1 Introduction
The date palm (Phoenix dactylifera L.) is a monocotyledonous and perennial Arecaceae that can be considered as an ancient relic of the Tertiary period. It is one of the most ancient perennial crops cultivated in the arid and semi-arid regions [1]. Date palm cultivation in Tunisia is of strategic importance in terms of economic, social, and environmental development. Although Tunisia represents only 2% of the global production, it is the leading producing country in terms of export value, since it reaches 30% of world's export and provides Europe with more than half of its Deglet Nour dates needs [2].
Date palm is a dioecious and heterozygous species whose male and female plants bear the vestigial organ of the opposite sex. It is known as a recalcitrant species to in vitro techniques because of the influence of genetic factors that impact the responsiveness of explants to tissue culture, thus hampering the establishment of simple, reliable, and reproducible micropropagation protocols [3,4]. Date palm tissue cultures grow very slowly: the initiation phase may require more than 24 months, especially when low amounts of plant growth regulators (PGRs) are used in order to control somaclonal variation [5].
Tissue culture is gaining importance in date palm breeding strategies because of its capacity to generate a large number of true-to-type plants from elite genotypes in a relatively shorter time. This approach can also be followed for the multiplication of disease-free planting material. Even though micropropagation is a tedious process requiring technical skills, it is definitely worth being implemented for such a tree crop with a long life cycle, whose breeding efficiency is hampered by the heterozygosity of sexual reproduction. In date palm, clonal propagation is preferred because the plant is dioecious and propagation by seeds does not produce 100% of female plants. The use of offshoots is limited because of their low number and their long generation time and it is therefore costly. Tissue culture is thus the most dependable and preferred choice for the mass propagation of elite types with higher genetic uniformity.
Tissue culture studies in date palm began in the early 1970s and progressed slowly due to slow in vitro response of cultures. In order to overcome the difficulties related to the regeneration of female date palm, young tissues, especially the apical bud [6] and the immature inflorescences [7–10], were used as primary explants. In addition, because of the in vitro recalcitrance of the plant, high doses of 2,4-D were used to fully achieve regeneration [11,12].
On the other hand, the staminodes, which are the vestigial stamens, are located in the sepal and petal axils and covered all the flower basal zone on two successive whorls [13]. The in vitro development of staminodes showed the high morphogenetic plasticity of floral tissues [14].
Another in vitro development type of staminodes was achieved by Masmoudi-Allouche et al. [13], who induced the development of these vestigial organs into typical stamens [13]. This finding permits, therefore, to support the hypothesis of an original hermaphroditic state of the species [13].
The present study aimed to assess the performance of staminodes as primary explants for date palm regeneration. We showed, here, that whatever their differentiation state, the female flowers maintained undifferentiated (dormant) and meristematic zones that can be reactivated under specific in vitro conditions and particularly through the use of appropriate combinations and concentrations of PGRs.
The in vitro cultivation of staminodes from both immature and mature date palm flowers highlighted their multiple capabilities in the morphogenetic process. We were able to evidence the cellular totipotency of the flower, whatever its differentiation state, and thus its ability to regenerate entire plants.
We describe, here, the various developmental processes revealed by date palm's staminodes and the experimental conditions leading to their expression. Both morphological descriptions and histological examinations were performed. The effect of the floral differentiation stage, on the one hand, and that of the composition and concentration of PGRs in the culture media, on the other hand, was studied. The ability of in vitro cultivation of stamonodes to provide new opportunities for date palm micropropagation was demonstrated.
In this report, the evolutionary importance of the persistence of flower staminodes at a vestigial state, was also discussed.
2 Material and methods
2.1 Plant material
Experiments were performed on immature and mature female inflorescences collected from adult date palms of the Deglet Nour variety, which were field-grown in the oases of southern Tunisia. Immature inflorescences bearing flower primordia at early stages of flower ontogenesis [13] were collected before their emergence. Adult inflorescences consisting of fully mature flowers at their final stage of differentiation [13] were sampled immediately after their emergence and prior to spathe opening.
2.2 In vitro culture
Inflorescences were disinfected with an aqueous solution of mercuric chloride at 100 mg/L for 1 h and then rinsed 3 times with sterile distilled water [13]. Spikelets were cut into 2-cm segments and transferred onto the culture medium. Spikelets shorter than 2 cm long were cultured without fragmentation.
The basic culture medium used in the present work was Murashige and Skoog's (MS) [15] supplemented with KH2PO4 (120 mg/L), myo-inositol (100 mg/L), glycine (2 mg/L), l-glutamine (100 mg/L), adenine (25 mg/L), nicotinic acid (0.5 mg/L), HCl-pyrodoxin (0.5 mg/L), saccharose (50 g/L), agar (8 g/L). The culture media were supplemented with various concentrations of PGRs (NAA: 1 and 5 mg/L; 2,4-D: 0.1, 1 and 10 mg/L; BAP: 1 mg/L) and the following combination of PGRs: NAA/BAP at 1/1 mg/L. The pH of the culture medium was adjusted to 5.8 before autoclaving at 120 °C for 20 min.
During the first 6 months (immature flowers) or 8–10 months (mature flowers) of cultivation, the cultures were maintained under complete darkness at 28 °C in order to avoid polyphenol oxidation. Explants were then gradually transferred to light conditions with a photoperiod of 16 h a day. Light intensity varied from 3000 to 6000 lux, depending on the multiplication stage. The room temperature was maintained at 26 ± 2 °C. The explants were subcultured every 2 months in the case of immature flowers and every 3 months for mature ones. Each treatment involved three replicates of 24 explants of about 500 flowers per essay condition.
2.3 Histological studies
Immature and mature female flowers were sampled at different morphogenesis stages for histological observation. Samples were fixed in Svaloff Navashine solution (chromic acid 0.5%, glacial acetic acid 5%, formaldehyde 15%, and ethanol 5%), then dehydrated through a graded series of ethanol, then embedded into paraffin. Serial 10-μm-thick sections were cut with a rotary microtome and stained with Regaud's ferric hematoxylin solution (Regaud hematoxylin solution 10%, and glycerol 10%) [13], and finally observed under a light microscope (Leica).
2.4 Statistical analysis
All experiments were performed in triplicate and results were assessed using Duncan's test for significant differences (P < 0.001 or P < 0.05). Data expressed in percentages were transformed by arcsin transformation and then analyzed.
3 Results
3.1 Development of date palm immature female flowers (DPIFFs) under different PGR regimes
Immature female flower designed a female flower whose perianthal parts (sepals and petals) and staminodes are already established, while the three future free carpels have delimited borders without showing any differentiation or separation between them (Fig. 1A, B).
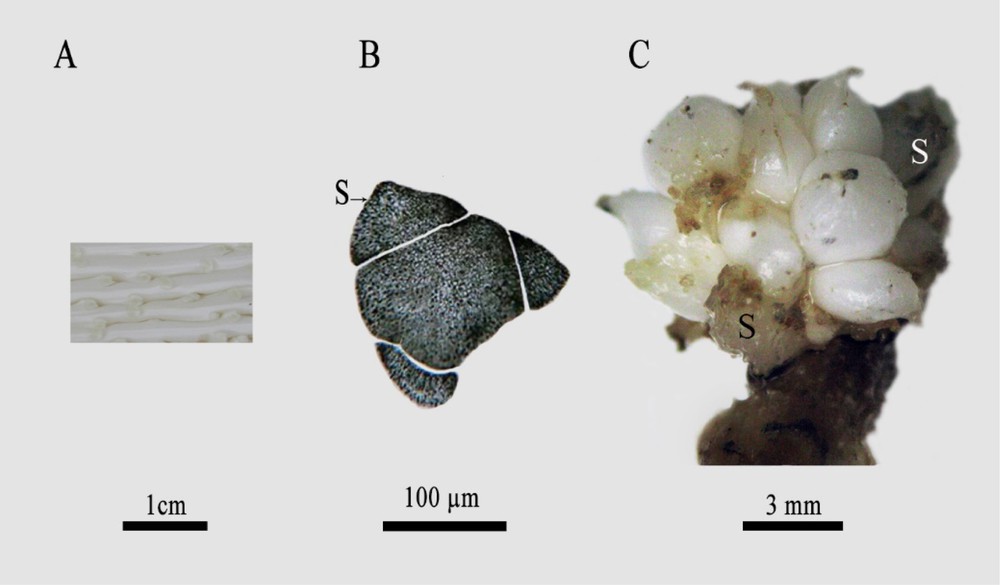
A Date palm immature female inflorescences. Scale bar: 1 cm. B: Transverse section of immature female flower of date palm, from Deglet Nour variety, revealing the presence of three sepals (S). Scale bar: 100 μm. C. Female date palm flower after 6-month cultivation, whose anterior part was delimited by sepals, differentiating numerous spherical units. Scale bar: 3 mm.
In this report, we show that the cultivation of DPIFFs for three months on MS media containing both α-naphthaleneacetic acid and 6-benzylaminopurine (NAA/BAP) (1/1 mg/L) induced the differentiation of several spherical structures (Fig. 1C) located at the anterior part of the flower surrounded by sepals. Histological analysis revealed that staminodes underwent sizeable extension and became observable (Fig. 2). The first staminode whorl (st1, st2, and st3) was arranged at petal axils (armpits) and the second whorl (st4, st5 and st6) was located at the sepal axils.
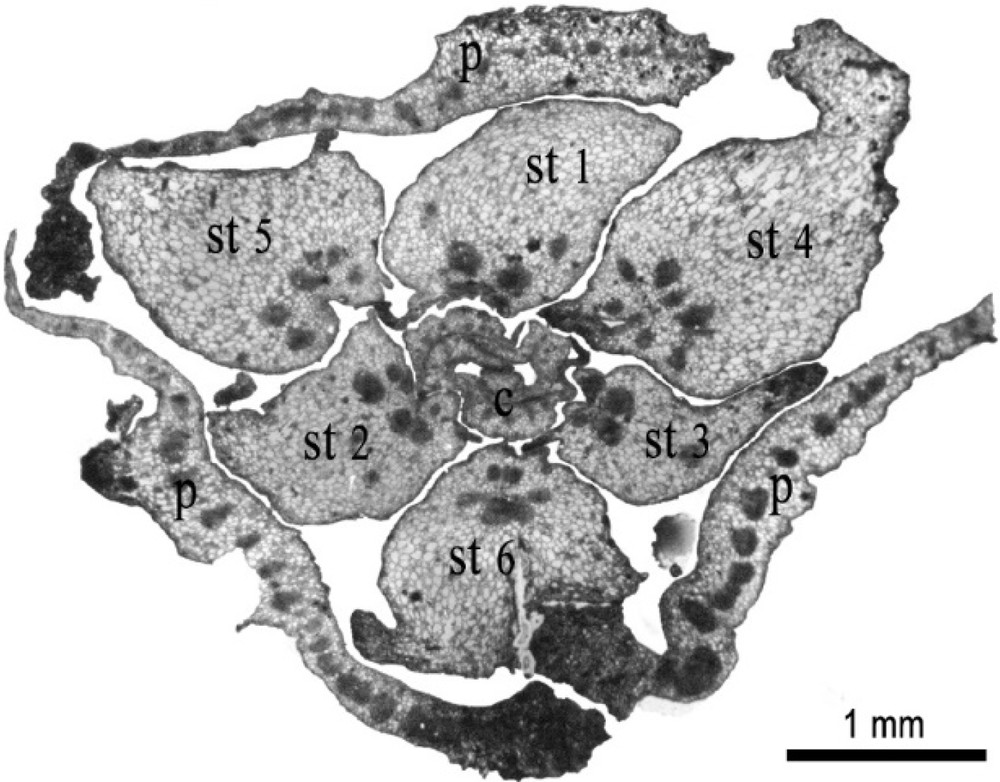
Transverse section of immature female flower of date palm after 3-month cultivation, showing 3 petals (P), the increase of the size of the 6 staminodes (st) and the early differentiation of carpels (C). Scale bar: 1 mm.
After five months of cultivation on the same media (NAA/BAP: 1/1 mg/L), some staminodes produced axial meristematic zones, which led to the neoformation of real buds (Fig. 3). The reactivation of several staminodes led to the appearance of a floral surface covered by numerous small globules. Some of these globules developed, later, to typical bud structures (Fig. 4). Subculturing such structures on the same media (NAA/BAP:1/1 mg/L) led about 8% of buds to develop into inflorescences. Such inflorescences were reduced to one single axis with typical female floral organs (Fig. 5).
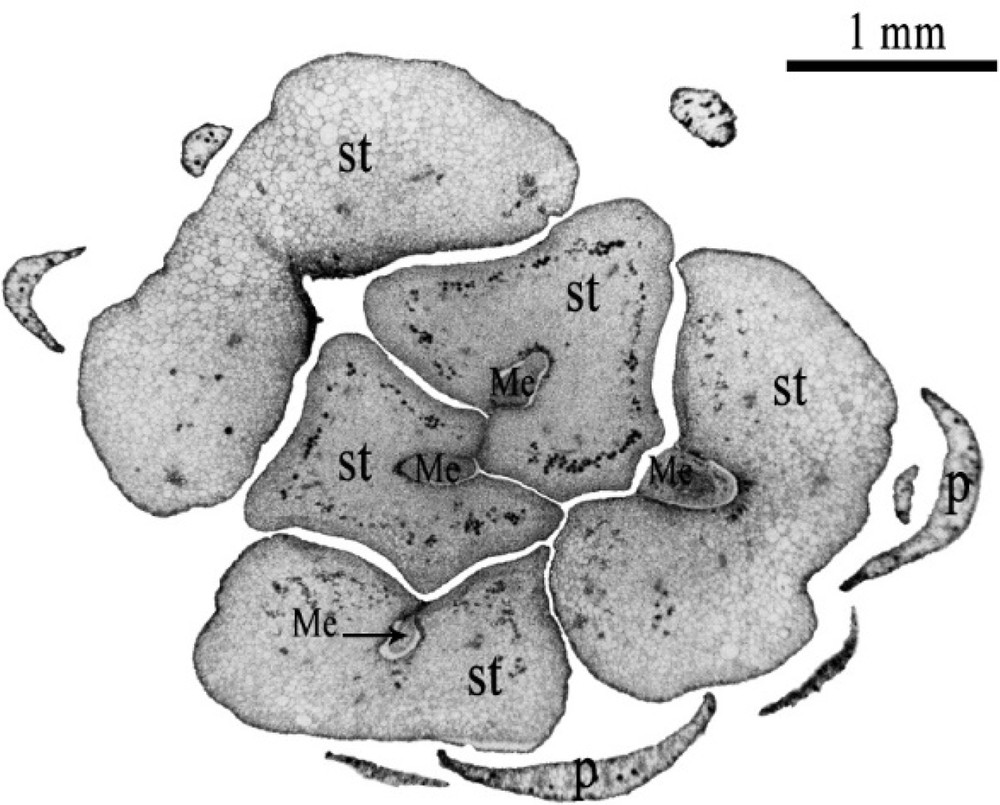
Transverse section of immature female flower of date palm after 5-month cultivation, showing a part of the petals (P) and 5 large-size staminodes (st), showing meristematic (Me) zones formed at the staminode axils. Scale bar: 1 mm.
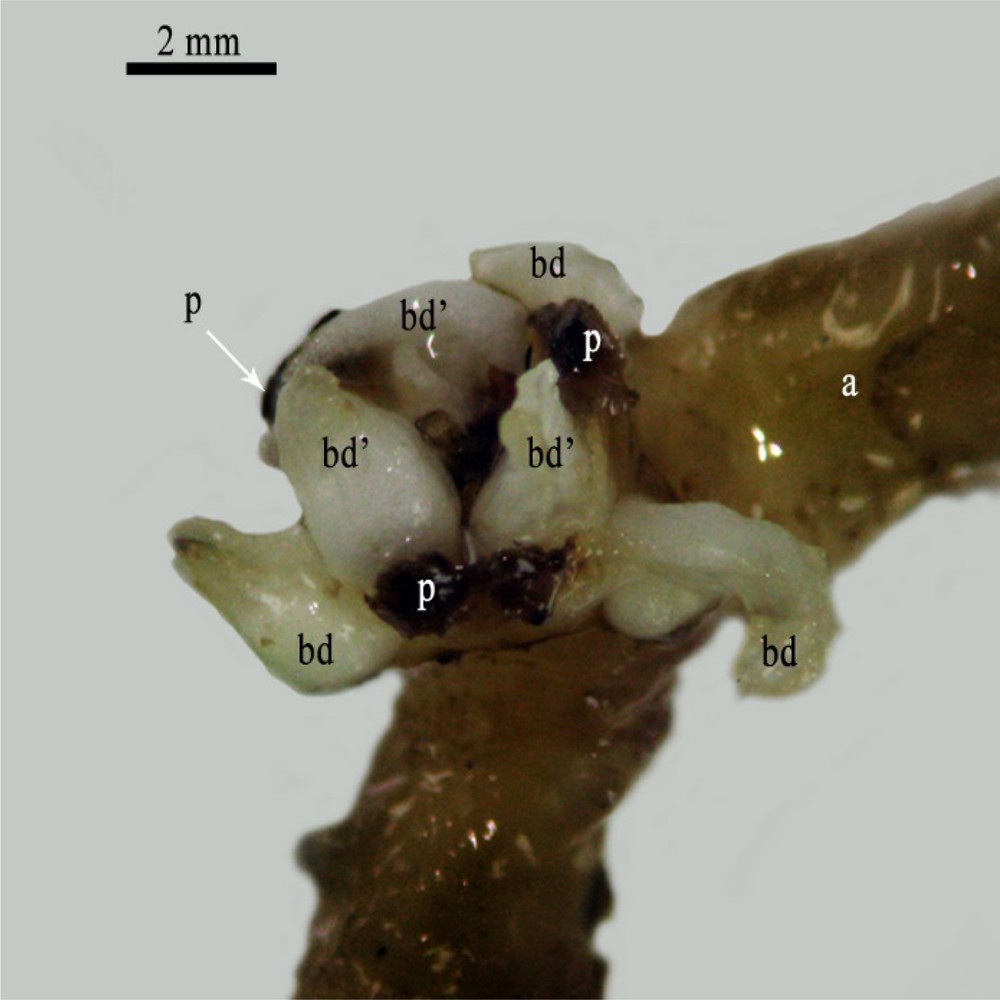
Female flower of date palm attached to its pedicel (axis) (a) after 7-month cultivation showing the presence of 3 petals (P) and the differentiation of 6 primordia buds (3 bd and 3 bd’) that were formed at the position of staminodes on two flower whorls. Scale bar: 2 mm.
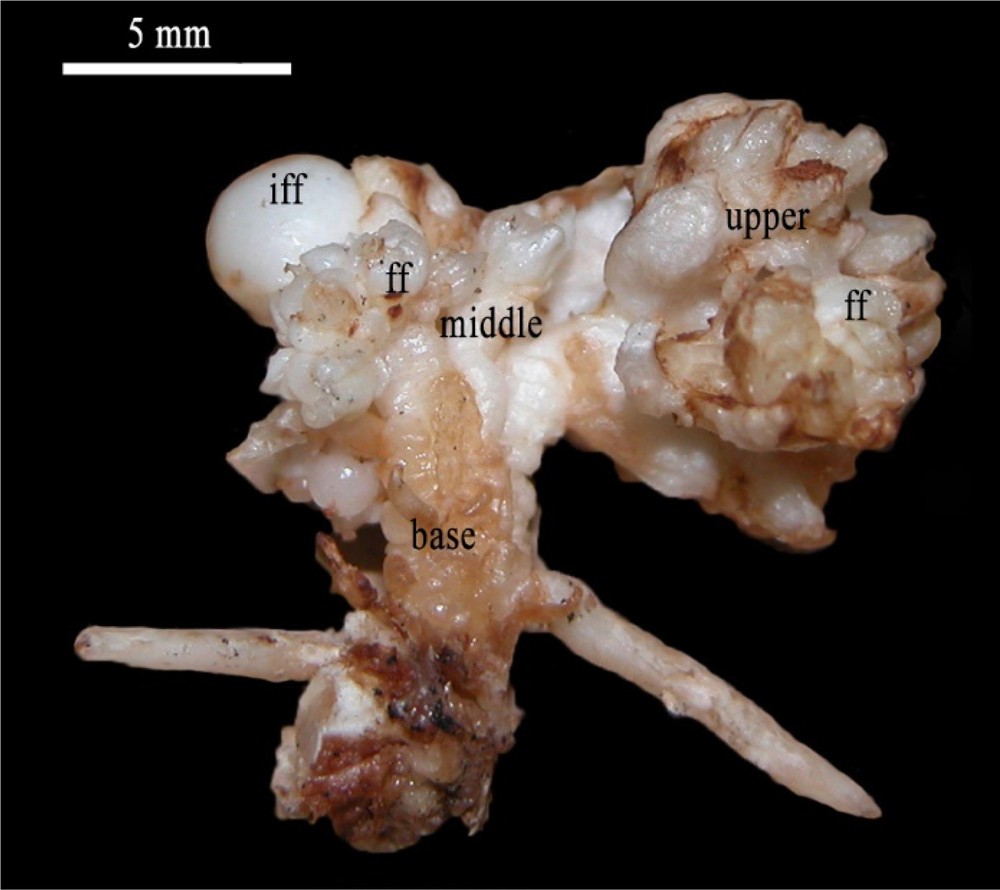
Inflorescence reduced to a single floral axis issued from the development of immature female flower (iff) bearing typical female flowers (ff) at their upper and middle parts. Scale bar: 5 mm.
On the other hand, the culture of DPIFFs on MS media supplemented with 2,4-dichlorophenoxy acetic acid (2,4-D) at a low dose (0.1 mg/L) stimulated the development of vegetative buds into foliar structures after four months of cultivation. Such development occurred without modification of pedicels or immature flower sizes. Histological analyses revealed that those buds derived from the reactivation of staminodes and led to the initiation of several small foliar structures at their axils (Fig. 6A). Subculturing these vegetative buds on the same culture medium (2.4-D: 0.1 mg/L) strongly stimulated their development and gave rise to normal and structured plants (Fig. 6B).
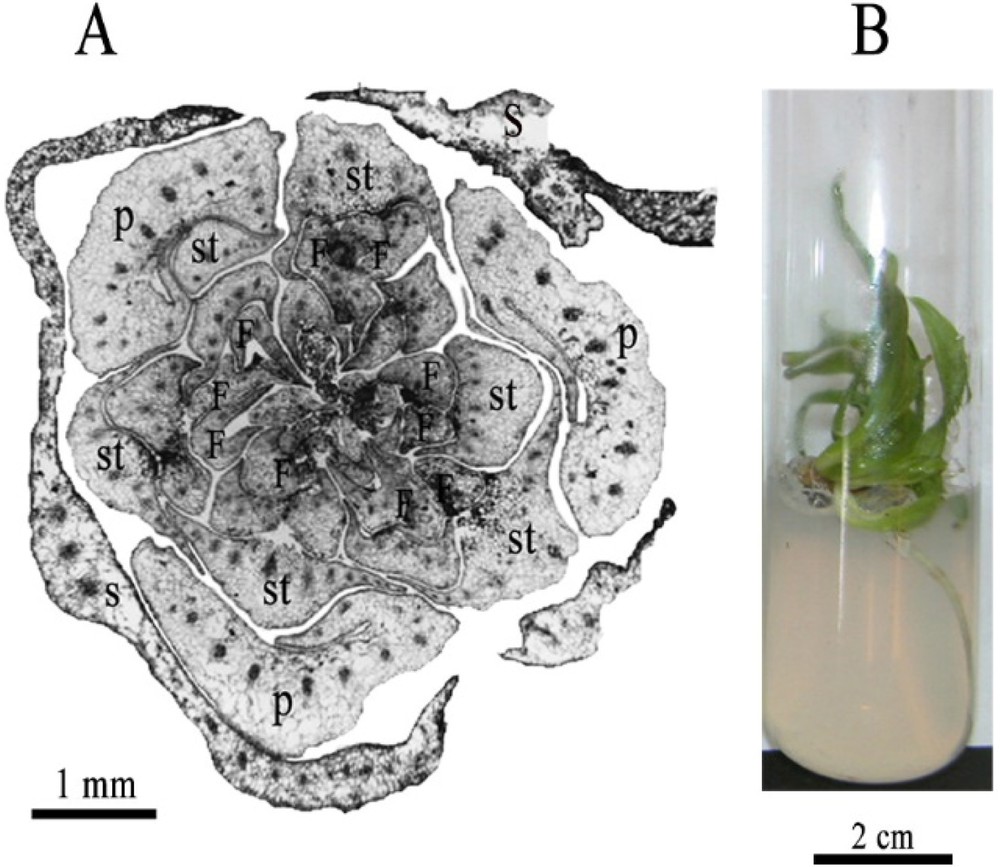
A. Transverse section of immature female flower of date palm after 3-month cultivation showing 3 sepals (S), 3 free petals (P), and 6 staminodes (st) that formed meristems at their axils initiating several small foliar structures (F); scale bar: 1 mm. B. Young rooted plants regenerated from the in vitro reactivation and development of the DPIFF staminodes; scale bar: 2 cm.
When cultured on MS media supplemented with 2,4-D at 1 and 10 mg/L, all DPIFF tissues, notably those of the central part, proliferated into callus with significant plant regeneration capacities. At these 2,4-D doses (1 and 10 mg/L), no direct initiation of vegetative or floral buds could be observed on all floral tissues, the morphogenetic development was totally produced through a callogenesis process.
The developmental processes exhibited by the DPIFFs, under the different hormonal concentrations, were presented in Table 1.
Development of the date palm's immature female flowers (DPIFFs) under different hormonal combinations. The different responses were determined at the end of the culture period and the calculations were based on the initial number of explants. Data followed by the same letter within the same column are not significantly different according to Duncan's test (P < 0.001 or P < 0.05).
Hormone (mg/L) | Response after 6 months (%)a | Final response |
NAA/BAP
(1/1) |
Induction of several spherical units 30.6 a Extension of the staminode's size 32.3 a The staminodes produced meristematic zones, which evolved into typical buds 12.2 b |
Plants |
2,4-D 0.1
1 10 |
Development of vegetative buds 15.2 a Callogenesis process 70.5 b Callogenesis process 72.3 b |
Plants Plants Plants |
a Number of callus or buds obtained/total number of flowers) × 100; about 500 flowers were used for each assay condition by several repetitions. Characters in bold: percentage of induced morphogenetic structures.
3.2 Development of the date palm mature female flowers (DPMFFs) under different PGR regimes
The histological examination of DPMFFs at their final developmental stage revealed three welded sepals, three largely recovered free petals, six small staminodes located at the sepal and petal axils, and three separate carpels harboring ovules (Fig. 7 A, B).
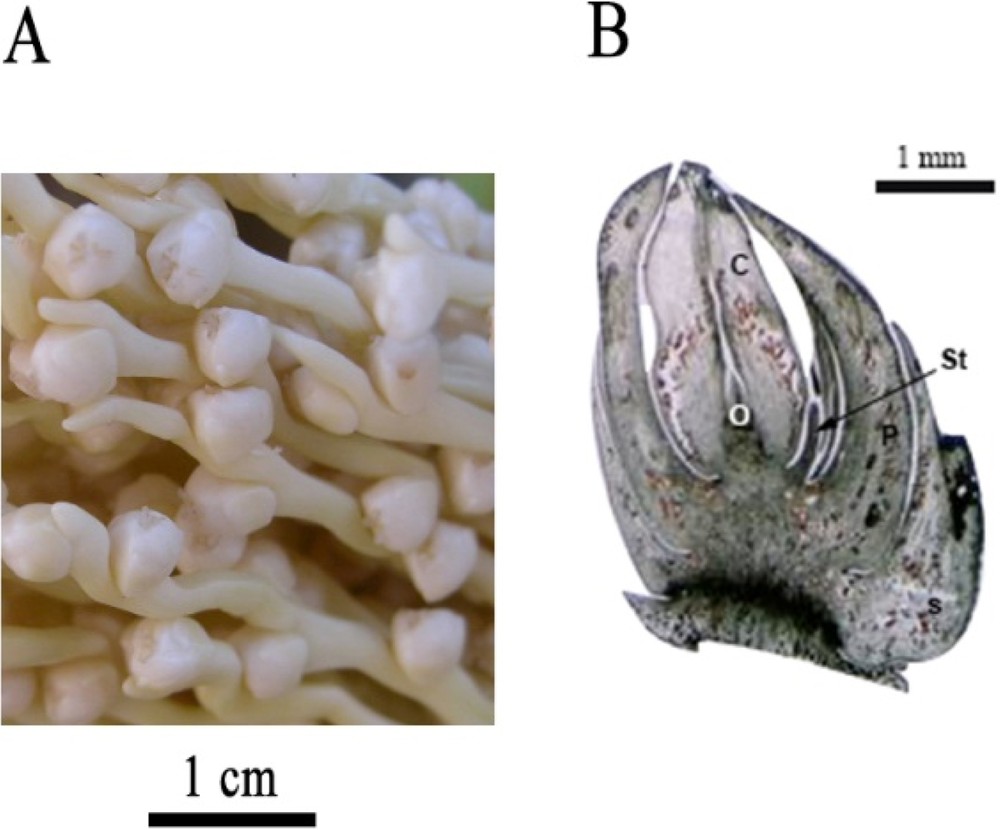
A. Date palm mature female inflorescences. Scale bar: 1 cm. B. Longitudinal section of mature female flower of date palm, from Deglet Nour variety, revealing the presence of sepals (S), petals (P), staminodes (St), carpels (C) and ovules (O). Scale bar: 1 mm.
The cultivation of DPMFFs on MS media supplemented with NAA (1 and 5 mg/L) or 2,4-D at 0.1 mg/L induced the development of roots that derived exclusively from the floral axis.
Furthermore, the cultivation of DPMFFs on MS media supplemented with 2,4-D concentrations of 1 and 10 mg/L enhanced callus proliferation (∼ 60%) at the flower basis (Fig. 8), and notably at the sepal and petal axils (Fig. 9), which corresponded to the staminode positions.
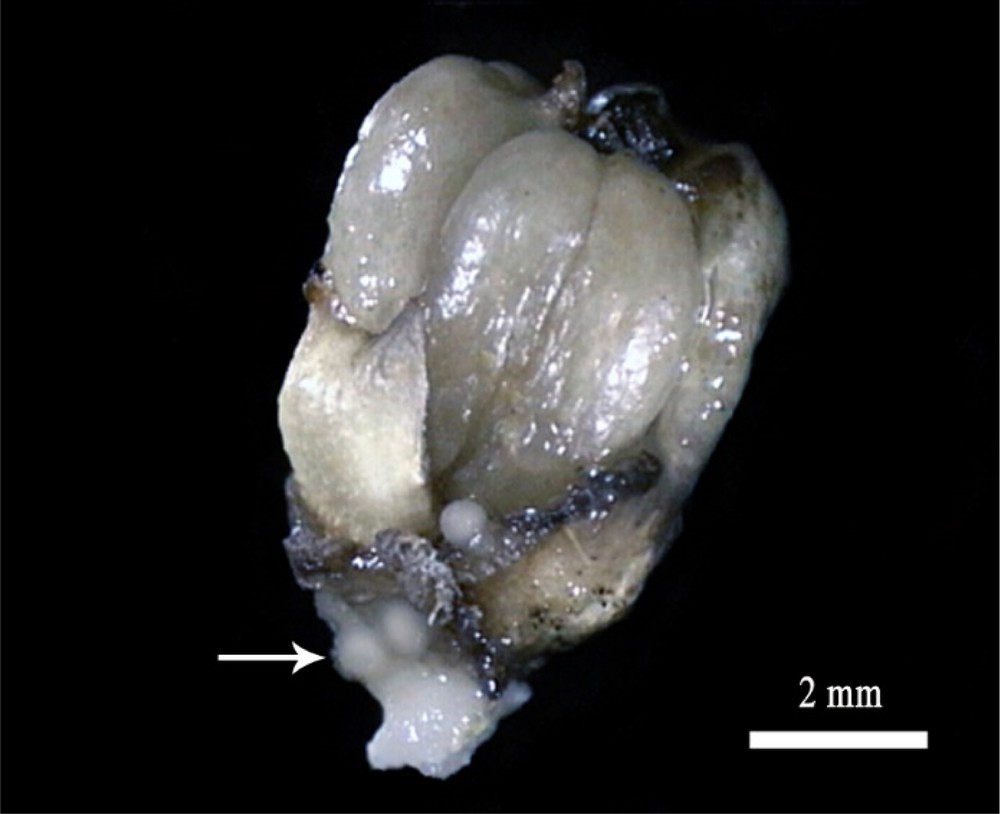
Mature female flower showing the development of callus at its basal zone. Scale bar: 2 mm.
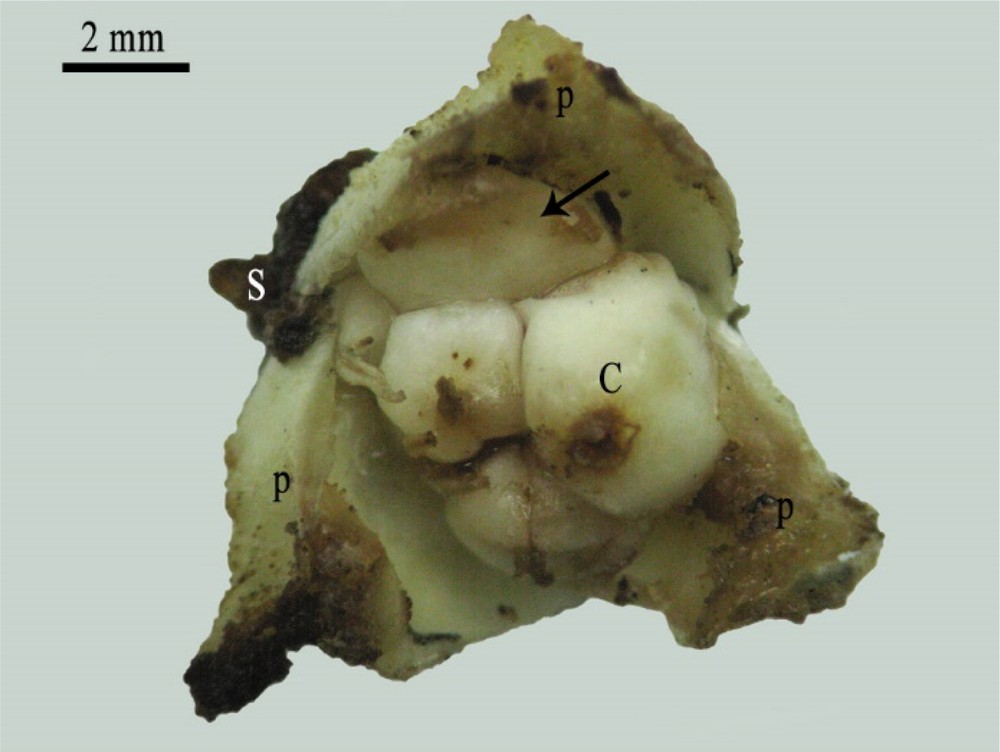
Mature female flower showing the development of callus in the petal's axil. S: sepal, P: petal, C: carpel. Scale bar: 2 mm.
Two callus types, hydrated and nodular, were regenerated. For hydrated callus, two forms were observed, namely hyper-hydrated and hydrated. While the first type (hyper-hydrated) did not show any morphogenic potentialities and showed a necrosis, the second type (hydrated) exhibited significant potential (∼ 20%) to change to an organogenic state. This shift is a main process that constitutes a major event for in vitro manifestation and expression of different capacities of DPMFFs. Concerning nodular calli, they showed a very slow growth rate and limited morphogenetic capacities. Actually, a few percent of these calli was capable to produce granular structures able to regenerate whole plants.
The data obtained showed that the transfer of DPMFFs from media containing high 2,4-D concentrations (1 and 10 mg/L) to another medium supplemented with lower 2,4-D dose (0.1 mg/L) for about a two-month culture period accelerated the transition from the callus undifferentiated tissues to vegetative bud development. Indeed, vegetative buds were produced at the sepal axils of the flower. Histological examination proved their initiation from staminodes through a callogenesis process (Fig. 10).
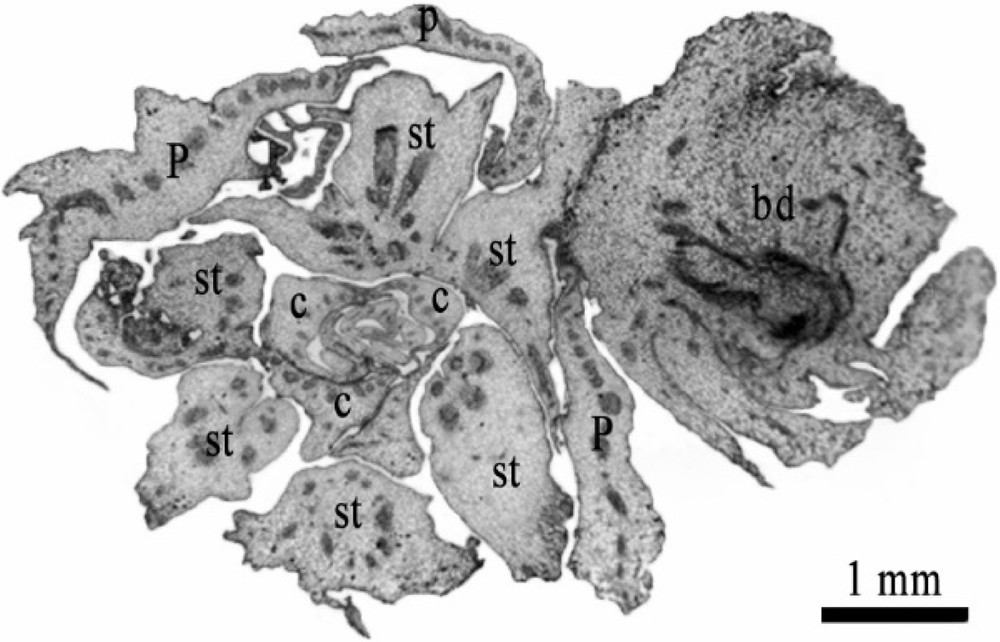
Transverse section on the basal level of mature female flower showing the development of bud issued from the tissue of staminodes. P: petal, C: carpel, st: staminode, bd: bud. Scale bar: 1 mm.
The developmental processes revealed by DPMFFs, under different hormonal combinations, are presented in Table 2.
Development of the date palm's mature female flowers (DPMFFs) under different hormonal combinations. The different responses were determined at the end of the culture period and the calculations were based on the initial number of explants. Data followed by the same letter within the same column are not significantly different according to Duncan's test (P < 0.001 or P < 0.05).
Hormone (mg/L) | Response after 10 months (%)a | Final response |
NAA 1
5 |
Development of roots | – |
NAA/BAP
(1/1) |
– | |
2,4-D 0.1
1 10 |
Development of roots and hyper-hydrated callus Callogenesis process 20 a Callogenesis process giving rise to vegetative buds 35 b |
Plants Plants |
a Number of callus obtained/total number of flowers) × 100; about 500 flowers were used for each assay condition by several repetitions Characters in bold: percentage of induced morphogenetic structures.
4 Discussion
In this report, we show that the staminode tissues in immature flowers (DPIFFs) and mature flowers (DPMFFs) acted as latent meristematic zones whose function was temporarily inhibited by morphogenetic correlations, and can be reactivated under particular in vitro culture conditions.
This result corroborates that reported in our previous study (Masmoudi-Allouche et al. [13]), in which we demonstrated that the in vitro interruption of the inhibitory correlations can be induced when using appropriate PGRs composition and physicochemical environment.
Masmoudi-Allouche et al. [13] reported that the cultivation of date palm female flowers, at different maturation stages, on MS medium supplemented with indole-3-butyric acid (IBA) (1 mg/L) and BAP (1 mg/L) reactivated staminodes and induced a new type of morphogenesis. In fact, those vestigial organs (staminodes) evolved into typical stamens without blocking carpel development and, thus, reconstituted the hermaphrodite floral state.
In this study, we observed cellular proliferation giving rise to the formation of buds, callus, miniature inflorescence structures, and to the regeneration of entire plants when DPIFFs and DPMFFs were cultivated on media supplemented with suitable PGR combinations and concentrations.
In fact, vegetative or floral buds were obtained by using NAA/BAP (1/1 mg/L) and 2,4-D (0.1 mg/L). Histological analyses revealed that those buds, derived from the reactivation of staminodes. Moreover, the callogenesis process can be produced when DPIFFs were cultivated on media supplemented with 2.4-D at 1 and 10 mg/L concentrations.
As far as the DPMFFs are concerned, a callogenesis process was gradually established, by using 2,4-D at 1 and 10 mg/L, which gave rise to nodular and hydrated calli. The latter showed a capacity for conversion into organogenic ones, leading to the generation of entire plants.
It is worth noting that most of the calli obtained (∼60%) in the case of DPMFFs were typically developed at the basal part of the flower corresponding to the staminode zones. Such results corroborate those of Kriaa et al. [16], who achieved in vitro regeneration using complete mature female flowers through staminode development. These authors [16] reported that different neoformations were observed in the female flower, the most abundant of which was callus proliferation at the basal level of the flower, corresponding to staminode location at the sepal and petal armpits.
In this context, Zayed et al. [17] studied the floral reversion of mature inflorescences of date palm and showed that the basal parts of the petals provide a stock of dormant tissues, corresponding to the staminode zone, which can be activated by ABA treatment.
Furthermore, Daher et al. [18] and Masmoudi-Allouche et al. [13] showed that staminodes, unlike other floral organs (sepals, petals, carpels, and ovules), exhibit an early growth arrest, and they remain at an immature state without undergoing apoptosis [13,18].
As shown above, and regarding to the diversity of the neoformations that were induced, the staminode tissues revealed themselves to be endowed with multiple proliferation, development, and reversion capacities when compared to other types of plant tissues.
As a matter of fact, it is important to understand the specificity of such tissues, their multiple developmental capacities, and the role that they can play in the plant's development.
Interestingly, the findings of the current study, together with those of our previous report [13], and those previously reported by Daher et al. [18] and Nadot et al. [19], corroborate the concept that dioecy in palms is not an evolutionary dead-end.
This characteristic was established in this study, which confirmed the staminode's ability to evolve when placed under favorable culture conditions, and proved the existence on its tissues of highly reactive zones that remain at a meristematic state whatever the floral differentiation stage. This high reactivity to particular environmental conditions explained the regeneration of the several types of neoformations obtained.
Accordingly, the regeneration of each structure in the female flower gives rise to the establishment of a new real axis. These multiple development processes provide opportunities for further branching in this species in accordance with Tomlinson's model [20]. According to this model, individual monocot plants produce offshoots at their base, whose lateral inflorescences arise from a pleonantic axis located at the top of the stipe [20,21].
Actually, from the date palm stipe corresponding to the order-1 axis of the plant, an emergence of spadices (the base of inflorescence) in the palm axils (order-2 axis) was observed. The latter ramified by establishing branch inflorescences (order-3 axis), which produced flowers (order 4 axis). Once cultivated in vitro, flowers would develop a new real axis from staminodes (order-5 axis).
The abovementioned investigations corroborate the findings of Emberger [22] and Nozeran [23], who indicated that the classic trimerous flowers of monocots represent a group that was contracted by the phylogeny effect.
As a consequence of this phylogeny process, the original flower structure became completely masked, but it can appear in particular conditions. Hence, specific in vitro culture conditions enabled us to reveal this state and to induce the expression of capacities that were normally repressed.
Additionally, thanks to their ability to regenerate different plant organs, as well as total plants, via several developmental pathways, the staminode vestigial tissues can be considered as an outstanding tissue type endowed with wide morphogenetic plasticity.
This large plasticity could constitute an advantage for a better adaptation to variable ecosystems through changes in the sexual reproduction system. In the same context, Nadot et al. [19] reported that the palm family showed some plasticity in its sexual expression. Moreover, environmentally dependent plasticity in the expression of male and female functions has been previously observed in Attalea Kunth [24,25]. Similarly, Adam et al. [26] stated that changes in sexual expression in the oil palm (Elaeis guineensis Jacq.) was influenced by abiotic conditions such as drought.
As a conclusion, the results of the current study provide essential findings in date palm investigation either at the practical or the fundamental level.
Accordingly, staminodes were found to be able, with appropriate PGR combinations and concentrations, to develop and regenerate several organ types. The various developmental processes exhibited by staminodes presented here were obtained thanks to the aptitude of these tissues to preserve an immature state, even in complete mature flowers. These multiple potentialities prove the totipotency of staminode tissues and, therefore, offer a novel opportunity to their exploitation as original and exceptional source for date palm regeneration through the in vitro cultivation of DPIFFs or DPMFFs.
Finally, the aforementioned data provides significant details on the flower evolution system in the date palm.
Author contributions
N.D. designed, supervised whole research, and wrote the article with contributions of all the authors. F.M.A. and W.K. performed experiments, analyzed data and provided interpretation of the results. All authors approved the final version of the manuscript.
Disclosure of interest
The authors declare that they have no competing interest.
Acknowledgements
The authors would like to express their sincere gratitude to Mr. Anouar Smaoui and Mrs. Hanen Ben Salem from the English Language Unit at the Sfax Faculty of Science for their appreciated translation and language polishing services. They also thank Dr. Riadh Drira for his valued contribution to language revision. This work was supported by the Tunisian Ministry of Higher Education, Scientific Research.