores | [64] |
6 | Biofilm formations | [65] |
Li et al. [66] studied the interaction of Bacillus amyloliquefaciens SQR9 strain to different soil-borne fungal pathogens through the alteration of the production of antifungal compounds. The fungi-induced extracts of Bacillus amyloliquefaciens strain SQR9 showed strong antagonistic activities against a broad range of soilborne pathogens including Verticillium dahliaekleb, Fusarium oxysporum, Fusarium solani, and Phytophthora parasitica. The spectrum of antifungal compound production by strain SQR9 changed when faced with different fungal pathogens. The fungi-induced SQR9 extracts exhibited strong antagonistic activities compared with the strains that are not fungi-induced. When strain SQR9 faced F. oxysporum, it produced lipopolysaccharide bacillomycin D. Similarly the antibiotic fengycin was produced by strain SQR9 when it confronted Verticillium dahliaekleb, Fusarium oxysporum, Fusarium solani, and Phytophthora parasitica. Surfactin production is associated with the antagonistic activity against Sclerotinia sclerotiorum, Rhizoctonia solani, and Fusarium solani. However, bacillibactin was produced when SQR9 faced all six fungal pathogens. These results revealed a new understanding of Bacillus spp. versus phytopathogen interaction.
3 Biocontrol of diseases
3.1 Biocontrol of black scurf
Among fungal infections, black scurf caused by R. solani affects the yield and the quality of potato tubers worldwide. Bacillus spp. members are antagonists having strong biocontrol activity found in the rhizosphere of the most crops. These species also promote the growth of plants. Potato is an important crop worldwide that originated in the Andean highlands of Peru. In the Andean highlands of Peru, potato face many nutritional and phytopathogenic constraints that have a significant impact on the quality as well as on the quantity of potatoes. Among the phytopathogenic constraints, black scurf caused by Rhizoctonia solani is prevalent in the potato growing areas of Peru. In this context, there is a need for isolating natural microorganisms from its rhizosphere, which can be used to manage black scurf disease. About sixty-three Bacillus strains were isolated from the rhizosphere of potato varieties that are commonly grown in the Andean highlands of Peru. About 91% of the strains showed antagonistic activity against R. solani. Phylogenetic studies confirmed that the majority of these Bacillus strains belonged to B. amyloliquefaciences. It can be concluded that the rhizosphere of potato is a rich source of Bacillus spp. and that these species can be used as antagonists against black scurf disease [67]. Khedher et al. [68] tested the efficacy of the Bacillus subtilis V26 strain against potato black scurf caused by R. solani. The strain was a local strain isolated from a Tunisian soil. Strain V26 significantly reduced the growth of R. solani compared to untreated control under in vitro studies. Moreover, microscopy studies revealed that strain V26 had the potential to deform the morphology of the mycelia of R. solani, causing effects such as enlargement of vacuoles, perforation of the protoplast, and breakage of mycelia. Strain V26 was inoculated into potato slices about 24 h prior to the inoculation of R. solani, and as a result, effective control was achieved. The antagonistic bacterium V26 decreased the disease by 63% and 81%, respectively. The strain also promoted plant growth under greenhouse experiment conditions.
3.2 Biocontrol of damping off and root rots
The application of Bacillus-based biocontrol agents is common to protect vegetables from diseases. These bio-control agents have shown great bio-control activity against R. solani, which causes damping off and root rot of vegetables, specifically tomato. Damping off and root rot caused by R. solani has been found infecting vegetables all over the world. These diseases cause severe losses in both nurseries as well as in the translated field. In the case of tomato, light-brown-colored lesions are found on the main root just below the ground level, and finally, the roots become rotten.
Janahiraman et al. [69] applied three strains of bacteria such as Delftia lacustris PPO-1, Bacillussubtilis PPT-1, and Bacillus cereus PPB-1 to manage root rot caused by R. solani and wilt disease of tomato plants. The three strains were identified based on 16SrRNA gene sequence analysis. These bacterial species exhibited direct antagonist effect by suppressing the mycelia growth of R. solani (2.18, 2.08, and 1.98 cm) under in-vitro conditions. The strains also released volatile inhibitory compounds. These bacterial species also reduced the disease severity and incidence of tomato in plant growth chamber. Moreover, the pathogenesis-related-proteins, i.e. β-1,3-glucanase and chitinase and defense enzymes (phenylalanine ammonia lyase, peroxidase, polyphenol oxidase, and catalase) were recorded five days after inoculation. Two Bacillus spp. B. subtilis (one isolate) and B. lentimorbus (two different isolates) were isolated from the rhizoplane and rhizosphere of the tomato plants. Both were found to be effective in managing root rot of tomato caused by R. solani in greenhouse as well as in field conditions. Therefore, these strains can be commercialized to manage root-rot disease of tomato crops [70]. Various antibiotics, such as iturin A and surfactin, were obtained from the Bacillus spp., specifically from the B. subtilis [71]. These antibiotics were purified and used to control R. solani. In the Petri plate assay, the purified antibiotics suppressed the growth of R. solani. The antibiotic iturin A has strong antibiotic activity as compared to the antibiotic surfactin [7]. Moreover, the antibiotic plipastatin was also obtained from the Bacillus spp.; however, the minimum inhibitory concentration of the antibiotic plipastatin was higher than that of purified iturin A. The iturin A antibiotic A is a cyclolipopeptide consisting of seven α-amino acids (L-Asn-D-Tyr-D-Asn-L-Gln-L-Pro-D-Asn-L-Ser-) and one residue of a β-amino acid [72]. Iturin A is one of the best active agents that can be used to control R. solani. The production of iturin A reached 4.4 g per liter when bacterial strain Bacillus subtilis RB14-CS was grown in SM medium containing maltose as well as soybean meal. The cultures of RB14-CS cultures grown in SM medium were applied to the pot to manage damping-off of tomato. The suppression of R. solani by SM grown cultures was higher than that of cultures grown in original or modified number 3S media. Therefore, spores must be inoculated to the soil, and when these spores germinate, they form vegetative cells. These vegetative cells are then produce iturin A which can be used to manage R. solani [73].
Asaka et al. [7] recovered Bacillus subtilis RB14 strain from the rhizosphere of the tomato crops. Strain RB14 was found to release antibiotics such as iturin A and surfactin to suppress the damping-off disease of tomato caused by R. solani under pot experiment conditions. Culture broth, cell suspension, and cell-free culture broth of RB14 strain was prepared and inoculated into the soil. The antibiotics iturin A and surfactin were obtained from the soil when only a cell suspension of RB14-C was inoculated. The gene responsible for the antibiotics called lpa-14 was cloned from RB14 strain and mutated. Consequently, the mutant RD1 was constructed. When this mutant was inoculated, the level of disease suppressibility was low. However, when the mutant RD1 was restored with the lpa-14 gene, suppressibility of the diseases was also found to be recovered. These results confirmed that the antibiotics iturin A and surfactin are actually the product of gene lpa-14 and play a significant role in the reduction of the severity of damping-off diseases caused by R. solani.
Kondoh et al. [74] isolated strain RB14-C of Bacillus subtilis as a potential bio-control agent against the damping-off disease caused by R. solani from the rhizosphere of tomato. The experiments were carried out in pots using strain RB14-C as well as the commercially available fungicide flutoianil. The strain was grown in a medium containing flutoianil as well a medium that was free from flutoianil. It was found that the fungicide had no effect on the proliferation of strain RB14-C. However, the amount of surfactin antibiotic decreased in the flutolanil-containing medium, but no effect on the amount of surfactin was observed in the flutolanil-free medium. Surprisingly, the iturin A amount was not affected by both media. As the amount of surfactin is decreasing in the soil, the combined use of strain RB14 and of the fungicide flutolanil will reduce the damping of diseases as well as environmental problems.
In another study, two bacterial isolates, i.e. Bacillus subtilis HS93 and B. licheniformis LS674, and one strain of Trichoderma harzianum were tested alone and also in combination with chitin for the control of root-rot diseases caused by Phytophthora capsici and Rhizoctonia solani in pepper plants under greenhouse conditions. The bacterial isolates were recovered from the roots of pepper plants during washing. Under in vitro conditions, it was found that all the three biocontrol agents were antagonistic to R. solani and P. capsici. During antagonism, the highest level of chitinase enzymes was recorded. Treatment of roots as well as seeds of pepper plants with bacterial suspensions of HS93 along with 0.5% chitin was found to be more effective against root rot than the addition of bacterial suspensions without chitin. The other strains, such as LS674 and T. harzianum, reduced Rhizoctonia solani, but not Phytophthora root rot. From this study, it can be concluded that the presence of chitin resulted in the improvement of the antagonistic activity of HS93, LS674, and T. harzianum [75].
In another study, two bacterial strains, Bacillus subtilis RB14-C and Burkholderia cepacia were envisaged. Strain BY was applied in combination to manage damping off in tomato caused by R. solani. The mixture of bacterial strains was produced, and the mixtures of both strains were applied to the soil on filter disks. In pot experiments, the best bio-control activity was obtained only in combination with both strains. The best control was found when strain BY was added two days after the application of RB14-C [76]. Kim et al. [77] reported the presence of a unique strain L324-92 of Bacillus spp. around the roots of wheat. The strain was collected from the area of Lind (WA, USA), where wheat has been cultivated for more than 60 years. The strain was found to be inhibitory against anastomosis groups (AGs) of R. solani. The strain also increased yields of wheat as well as barley crop. The yield was significantly higher in response to Bacillus strain than in response to fungicides. Ryder et al. [78] selected Bacillus spp. family members such as Bacillus subtilis and B. cereus to promote wheat plant growth and to manage root-rot disease caused by R. solani Ag-8. Bacillus subtilis B931 was found to be more effective than B. cereus strain A47 in reducing the severity of root-rot disease caused by R. solani. R. solani also causes pre- and post-emergence damping off of most of the crops. In the case of oil seed crops such as rape and canola, damping off is found wherever the oil seed crops are grown. Strain Bacillus subtilis 205 was isolated from the roots of oil seed crops. The strain reduces pre-emergence damping with plants stands being almost as high as in non-diseased plants. Though the strain failed to properly reduce post-emergence damping off, it reduced however rotting in the hypocotyl [79]. A strain SQR-N43 of Bacillus pumilus was used to control R. solani in cucumbers. B. pumilis has the ability to colonize roots of cucumber and this colonization ability of B. pumilis was investigated with a green fluorescent protein (GFP) tag in vivo. Moreover, its biocontrol efficiency against R. solani was also investigated. Strain SQR-N43 deformed the hyphae of R. solani, enlarged cytoplasmic vacuoles, and perforated the cell membrane of R. solani. The results indicate that B. pumilus SQR-N43 induced hyphal deformation, enlargement of cytoplasmic vacuoles and cytoplasmic leakage in mycelia of R. solani Q1. When GFP-tagged B. pumilus SQR-N43 were inoculated, biofilm formation takes place on the root. In greenhouse experiments, B. pumilus SQR-N43 reduced the disease severity and the incidence of R. solani. Cook et al. [80] used Bacillus strain L324-92, alone and also in combination with fungicides such as difenoconazole plus metalaxyl, difenoconazole + mefoxam, tebuconazole + thiram, and tebuconazole + metalaxyl for control of the wheat root rot assumed to be caused by Rhizoctonia solani AG8. The yield was found to be higher with the difenoconazole + mefoxam + strain L324-92 treatment than in the control when either fungicide or Bacillus strain was used on winter wheat. In one location, the researchers got surprising results, since they observed that the treatment tebuconazole + thiram + strain L324-92 also reduces the disease severity of R. solani significantly [10].
Zheng et al. [81] used Bacillus megaterium strain B153-2-2 as a potential bio-control agent of R. solani isolate 2B12 (ISG-2B) that was responsible for soybean root rot. The bacterial cells were inoculated into soil either as a seed coating or as a soil application. The antagonism, chemotaxis, motility, and sporulation during the bacterial colonization of soybean seeds and roots as well the correlation between the suppression of root rot and root colonization were also studied [81]. Two soil types were selected for study, i.e. coarse and fine soil. It was found that the chemotactic response of bacterial cells to the exudates released from the soybean seeds and roots as well the antagonistic property to R. solani were significantly correlated with the seed and root colorization in some of the treatments. Those mutants that were defective in sporulation properly were found to have low population after the application. Therefore, minimum root colonization was recorded. The roots of soybean seedlings grown in coarse soils were found to have greater colonization of B153-2-2 and lower severity of root-rot disease than those in fine soils.
B. amyloliquefaciens strain FZB42 was used in pot and field experiments to control bottom root rot of lettuce caused by the R. solani AG1-IB. Moreover, the impact of strain FZB42 on the microbial community found in the rhizosphere of lettuce was also studied. These experiments revealed that strain FZB42 can effectively reduce the disease severity of bottom rot. Some of the strains of B. amylolquefaciens were mutated by continuously growing on rifampicin. The mutant FZB42-Rif could not reduce the disease and also demonstrated comparable effects on the health of lettuce as compared to wild-type FZB42 strains. Moreover, the density of FZB42 was recorded as 7.45 to 6.61 Log 10 CFU g−1 root dry mass throughout the growing season of lettuce in the field. Further, through terminal restriction fragment length polymorphism (T-RFLP), it was found that strain FZB42 did not have any major impact on the rhizosphere of the microbial community. This result indicated that bacterial strain FZB42 can establish itself in the rhizosphere of lettuce plants without affecting other microbial communities [82].
A strain of B. subtilis designated as RB14-C was investigated for its role as an antagonist against the damping-off disease of tomato caused by R. solani. The strain was reported to produce the antibiotic iturin A. Tomato seeds were coated with strain RB14-C and also directly inoculated in the soil. Seed coating and seed coating plus soil inoculation as a combined treatment could not protect the tomato seedlings from damping off. However, only upon inoculation into the soil, the disease was controlled. In the combined treatment, the colonization of the strain was recorded in uniform. The plants grown from seed coating were found to be deficient in the total number of strains. Moreover, the concentration of antibiotic iturin A was highest in non-planted soils at the beginning, and after three days, the concentration was reduced. Upon seed planting, the concentration of iturin A again increased around the roots of tomato plants [83]. Damping off and root rot of tomato caused by R. solani is commonly found in the tomato growing areas of the Haryana State in India. Four antagonist bacteria, namely Bacillus megaterium MB3, B. subtilis MB14, B. subtilis MB99, and B. amyloliquefaciens MB101 were tested against R. solani under greenhouse conditions. The roots of tomato were treated with the Bacillus strains, and these treated roots showed higher defense against R. solani. The Bacillus strains were able to secrete enzymes such as chitinase, β-1,3-glucanase and protease in the presence of cell walls of R. solani as a carbon source. Moreover, these enzymes were found at higher concentrations in the leaves of tomato than in roots. These defensive enzymes were directly correlated with the protection of tomato plants against R. solani. Among the strains, the MB101- and MB14-treated tomato plants offered 69.76% and 61.51% of disease reduction, respectively, compared to the infected control. These results indicated that Bacillus spp. have the potential to act as biocontrol agents to manage damping-off and root-rot disease of tomato [56]. Elkahoui et al. isolated various bacterial species from marine biofilms. These species were then screened to check their antifungal activity against R. solani in vitro. Among the bacterial species, two species, Bacillus subtilis SE14 and Bacillus cereus, exhibited clear antifungal activity against R. solani. Moreover, to manage the rotting disease of potato tuber, slices were treated with Bacillus subtilis SE14 and Bacillus cereus. Heavy rotting was recorded in potato tuber slices that were treated with Bacillus cereus, whereas no rotting was observed in the potato tuber slices treated with B. subtilis following inoculation with R. solani. A strain of B. subtilis MB73/2 was isolated from a meadow soil in Zulawy (Poland). This strain exhibited strong in vitro antagonistic activity against R. solani, which causes crown rot. To identify the antibiotics responsible for the antagonistic activity, whole genome short gun sequencing was performed. The obtained clusters revealed the presence of a novel antibiotic non-ribosomal peptide synthetase (NRPS) gene. This gene was responsible for slightly different biological activity against R. solani [84]. Lettuce is among the foods eaten raw and plays a significant role in the health of animals and humans. Various phytopathogens and beneficial microbes are associated with the rhizosphere and phyllosphere of lettuce plants. However, there is no published report about the impact of these phytopathogens and beneficial microbes on the microbial diversity found in the rhizosphere as well as in the phyllosphere. Erlacher et al. [85] carried out an experiment to demonstrate the impact of R. solani, the causal agent of bottom rot in lettuce, and of the beneficial microorganism B. amyloliquefaciencs FZB42 on the microbial community of the lettuce rhizosphere and phyllosphere. The highest microbial diversity was recorded when both the pathogen and FZB42 strain were co-inoculated. However, upon inoculation of R. solani the highest impact on the microbiome of the rhizosphere and phyllosphere and microbial diversity was recorded. Surprisingly, when FZB42 strain was inoculated, it lowered the impact of R. solani on the microbiome of lettuce plants. This experiment shows that both the pathogen and FZB42 may cause a potential shift in the lettuce-associated microbiome. Furthermore, when the pathogen attacks, a shift in the microbiome is observed; however, an inoculation of FB42 strain can compensate these shifts; hence FB42 can be used as a bio-control agent.
3.3 Biocontrol of rice sheath blight
Sheath blight of rice (Oryza sativa) is a serious disease caused by R. solani. The management of this disease is necessary as half of the population of the world relies on rice for food [86]. The rice sheath blight disease has also severely infected rice in Asian countries like China, India, Pakistan, Bangladesh, Sri Lanka, and is also a severe disease in South America, USA, and Africa [87] as R. solani is an inhabitant in the soil and, under moist conditions, the mycelia spread externally and sclerotia are formed. The fungus slowly reaches the upper leaves by forming lesions on the tillers. The disease initially is soil-borne but it finally becomes foliar because of the spread of mycelia [87]. The bacterium Bacillus subtilis has been used to manage rice sheath blight [86]. This bacterium is found common in Nature, and is harmless as well as non-toxic to humans, animals, and plants [88]. The bacterium secretes some antifungal compounds such as zwittermicin-A and kanosamine, lipopeptides [89] and a special protein called bacisubin [33]. In a study described in [86], B. subtilis strain NJ-18 was found to manage sheath blight disease in rice in lab and field experiments. The bacterial strain was applied at the rate of 5.0 × 107 cfu mL−1. In the lab experiments, the zone of inhibition around R. solani indicated the presence of active metabolite (antibiosis) that may have diffused in the PDA media. The morphological changes in the mycelium of R. solani are actually induced by that active metabolite. In field experiments, the strain reduced the disease incidence and severity and provided high levels of disease reduction; however the mode of action under field conditions required more investigation. Previously, Bacillus spp. family members have been used to manage rice sheath blight [90]. The bacterial strain Bacillus vallismortis EXTN-1 was used to manage rice blast and sheath blight caused by R. solani [91].
De Curtis et al. [92] selected two strains — T1A-2B and T4B-2A — from natural soils. Later these two strains were identified as B. cepacia and Pseudomonas spp. strains. The two isolates were found to show strong bio-control activity against soil-borne fungi such as R. solani and S. rolfsii, both in vitro and in vivo. These strains were tested on tomato plants in open-field conditions for two years. These two strains under field conditions were found to exert high antagonistic activity against the soil-borne pathogen as compared to commercially available chemical fungicides. Surprisingly, the bio-control activity of B. cepacia and Pseudomonas spp. strains was better than that of two commercially available bio-fungicides such as B. subtilis BSF4 and T. asperellum as well as four fungicides (tolclofos-methyl, azoxystrobin, fosetyl-Al and fosetyl-Al + propamocarb). Moreover, the population of the bacterial community was also recorded in the rhizosphere treated with biocontrol agents (T1A-2B and T4B-2A) and it was found that the population was significantly higher than that of the untreated soil. These results further indicate that the T1A-2B and T4B-2A antagonists are capable of surviving at a high level of population in the rhizosphere.
In another study, about 26 rice-associated Bacillus spp. (RABs) were isolated from the rhizosphere of rice plants. The majority of them were identified as B. amyloliquefacians spp. based on 16 S rDNA sequences and gyrB genes. Some of these RABs appeared to be B. methylotrophicus and B. subtilis. These both Bacillus spp. were also considered as strong antagonists against R. solani and also promote plant growth. Three of the RABs did not belong to Bacillus spp. and were found to be the closest to Lysnibacillus spp. [93]. These RABs exhibited bio-control activities against rice sheath blight diseases. Out of 26 RABs, five RABs exhibited strong antagonistic activities (RAB6, RAB9, RAB16, RAB17S and RAB18) against rice sheath blight disease caused by R. solani. The experiment was performed in rice fields, and these RABs were applied before inoculation of R. solani.
In another study, four B. subtilis strains were isolated from the rhizosphere of rice soils. These strains exhibited strong bio-control activity against sheath blight disease. Among the four strains, strain UASP17 showed strong antagonistic activity under field trials. This strain was evaluated against sheath blight for two seasons at different doses and methods of applications in Rajchur (India). The seedlings of rice were dipped in strain UASP17 for 30 min before transplanting the seedlings in the field. Moreover, the strain was also applied technically by foliar application at the rate of 2.50 L/ha after transplanting the seedlings in the field. The strain was found to reduce the severity of sheath blight (11.93% and 4.17%) disease for two seasons. About (61.00 and 64.30 Q/ha) yield also increased in the two seasons, respectively [94]. In another study, strain Bacillus amyloqliquefaciens FZB42 was applied on lettuce plants to promote growth as well as to manage root rot caused by R. solani. These strains were regarded as eco-friendly plant protective bio-control agents. Various secondary metabolites such as cyclic lipopeptides (CLP) and polyketides having antifungal and antibacterial activities were also recovered from this strain. To confirm whether these secondary metabolites have a possible impact on the microbial community of lettuce rhizosphere [95], an experiment was carried out under field conditions. Though the strain has a positive impact on the health of the host plant, however, there was a need to verify whether the strain is an eco-friendly plant protective agent or not; therefore, a check of its effect on the native microbial community metagenomic sequencing was performed. The rhizosphere of lettuce plants was treated with B. amyloliquefaciens FZB42, and the non-treated rhizosphere was profiled by metagenomic sequencing of whole-community DNA. When the taxonomic community profile was analyzed, marginal changes were found in the treated rhizosphere of lettuce plants [95].
Cottyn et al. [96] studied the bacterial species associated with the rice seeds. Further, the antagonistic activities against several phytopathogens were assessed. For this experiment, seeds of a local rice cultivar, PSBRc14, were procured from the fields of irrigated areas of southern Luzon (The Philippines). From the seeds, distinct colonies of bacterial strains were isolated. The strains were classified according to colony morphology, cellular features, and fatty acid compositions of whole cells. Among the bacterial isolates, the predominant ones were Coryneform spp., Pantoea spp. and Pseudomonas spp., Actinomycetes spp., Bacillus pumilus, B. subtilis, Burkholderia glumae, Enterobacter cloacae, Paenibacillus polymyxa, Staphylococcus spp. and Xanthomonas spp. The Bacillus spp., i.e. Bacillus pumilus and B. subtilis showed strong antifungal activity as compared to other bacterial species. Moreover, the Bacillus spp. significantly inhibited the mycelial growth of rice pathogens such as R. solani and Pyricularia grisea.
4 Conclusions
The pathogen R. solani is clearly a major production constraint of economically important crops. Bacillus spp. plays a significant role in the control of diseases caused by R. solani. Efforts are underway to promote the Bacillus spp. family as bio-control agents, which has led to substantial achievements. Nowadays, the market demand for bio-control agents and PGBR has increased because of the deleterious impact of pesticide residues on the health of organisms and the environment. Due to their supreme dominant traits, Bacillus spp. species are considered to be suitable biological candidates as well as plant growth promoters. With the increase of research regarding genomics, metabolomics, proteomics and transcriptomics analysis, it is expected that the future exploitation of Bacillus spp. against R. solani will be maximized. Currently, metagenomic sequencing is performed to check the effect of Bacillus spp. on the microbial community and on R. solani in the rhizosphere of plants. There are several reports on the genetic basis for the interaction of Bacillus spp. with R. solani and with the plants; however, a proper understanding of the mechanism involved still needs to be investigated. In conclusion, Bacillus spp. has multifaceted beneficial features that may be ideal for its integrated use in disease control.
Author contributions
A.A. and S.U.K. wrote the manuscript, T.A.S. and M.H.U.K. critically read the manuscript, and X.L., and W.Z. did some artwork in the paper and S.U. and A.A. did some work in the revised manuscript.
Disclosure of interest
The authors declare that they have no competing interest.
Acknowledgments
The author Aqleem Abbas has been supported by the Chinese Scholarship Council (CSC) for his PhD study.